Significance of Single Stranded Structures in Molecular Biology
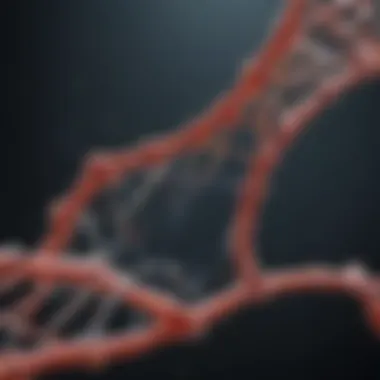
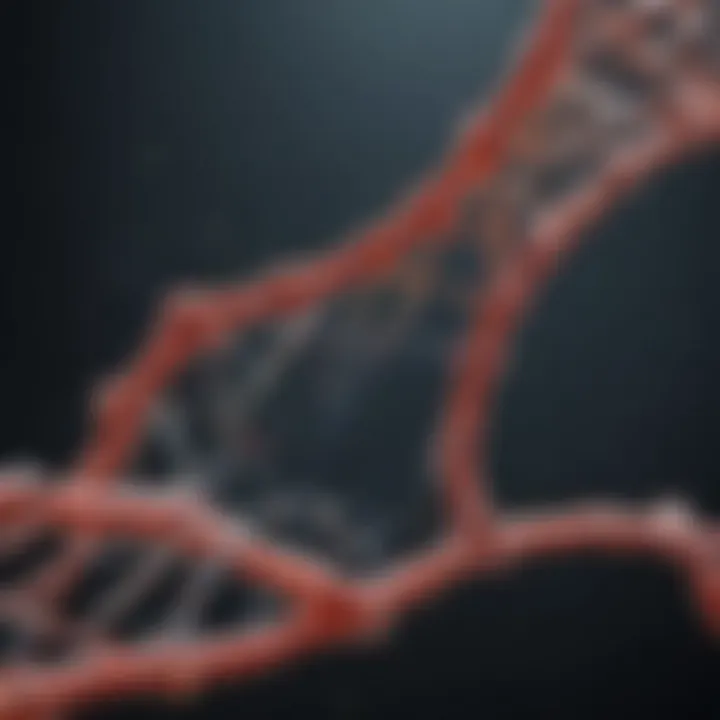
Intro
In the vast landscape of molecular biology, single stranded structures present a fascinating realm of study. These structures, often overlooked, play monumental roles in genetic processes and interact vividly with various biological systems. The importance of these single stranded molecules cannot be overstated; they are involved in everything from the foundation of life to the cutting-edge innovations in biotechnology and therapeutics.
This article aims to dissect this intriguing aspect of molecular biology, breaking down the intricacies of formation, stability, and function of single stranded structures. As we explore this paradigm, we will lay the groundwork for understanding not just the scientific principles, but also the practical implications these have on research and potential future applications.
Preamble to Single Stranded Structures
In the vast landscape of molecular biology, single stranded structures play a pivotal role, acting as both the foundation and the framework of many biological processes. By delving into the nuances of these structures, this article aims to shed light on their significance, which is often overshadowed by their more robust double stranded counterparts. Understanding single stranded molecules is not merely an academic pursuit but is instrumental in grasping the fundamentals of genetic information, mutation processes, and therapeutic advancements.
The importance of focusing on single stranded structures lies in their versatile nature. These molecules are not simply mere strands of genetic material; they are the dynamic players in the orchestra of life, participating in various interactions and processes that extend beyond mere information storage. In many ways, they are at the heart of gene expression and regulation, contributing to the intricate tapestry of life.
To grasp the depth and impact of single stranded structures, consider the following key elements:
- Defining Characteristics: Single stranded molecules, like DNA and RNA, come with unique properties that influence their interactions and functionalities.
- Historical Significance: The journey of discovering these structures parallels the evolution of molecular biology itself, marking a continuous quest to understand the building blocks of life.
- Contemporary Relevance: As research gains momentum, the study of single stranded molecules unearths many applications in therapeutic interventions, diagnostics, and genetic engineering.
Defining Single Stranded Molecules
Single stranded molecules, as the name suggests, consist of a single chain of nucleotides or amino acids. Their structure is less stable than that of double stranded counterparts, often making them more malleable and functional in specific cellular processes.
