Understanding Thermal Conduction: Principles and Applications
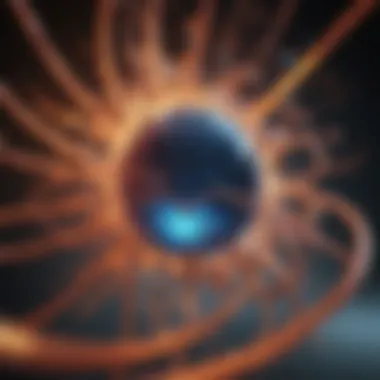
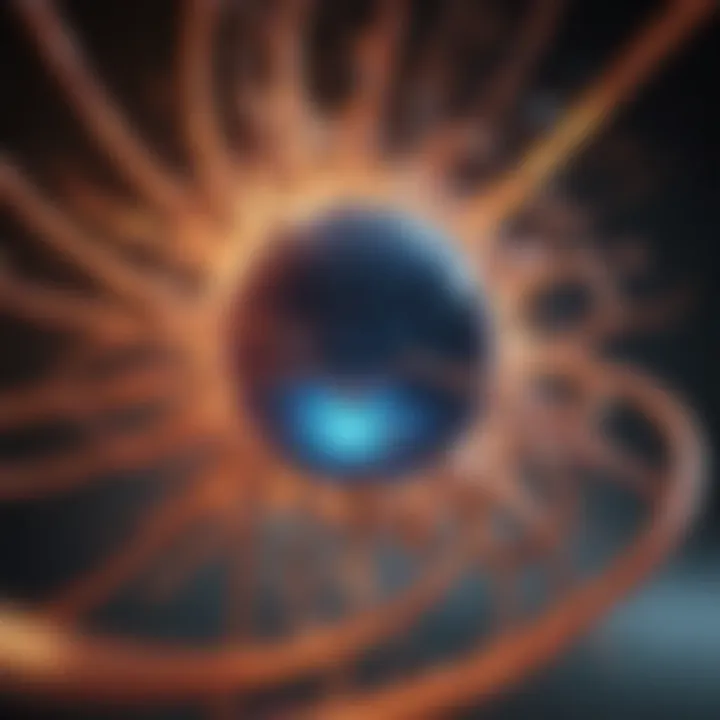
Intro
Thermal conduction is a crucial topic within the field of thermodynamics. It defines how heat moves through materials and is fundamental to various applications in technology and nature. Understanding thermal conduction is imperative for students, researchers, and professionals alike, as it influences both natural phenomena and engineered systems.
This article examines the underlying principles and mechanisms of thermal conduction, providing insightful analysis and practical implications.
Methodology
Study Design
The study of thermal conduction involves observing how heat transfers between materials under various conditions. This includes experimenting with different materials such as metals, ceramics, and polymers. The aim is to understand how their physical properties impact thermal conductivity.
Instruments used in these studies vary from simple calorimeters to advanced thermal imaging systems. These tools help gather data under controlled conditions, ensuring accurate measurement of temperature differences.
Data Collection Techniques
Data collection often employs both qualitative and quantitative methods. Researchers may conduct controlled experiments to measure heat transfer rates while simultaneously noting environmental factors like temperature, pressure, and humidity.
Moreover, computational simulations are frequently used to model thermal behavior in complex systems, offering visual representations of heat flow and material interactions. This aids in predicting how materials will perform in real-world applications.
Discussion
Interpretation of Results
The results often reveal significant insights into how different materials respond to thermal energy. For example, metals typically exhibit higher thermal conductivity than non-metals. This knowledge is pivotal when selecting materials for heat exchangers, insulation, and electronic devices.
Limitations of the Study
Despite extensive research, limitations exist. Not all environmental conditions can be replicated in laboratory settings, which may affect the generalizability of results. Additionally, the inherent properties of new or composite materials can lead to unpredictable behavior during thermal conduction studies.
Future Research Directions
Future research aims to explore nanomaterials and their unique thermal properties. As technology advances, the potential applications for these materials in energy efficiency and thermal management become increasingly relevant. Researchers will likely focus on innovative materials that challenge traditional understandings of thermal conductance and its applications.
Thermal conduction affects numerous fields, including engineering, climate science, and materials development. Its understanding informs better designs and improvements in technology.