Unlike double stranded DNA, which forms a double helix, single stranded DNA (ssDNA) and single stranded RNA (ssRNA) are more vulnerable yet also more adaptable. This adaptability allows them to engage in various biological functions, such as serving as templates in synthesis and carrying genetic information.
Historical Perspectives on Molecular Structure
Reflecting on the historical context, the narrative of molecular structures is intriguing. The early 20th century brought forth groundbreaking discoveries about nucleic acids. Scientists like James Watson and Francis Crick would later build upon these findings by elucidating the structure of double stranded DNA in 1953. However, it’s essential to recognize that the research on single stranded structures has been equally instrumental in providing insights into molecular functions.
The exploration of single strands began long before the crick-watson model, where pioneering researchers grappled with the nature of genetic material. Early studies on bacterial viruses (bacteriophages) led to the realization that ssRNA or ssDNA could also deliver genetic information, setting the stage for contemporary work in molecular biology, informatics, and biotechnology.
Through this journey, single stranded structures have not only aided in understanding life at a molecular level but have also opened new pathways to innovations in genetic engineering and molecular therapies. The implications of these advancements are far-reaching, establishing single stranded molecules as essential components in the toolkit of modern science.
Types of Single Stranded Molecules
The section on Types of Single Stranded Molecules is critical to grasping the core essentials of single stranded structures in molecular biology. Each type exhibits unique characteristics that not only define their structure but also elucidate their varying functions in biological systems. Understanding these differences paves the way for appreciating their implications in genetic processes and biotechnology.
Single Stranded DNA
Structure and Characteristics
Single stranded DNA, or ssDNA, is an essential component of genetic material that serves various crucial functions. Its linear form consists of a backbone made of sugar and phosphate groups, while the nucleotide bases attach perpendicularly to this backbone. A hallmark of ssDNA is its susceptibility to environmental factors, which can affect its stability and functionality. This susceptibility brings both benefits and challenges: on one hand, it allows for quick interactions with other molecules; on the other hand, it means ssDNA is often less stable than its double-stranded counterpart. The incorporation of various modifications can enhance its stability, making it a favorable choice in applications such as genetic engineering.
Role in Genetic Information
In the realm of genetic information, single stranded DNA takes on a vital role during processes like replication and transcription. It serves as a template for synthesizing complementary strands, enabling the flow of genetic information from DNA to RNA. The key characteristic that sets ssDNA apart here is its accessibility; the single strand allows for more rapid interaction with polymerases and other enzymes compared to double-stranded forms. This accessibility is a beneficial aspect especially during genetic manipulation procedures, making it easier to synthesize genes in a laboratory setting.
Comparison with Double Stranded DNA
When comparing single stranded DNA to its double stranded counterpart, several distinctions become prominent. While double stranded DNA (dsDNA) is often viewed as the stable form critical for long-term storage of genetic information, ssDNA is more adaptable and dynamic. This contrast can be advantageous in specific applications such as phage therapy and cloning. For instance, ssDNA can readily hybridize with target nucleic acids, making it beneficial for diagnostic tools. However, due to its less stable nature, it may require controlled conditions to maintain its structure and prevent degradation, presenting certain challenges when in use.
Single Stranded RNA
Functions in Cellular Processes
Single stranded RNA (ssRNA) is ever-present in cellular processes, performing a myriad of functions including acting as a messenger, structural component, and even a catalyst. A defining feature of ssRNA is its ability to fold into complex three-dimensional shapes which contributes to its diverse functionalities. This property is particularly advantageous in coding, where ssRNA can directly influence protein synthesis. Moreover, its role in the regulation of gene expression showcases the importance of ssRNA; by mediating the flow of genetic information, ssRNA is crucial for cellular responses to environmental changes.
Types of RNA and Their Properties
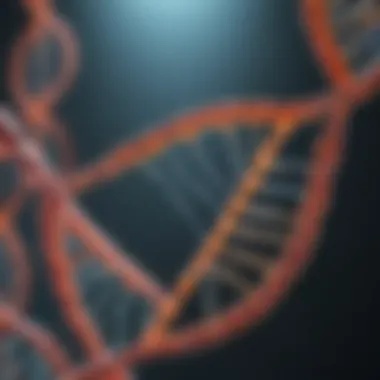
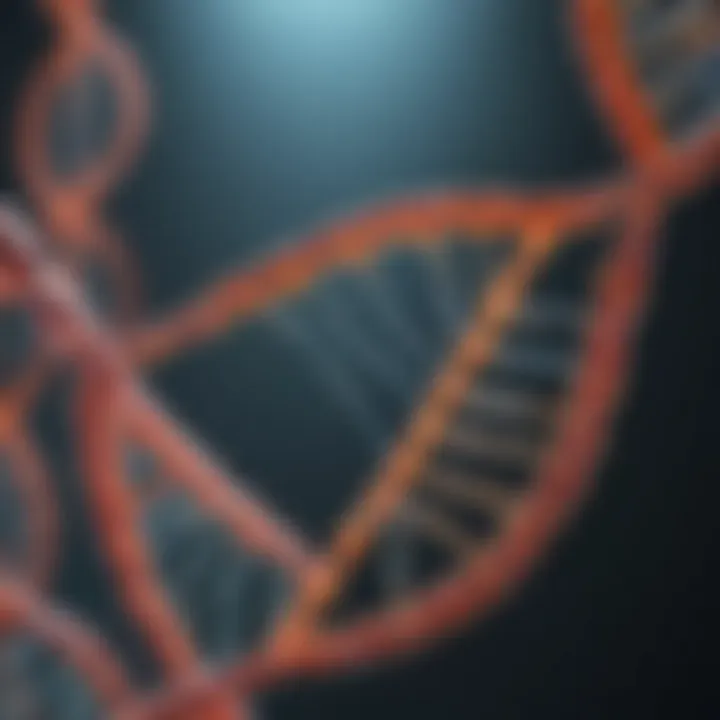
When examining the various types of single stranded RNA, one encounters a range of functional roles. For example, messenger RNA (mRNA) plays a key role in conveying genetic instructions from DNA to the ribosome, whereas transfer RNA (tRNA) helps in assembling amino acids into proteins. The distinct properties of each type stem from their structural differences and the specific functions they embody. This diversity is an asset, as different types of RNA can interact uniquely with other molecules, enabling a myriad of biological reactions.
Peptide Chains and Other Single Stranded Structures
While primarily focusing on nucleic acids, single stranded structures also extend to peptide chains. Peptide chains can be viewed as another tier of single-stranded structures, where their configurations determine functionality in biological systems. These chains consist of amino acids linked by peptide bonds, and their sequences dictate the biological roles they play. The unique folding patterns these peptide chains adopt enable them to perform various functions. However, unlike nucleic acids, peptide chains can be more resistant to the denaturing effects of heat, reflecting a different set of stability concerns.
In a nutshell, understanding the diverse types of single stranded molecules is paramount in molecular biology. By examining their structure, function, and interactions, one gains insight into their indispensable roles in genetic processes and their potential applications in biotechnology.
Formation of Single Stranded Structures
The formation of single stranded structures is a foundational aspect of molecular biology, offering insights into both genetic processes and the underlying biophysical interactions that define life. Understanding how these structures come into being aids in comprehending their functionality, significance, and stability under various conditions. This section sheds light on the mechanisms and environmental factors that are pivotal in the formation of single stranded molecules.
Synthesis Mechanisms
Transcription and Translation Processes
Transcription and translation processes are at the heart of how genetic information is expressed. During transcription, a section of DNA is copied into messenger RNA (mRNA), which serves as a template for protein synthesis during translation. This conversion from DNA to RNA exemplifies a key route whereby single stranded molecules emerge.
The critical characteristic of this process is its specificity; only the required portion of DNA is transcribed, creating a precise mRNA strand that carries the code for a particular protein. This approach is beneficial as it allows for a controlled production of proteins based on cellular demand.
A unique feature of transcription and translation is the regulation at multiple levels. Regulatory sequences can enhance or inhibit the expression of genes, providing an additional layer of control. The advantage here lies in adaptability. Cells can quickly respond to environmental changes by altering the rates of gene transcription. However, the complexity of this regulation can also pose disadvantages, as it increases the potential for errors during transcription, leading to non-functional or harmful proteins.