In summary, the principles of thermal conduction are essential for a profound comprehension of heat transfer mechanisms. Understanding these concepts not only aids academic pursuits but also enhances practical applications across various sectors.
Prelims to Thermal Conduction
Thermal conduction is a fundamental concept in the field of thermodynamics. It involves the transfer of heat through materials as a result of temperature differences. Understanding this principle is crucial in various scientific fields, as well as in everyday applications. This section will provide clarity on the concept, its significance, and the general context in which thermal conduction operates.
Definition of Thermal Conduction
Thermal conduction can be defined as the process through which heat energy moves within a material or between materials in direct contact. This occurs at the molecular level; high-energy particles transfer their energy to adjacent low-energy particles, causing a flow of heat from the hotter to the cooler region. The efficiency of this transfer is greatly influenced by the material properties such as thermal conductivity. This property quantifies how well a material can conduct heat and is a vital consideration in fields like materials science and engineering.
Historical Context
The study of thermal conduction has evolved over centuries. One of the key figures in this field is Joseph Fourier, a French mathematician, who formulated the Fourierβs Law of heat conduction. His work in the early 19th century provided a mathematical foundation for understanding how heat flows through materials. Early applications of these principles began in industrial settings, where managing heat transfer became crucial for efficiency and safety in machinery. As scientific knowledge has advanced, so too has our understanding of thermal conduction, leading to innovations in technology and materials. Today, we recognize its importance not only in engineering but also in nature, influencing climates and ecosystems.
Understanding thermal conduction is essential in the development of effective thermal insulators and exchangers, impacting energy efficiency in modern systems.
In summary, the principles of thermal conduction form a basis upon which many other concepts are built. A clarity on this topic is essential for anyone looking to delve deeper into thermodynamics, physics, or engineering.
Fundamental Principles of Thermal Conduction
Thermal conduction is a vital aspect of heat transfer, serving as the primary mechanism through which heat is distributed in solids. Understanding the principles behind thermal conduction is crucial for a variety of fields, including engineering, physics, and materials science. It allows professionals to design efficient systems for managing heat, which can lead to improved energy efficiency and performance.
Thermal conduction works on the principle that heat flows from areas of higher temperature to areas of lower temperature. The efficiency of this process depends on the material's properties, including thermal conductivity. As such, grasping these principles enhances the ability to predict and control thermal behaviors in practical applications.
The Law of Fourier
The Law of Fourier is fundamental to the understanding of thermal conduction. It quantitatively describes how heat transfer occurs in materials. According to Fourier's Law, the rate of heat transfer through a material is proportional to the negative gradient of temperature and the area through which the heat is flowing. The law can be expressed mathematically as:
[ q = -k \cdot A \cdot \fracdTdx ]\
Here, ( q ) represents the heat transfer rate, ( k ) is the thermal conductivity, ( A ) is the cross-sectional area, and ( \fracdTdx ) is the temperature gradient. This equation highlights the interplay between material properties and the directional nature of heat flow, underscoring its significance in applications ranging from thermal insulation to heat exchangers.
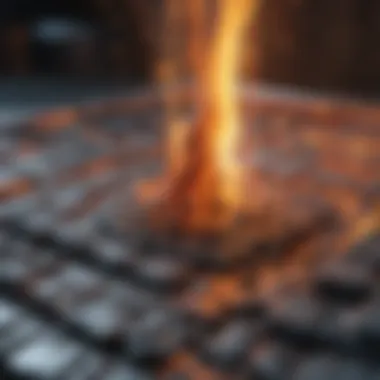
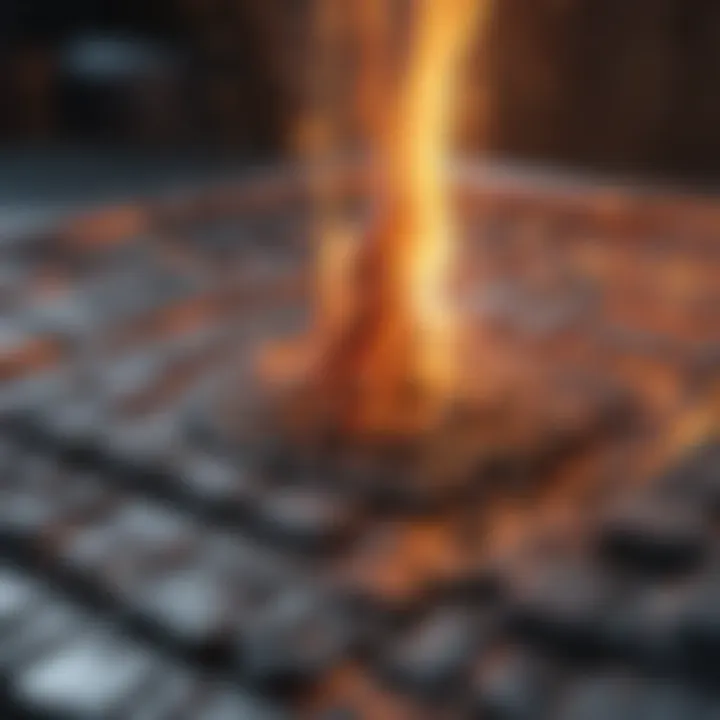
Heat Transfer Mechanisms
Heat transfer occurs through three primary mechanisms: conduction, convection, and radiation. Each mechanism has unique characteristics, advantages, and disadvantages.