Enzymatic Activity in Formation
Enzymatic activity plays a crucial role in the formation of single stranded structures. Enzymes like RNA polymerase not only synthesize RNA but also assure that the process occurs with high fidelity. The ability of enzymes to catalyze reactions means that they can speed up the formation of these essential molecules, an attractive characteristic for researchers focusing on molecular biology.
A significant feature of enzymatic activity is its efficiency. Enzymes can work with remarkable speed, constructing long RNA strands from short DNA templates in mere moments. This efficiency is advantageous for cellular functions, where time is often of the essence for survival and adaptation. Nevertheless, a downside of relying on enzymes is that their activity is influenced by a myriad of environmental factors. If conditions are not optimal—such as in the presence of inhibitors or extreme pH levels—their performance may be compromised, resulting in incomplete or incorrect structures.
Environmental Factors Influencing Stability
pH and Temperature Effects
The stability of single stranded structures is significantly influenced by pH and temperature, two critical environmental factors. The specific pH can affect the ionic state of nucleotides and the hydrogen bonds that stabilize single stranded configurations. When pH levels vary, the charges of the components change, leading to potential destabilization of structural integrity.
Temperature, on the other hand, directly impacts molecular motion. High temperatures can enhance molecular interactions, but they may also lead to denaturation—the loss of structure and function. The balance between these factors is essential for maintaining the stability of single stranded molecules, making this discussion particularly relevant for researchers focusing on molecular integrity.
A unique aspect of pH and temperature effects is their duality. They can both support and challenge the formation of stable structures, providing insight into how natural and artificial systems might regulate stability. On one end, these characteristics give an advantage in designing experiments and treatments in biotech, while on the other hand, they show potential challenges that must be navigated to ensure the integrity of bio-molecules.
Ionic Strength and Solvent Effects
Ionic strength plays a critical role in the stability of single stranded structures, as it influences the interactions between charged groups within molecules. Higher ionic strength can stabilize single stranded configurations by shielding the negative charges on the phosphate backbone of nucleic acids, reducing repulsion forces between strands. This makes ionic strength a beneficial focus for researchers in molecular dynamics.
Solvent effects are equally important, as the choice of solvent can influence the solubility and stability of single stranded molecules. The presence of certain solvents can facilitate or hinder the formation of hydrogen bonds and other interactions pivotal for maintaining single stranded integrity.
A unique attribute of ionic strength and solvent effects is their interplay. Together, they can create environments that either promote stability or induce destabilization of single stranded structures, making them key considerations in both research and application. The comprehensive understanding of how these factors operate allows for more effective manipulation of single stranded structures in various scientific disciplines, from genetic research to therapeutic development.
The Stability of Single Stranded Structures
The stability of single stranded structures plays a crucial role in their functionality within molecular biology. Without a solid understanding of stability, the implications of these molecules in genetic processes and biotechnological applications would remain murky. The stability directly affects a number of aspects, including the behavior of single stranded DNA and RNA during replication and transcription, and the effectiveness of therapeutic interventions that utilize these strands.
In molecular terms, stability encapsulates the resilience of these structures against external conditions such as temperature, pH, and ionic strength. This stability is not merely a static property but rather a dynamic balance of several forces at work. Realizing how these forces interact helps in appreciating the intricate nature of biological processes, particularly in the context of their implications for genetic engineering and medicine.
Thermodynamics of Stability
Thermodynamically, the stability of a single stranded molecule is often analyzed through the lens of free energy changes. A single stranded DNA or RNA's propensity to adopt certain conformations is a reflection of how energy is distributed in the system. The free energy is influenced both by enthalpic contributions—vital for formation of intermolecular interactions—and entropic components, which come into play through molecular disorder and flexibility. A stable single stranded structure will typically reflect a minimum in Gibbs free energy, where the system sits comfortably at a certain energy state.
In simpler terms, the interplay of energy states and molecular movements dictates whether a single stranded structure can maintain integrity or succumb to conditions that might compromise its form.
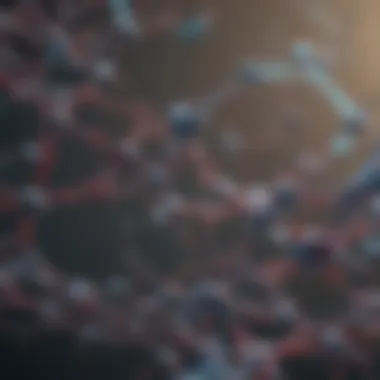
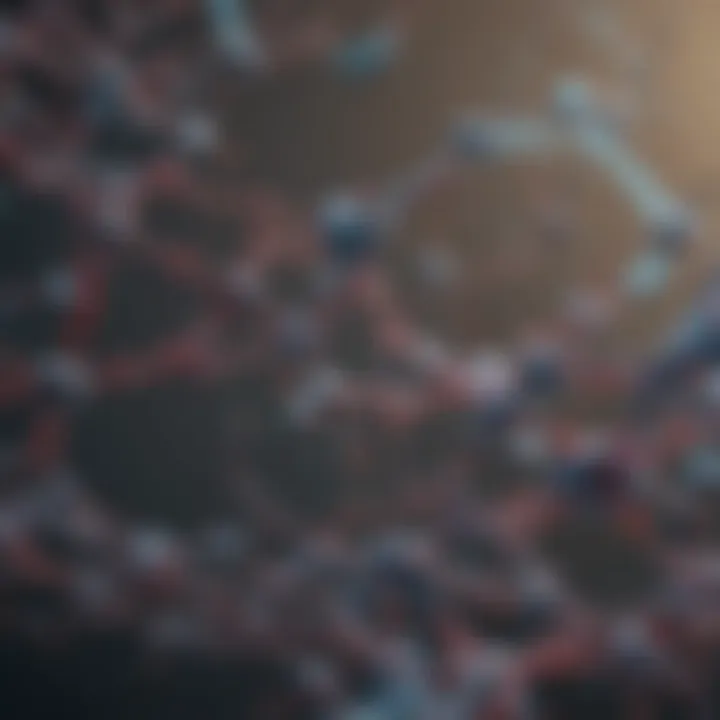
Interactions with Other Molecules
Hydrogen Bonding and Hydrophobic Interactions
Looking closely at interactions, hydrogen bonding and hydrophobic interactions emerge as fundamental contributors to the stability of single stranded molecules. Hydrogen bonds, while less robust than covalent bonds, provide a necessary network of interactions that stabilizes various molecular conformations. Furthermore, amid the watery landscapes of cellular environments, hydrophobic interactions persuade nonpolar regions of molecules to minimize contact with water, thus enhancing overall stability.
The real beauty of these interactions lies in their adaptability. Hydrogen bonds can transiently form and break, allowing for necessary flexibility. This flexibility is vital, especially under biological processes like transcription where dynamic changes are key. These interactions are a beneficial choice as they help forge a delicate balance between stability and flexibility. Accepting unique features, like the variability in number and strength of hydrogen bonds, reveals both advantages and disadvantages; more bonds mean greater stability, yet there’s always a risk of instability if the right conditions aren’t met.
Role of Cation and Anion Stabilization
The stabilizing role of cations and anions can't be overlooked when discussing molecular stability. When these ions come into proximity with single stranded structures, they can neutralize charges, which in turn minimizes repulsion between negatively charged phosphate backbones. This ion-induced stabilization can be observed significantly in solutions, where certain ions enhance the overall attractiveness of molecular structures.
The key characteristic of cation and anion stabilization lies in their ability to bridge gaps between charges. By doing so, they're pivotal in combating the natural repulsiveness of single stranded structures. The distinctive feature here is that not only do they boost stability, they also maintain the structural integrity of molecules through various environmental changes. While leveraging such stabilizing properties can be advantageous, the dependence on specific ionic conditions may pose limitations in less controlled or variable experimental environments.
Understanding the balanced interplay of these molecular interactions enhances our grasp on how single stranded structures can effectively serve critical roles in biological systems, allowing for more innovative applications in the fields of genetics and therapy.
Functional Roles of Single Stranded Molecules
Single stranded molecules play vital roles in various biological processes, especially in genetic applications and therapeutic contexts. They are not merely structural components; instead, they're at the core of how genetic material is expressed and manipulated, influencing advancements in molecular biology and biotechnology. The functional diversity of these molecules opens doors to groundbreaking applications, bridging basic molecular functions to clinical innovations.