Conduction
Conduction is the direct transfer of heat through a material without any movement of the material itself. This process depends heavily on the material's thermal conductivity. As heat energy is added to a region of a material, the particles vibrate more vigorously, transferring energy to neighboring particles. This mechanism proves effective in solids, especially metals, which exhibit high thermal conductivity.
Key Characteristics of Conduction:
- Direct Transfer: Heat moves directly through a material.
- Dependent on Material Properties: Metals like copper and aluminum are excellent conductors, while materials like wood or rubber are poor conductors.
The main advantage of conduction is its efficiency in solid materials for localized heating. However, it is less effective over larger distances or in gases, where convection often proves more effective.
Convection
Convection involves the movement of fluids, either liquids or gases, resulting in the transport of heat. This process can be natural or forced. In natural convection, fluid movement occurs due to density differences caused by temperature variations. In forced convection, external means, such as fans or pumps, induce fluid movement.
Key Characteristics of Convection:
- Movement of Fluid: Unlike conduction, convection requires the bulk movement of the fluid.
- Efficient Heat Distribution: Convection can transfer heat over larger distances more effectively than conduction alone.
Convection is often used in applications like heating systems and cooling circuits. However, its effectiveness can be influenced by factors such as fluid viscosity and flow patterns, which can complicate heat transfer predictions.
Radiation
Radiation is the transfer of heat through electromagnetic waves. Unlike conduction and convection, radiation does not require a medium, allowing heat to transfer through a vacuum. Every object emits some level of thermal radiation based on its temperature, affecting the surrounding environment.
Key Characteristics of Radiation:
- No Medium Required: Heat can transfer through space.
- Depends on Surface Properties: The emissivity of a surface affects how well it radiates heat.
The primary advantage of radiation is its ability to transfer heat across empty space, such as the warmth felt from the sun. However, radiation is less effective at close range compared to conduction and convection.
"Understanding these heat transfer mechanisms is essential for successful thermal management in various applications."
By understanding these fundamental principles, students, researchers, and professionals can better design and optimize systems for effective heat transfer.
Material Properties Affecting Thermal Conduction
Understanding the material properties that influence thermal conduction is crucial. Each material transmits heat differently, affecting performance in applications ranging from household appliances to advanced engineering systems.
Thermal conduction is not solely a physical phenomenon; it is also remarkably dependent on intrinsic properties of the materials involved. This includes their thermal conductivity, specific heat capacity, and density. A detailed examination of each of these factors reveals how they interact with environmental conditions and contribute to the efficiency of heat transfer.
Thermal Conductivity Explained
Thermal conductivity defines how well a material conducts heat. It is quantified by the rate at which heat flows through a unit area in response to a temperature gradient. Materials like metals have high thermal conductivity, making them ideal for heat sinks in electronic devices. In contrast, materials such as rubber exhibit low thermal conductivity and serve as effective insulators. Understanding this property helps in selecting the right materials for specific applications, whether in construction, electronics, or manufacturing.
Factors influencing thermal conductivity include:
- Temperature Effect: As temperature rises, thermal conductivity in some materials can also increase, while it decreases in others.
- Material Structure: Crystalline materials generally have higher thermal conductivity than amorphous materials due to the orderly arrangement of atoms.