Genetic Applications
Role in Genetic Engineering
The role single stranded molecules play in genetic engineering is nothing short of revolutionary. This aspect focuses on how these molecular forms facilitate the precise alteration of genetic material. One significant example is the use of single stranded DNA in designing constructs that interact well with host genomes. Unlike double stranded structures, single stranded DNA can hybridize with complementary sequences, allowing for more efficient incorporation into cells. This characteristic renders single stranded DNA an attractive choice for techniques like gene editing and cloning.
The unique feature of single stranded DNA's ability to specifically target regions in genetic sequences enhances its popularity in synthetic biology and genetic modification. However, while it presents many advantages, such as higher efficiencies in nucleotide incorporation, it can also face challenges, particularly regarding stability and susceptibility to degradation. Thus, understanding both its strengths and limitations is essential for effective application in research and development.
Utilization in CRISPR Technology
Utilization of single stranded molecules in CRISPR technology has underscored their significance in modern molecular biology. By employing single stranded RNA guides, researchers can target specific DNA sequences for editing. The flexibility in design ensures that various sequences can be effectively modified, thus broadening the scope of genetic engineering.
This method's appeal lies in the precise and controllable nature of the single stranded RNA, allowing scientists to direct molecular machines to exact locations within the genomic landscape. The unique aspect here is the synergy between single stranded RNA and Cas proteins that makes editing highly efficient. However, while CRISPR’s reliance on RNA guides is a powerful tool, off-target effects remain a concern, necessitating continued research to refine techniques and applications.
Therapeutic and Biotechnological Uses
Single Stranded Oligonucleotides in Therapy
In therapeutic realms, single stranded oligonucleotides offer significant promise. These short sequences of nucleotides can be designed to complement specific RNA or DNA targets, leading to the downregulation of genes associated with diseases. This therapeutic potential lies in their ability to serve as antisense oligonucleotides, effectively shutting down the expressions of problematic genes.
The major advantage of using single stranded oligonucleotides in therapy is their specificity and ability to target the gene expression at the transcriptional level. As a result, they present as timely and effective strategies in the management of various genetic disorders. Nonetheless, challenges such as delivery mechanisms and the development of resistance in target cells can add layers of complexity to their application. A nuanced understanding of these elements is crucial for therapeutic implementations.
Diagnostic Applications in Medicine
The landscape of diagnostic applications in medicine has also been transformed through the integration of single stranded structures. These molecules enable precise detection of pathogens or mutations through various assays. Their use in techniques like polymerase chain reaction (PCR) highlights their capability to amplify tiny amounts of DNA or RNA, making them invaluable for diagnostic purposes.
The key characteristic that makes single stranded molecules a staple in diagnostics is their specificity and sensitivity. Assays that deploy these molecules have a higher level of accuracy in identifying mutations or infections compared to traditional methods. However, the complexity of sample preparation and the potential for contamination are notable considerations that can impede diagnostic accuracy. Hence, adapting strategies to counteract these challenges can significantly enhance their utility in clinical settings.
Challenges in Studying Single Stranded Structures
Examining single stranded structures in molecular biology presents a captivating yet complex set of challenges. To grasp the full scope of these hurdles is crucial for researchers who aim to push boundaries in genetic engineering and therapeutic applications. Failure to navigate this terrain can hinder advancements and limit understanding, making insight into these challenges invaluable.
Technical and Methodological Limitations
Analytical Techniques Overview
Delving into analytical techniques reveals a mixed bag of powerful tools for studying single stranded molecules. Techniques such as nuclear magnetic resonance (NMR) and mass spectrometry are kind-of like the Swiss Army knife for molecular biologists; they provide a versatile approach to not only identify but also characterize the molecular structures. These methods stand out for their ability to analyze the properties of single strands under varying conditions, making them a popular choice for researchers eager to unlock the secrets of molecular interactions.
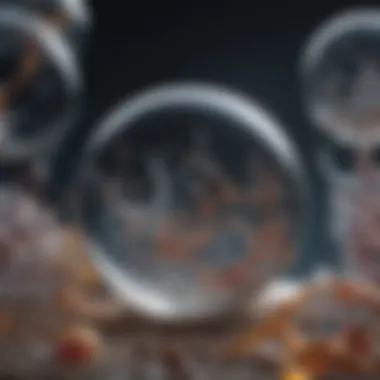
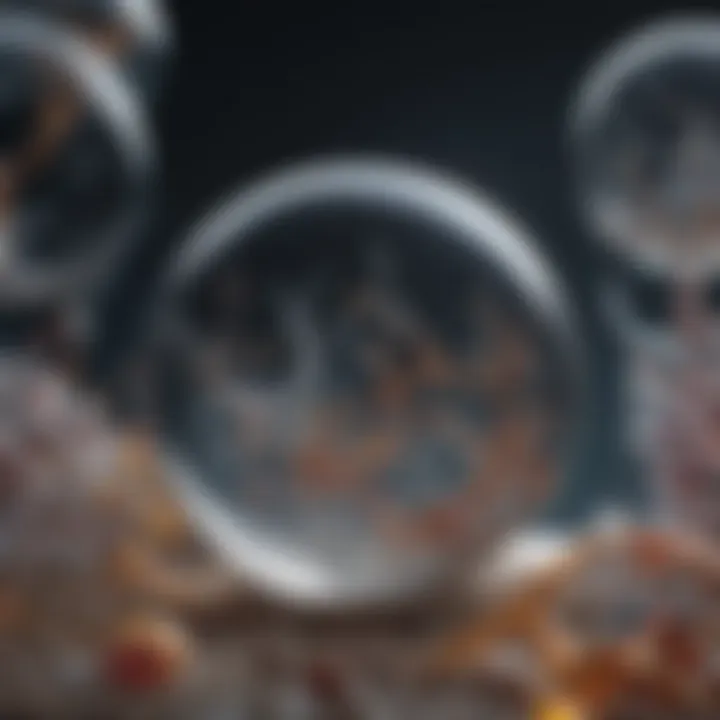
One unique aspect of these analytical techniques is their adaptability. For instance, NMR can analyze both liquid and solid samples, providing insights into the internal dynamics of these molecules. However, it doesn't come without its downsides. The complexity of sample preparation and the requirement for highly sophisticated equipment can be taxing, particularly for labs with limited resources.
Resolution and Sensitivity Issues
When it comes to resolution and sensitivity issues, these throw yet another spanner in the works. High-resolution techniques, while offering fantastic detail, often require substantial amounts of material. This is especially hard with single stranded molecules that might be present in minimal quantities in biological samples. Think of this like trying to find a needle in a haystack; without enough sensitivity, the chances of detection diminish dramatically.
The challenge is heightened when researchers contend with varying molecular conformations, which can affect how these strands are detected. Despite these concerns, a big upside to addressing resolution issues comes from innovations in imaging techniques, offering potential paths for improvement in detection capabilities. Yet, caution remains salient, as tweaking these methods might lead to bias in the results, obscuring true biological variability.
Biological Variability and Its Impact
Biological variability introduces another layer of complexity in understanding single stranded structures. Each biological system possesses its own specific set of variables, such as age, health, and environmental influences, creating a landscape of diversity that can skew experimental results. For instance, the biological differences between individual organisms can lead to discrepancies in molecular behavior, making standardization across studies an elusive goal.
"In the realm of molecular biology, variability isn't merely a nuisance; it embodies the intricate tapestry that reflects the complexity of life."
To cope with these issues, researchers are now exploring ways to quantify these variabilities rather than merely noting them as problematic. This approach might lead to more robust models that integrate variability as a factor, rather than treating it as a confounding variable. Addressing these challenges holds significant promise for more accurate models in both research and therapeutic contexts.
Future Directions in Single Stranded Research
The exploration of single stranded structures in molecular biology is akin to digging through a treasure chest brimming with knowledge. Progress in this field has highlighted unique aspects of cellular mechanisms and genetic processes that were once shrouded in mystery. This section aims to shine a light on where the future of this research is headed, as it holds great importance for various applications in biotechnology, medicine, and beyond.
Emerging Technologies and Innovations
Advancements in Sequencing Techniques
Recent advancements in sequencing techniques have revolutionized molecular biology. The ability to sequence single stranded DNA and RNA has opened doors to understanding genetic variations with unprecedented accuracy. High-throughput sequencing technologies enable researchers to analyze entire genomes at breakneck speeds, which is crucial for both clinical and research settings.