- Phase Changes: When materials change from solid to liquid or gas, their thermal conductivity may alter significantly.
Specific Heat Capacity
Specific heat capacity plays a pivotal role in thermal conduction. It refers to the amount of heat required to change a unit mass of a substance by one degree Celsius. This property helps in determining how quickly a material will heat up or cool down when exposed to a heat source. Substances with high specific heat, like water, can absorb substantial amounts of heat before experiencing a notable temperature change, making them pivotal in processes such as refrigeration and heating systems.
The specific heat capacity of materials can influence processes such as:
- Thermal Storage: Materials with high specific heat can store heat effectively, making them useful in passive solar heating applications.
- Energy Efficiency: Understanding specific heat allows engineers to design systems that optimize energy use, reducing waste in industrial applications.
Role of Density
Density is another important factor affecting thermal conduction. In general, denser materials tend to conduct heat better. This is primarily because molecules are more closely packed in denser materials, allowing quicker heat transfer between them.
However, it is important to note that density must be considered along with other properties. For instance:
- Composite Materials: In composite materials, the combination of different densities from various constituents can lead to complex thermal behaviors.
- Porosity: A material may have high density but significant porosity, affecting its overall capacity to conduct heat.
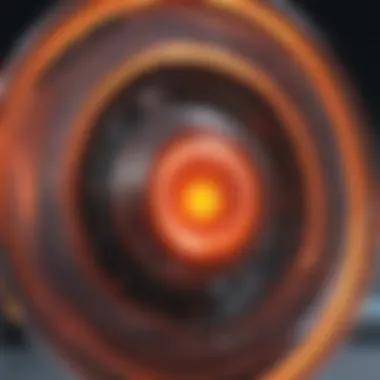
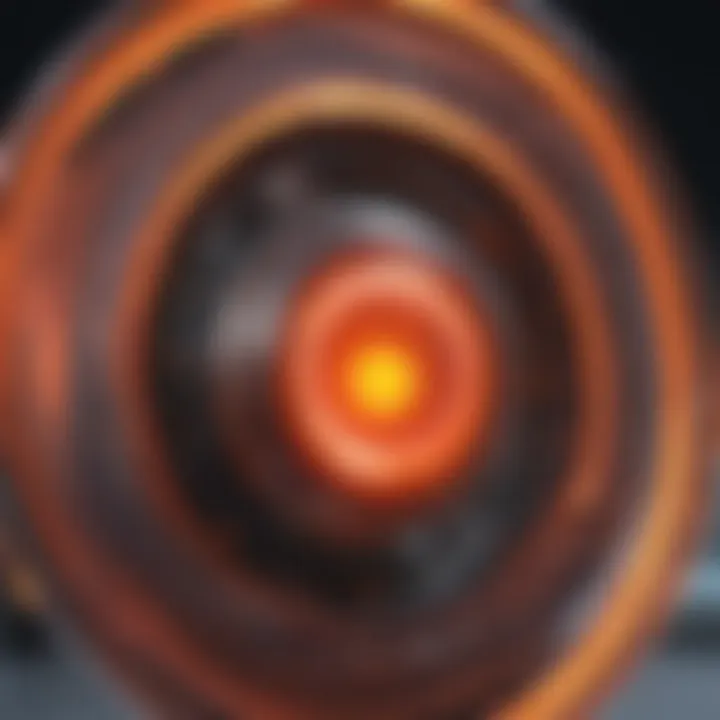
Mathematical Description of Thermal Conduction
Mathematics plays a crucial role in understanding thermal conduction. It gives precise tools to model heat transfer processes. With mathematical formulations, we can predict how heat moves through different materials under various conditions. For students and researchers, mastering these equations helps in grasping complex thermal dynamics. It also facilitates the design of systems where temperature control is vital.
Heat Equation Derivation
The heat equation is a fundamental aspect of thermal conduction. It relates the distribution of temperature in a given material as a function of time and space. This equation is derived from both Fourier's law of heat conduction and the principle of conservation of energy.
The general form of the heat equation is:
[ \frac\partial T\partial t = \alpha \nabla^2 T ]
In this equation, ( T ) represents the temperature, ( t ) is time, and ( \alpha ) is the thermal diffusivity of the material. The term ( \nabla^2 T ) represents the Laplacian operator, indicating how temperature changes with respect to spatial coordinates.