One key characteristic of these advancements is their ability to produce massive amounts of data. This feature makes them particularly appealing to researchers aiming to understand complex biological environments. One such popular and beneficial approach is the use of next-generation sequencing (NGS) methods, which significantly reduce the time and cost associated with traditional sequencing techniques.
With NGS, distinguishing between single nucleotide polymorphisms has become more straightforward, even in organisms with vast genetic diversity. However, the sheer volume of data generated can be overwhelming, necessitating sophisticated computational tools to manage and interpret results.
Computational Models in Molecular Dynamics
The role of computational models in molecular dynamics cannot be overstated. These models help simulate and visualize the behavior of single stranded structures on a molecular level. They provide insights that experimental methods alone sometimes cannot offer, especially when it comes to studying dynamic processes over time.
A standout feature of these computational models is their ability to predict structural changes based on different environmental conditions—such as temperature or pH. Such modeling tools have become indispensable in designing experiments that further elucidate the functionality of single stranded molecules. Their ability to simulate interactions at an atomic level aids researchers in anticipating behavior before it occurs in laboratory settings.
However, a common disadvantage lies in the assumption of ideal conditions that may not always reflect reality. These models rely heavily on the accuracy of the parameters inputted, which can lead to discrepancies in predicted outcomes versus real-world observations. Still, their contribution to advancing our understanding of molecular dynamics remains invaluable.
Potential Applications in Synthetic Biology
The concept of synthetic biology is rapidly changing the landscape of biotech research. The study of single stranded structures presents countless opportunities for engineering organisms with desired traits.
- Genetic Circuits: By manipulating single stranded RNA, engineers can create genetic circuits that respond to specific stimuli. This has implications for creating bacteria capable of biosensing environmental changes.
- Targeted Therapy: Single stranded oligonucleotides can be designed to target specific genetic sequences for therapeutic purposes, offering potential solutions to inherited diseases.
- Bioluminescence and BioReports: Incorporating single stranded elements into bioluminescent systems can enable real-time monitoring of biological processes in living cells.
In summary, as we venture further into the world of single stranded structures, emerging technologies and innovative applications promise to reshape our understanding and utilization of these fundamental biological components. With the mapping of genetic blueprints and the simulation of molecular interactions, the future of research in this area looks bright and full of potential.
The End
In the tapestry of molecular biology, single stranded structures emerge not just as components, but as pivotal players in the grand narrative of life. These delicate strands—whether they be single stranded DNA or RNA—carry the heavy burden of genetic coding, regulation, and expression. Their significance is multilayered, underpinning numerous biological processes and offering a glimpse into the intricate workings of living systems.
Understanding the nature and roles of single stranded molecules is critical for several reasons. Firstly, they serve as essential tools in genetic engineering and synthetic biology. This is highlighted by their applications in CRISPR technology, where single stranded guides direct precise genomic alterations, showcasing the delicate balance between stability and adaptability in molecular interactions.
Moreover, the stability of these structures impacts their functionality, reflecting an ongoing dance of thermodynamics and molecular bonding. Factors such as pH, temperature, and ionic strength present ongoing challenges that scientists continuously seek to master. Enhancements in understanding these parameters not only digitize the bonds of great scientists past but also pave the way for breakthroughs in therapeutic and diagnostic realms.
The future direction of research into single stranded structures promises an expansion of our knowledge base. Innovations such as next-generation sequencing and computational modeling will likely revolutionize the field, enabling more refined approaches to dissecting molecular behavior. As researchers push boundaries, the therapeutic implications of these single stranded molecules will increase, thus fostering more substantial connections among biotechnology, medicine, and the very fabric of life itself.
As we reflect upon the discussions within this article, we recognize that single stranded structures are not mere curiosities in molecular biology. Instead, they are fundamental to our understanding of genetics, biochemistry, and the larger framework of biological systems. This holistic perspective undoubtedly enhances our approach toward education, research, and application, reminding us to appreciate the simplicity and elegance that single stranded structures encapsulate.
In summary, mastering the complexities of single stranded molecules is not just an academic pursuit; it's a step toward unlocking the secrets of life, one strand at a time.
Keywords
- Single Stranded DNA
- Single Stranded RNA
- Genetic Engineering
- Molecular Biology
- CRISPR Technology