Deriving the heat equation involves several steps. First, consider a small volume of material. Next, apply Fourier's law to relate the heat flux to the temperature gradient. Finally, integrate and simplify the equations under steady-state and transient conditions. This derivation not only provides insight into how heat moves but also establishes a connection between theoretical concepts and real-world applications.
Steady-State vs. Transient Conduction
Understanding the difference between steady-state and transient conduction is important in thermal management. In steady-state conduction, the temperature profile remains constant over time. This means that heat entering a material is equal to the heat leaving it. This scenario is often seen in systems that are operated long enough for transient effects to diminish.
Conversely, transient conduction describes the changes in temperature over time. As heat is added or removed, the material experiences variations until it reaches steady-state. Transient conduction can be analyzed using time-dependent solutions to the heat equation. The initial conditions and boundary conditions significantly influence how quickly the system reaches equilibrium.
In practical applications, knowing when to use steady-state vs. transient analysis is essential for efficient thermal design.
Both concepts hold importance in engineering designs. They affect decisions related to insulation, heating systems, and temperature control mechanisms. Thus, a sound understanding of these distinctions helps inform proper methodologies to ensure effective heat management.
Factors Influencing Thermal Conduction
The study of thermal conduction encompasses various factors that significantly affect how heat is transferred through materials. Understanding these factors is essential for both practical applications and theoretical research. Assessing these influences not only aids engineers and scientists in selecting appropriate materials for specific applications but also informs practices in energy efficiency and thermal management.
Temperature Gradients
Temperature gradients play a pivotal role in thermal conduction. Simply put, the greater the difference in temperature across a material, the more rapid the heat transfer. This phenomenon can be described through Fourier's Law, which asserts that heat transfer is directly proportional to the temperature gradient. In engineering, managing temperature gradients is critical. For example, in insulative materials, maintaining a low gradient ensures that less heat escapes. Conversely, in heat exchangers, high gradients promote efficient heat transfer between fluids.
Effective thermal management often hinges on understanding and controlling temperature gradients across systems.
Surface Area Considerations
The surface area of a material also greatly influences thermal conduction. As the surface area increases, heat can be transferred more efficiently. This principle is particularly relevant in applications like cooking, where pans with larger surfaces allow for quicker heating. In building materials, designs that maximize surface exposure improve energy efficiency. Additionally, the interface between two materials can significantly affect how heat is conducted. For example, joints and connections may introduce thermal resistance, influencing overall performance. Therefore, engineers must carefully consider surface geometry and material interfaces in their designs.
Phase Changes of Materials
Phase changes in materials introduce unique aspects to thermal conduction. When a substance transitions from solid to liquid or from liquid to gas, it absorbs or releases energy, which can affect overall heat transfer capabilities. For example, when ice melts, it requires energy, known as latent heat, without a change in temperature. Understanding these changes enables engineers to utilize materials effectively in thermal storage systems and climate control applications. In nature, phase changes contribute to heat distribution in ecosystems, affecting climates and weather patterns. Hence, thorough comprehension of these transitions is vital for both natural and engineered systems.
Applications of Thermal Conduction
The practical applications of thermal conduction are integral to understanding how heat transfer operates in various environments. This section aims to clarify the role of thermal conduction in engineering, design, and everyday life. Applications are vast and can range from thermal insulation in buildings to efficient heat exchangers in appliances. Studies in thermal conduction are not just academic; they significantly impact efficiency and sustainability in technological developments.
Engineering and Design
Thermal Insulators
Thermal insulators are materials designed to reduce the rate of heat transfer. Their primary function is to maintain temperature and improve energy efficiency. Insulators, such as fiberglass, polystyrene, and cellulose, form an essential part of construction and manufacturing processes. These materials feature low thermal conductivity, which minimizes heat loss or gain. Their ability to keep environments stable makes them popular in various applications, from residential homes to industrial facilities.
One unique feature of thermal insulators is their ability to handle a broad range of temperatures, making them versatile in various geographic locations. However, many insulators may have lower resistance to mechanical stress, potentially limiting their use in certain structural elements. This balance between thermal efficiency and physical durability is a crucial consideration in design and engineering practices.
Heat Exchangers
Heat exchangers are devices designed to transfer heat between two or more fluids without mixing them. In various fields such as HVAC, refrigeration, and power generation, they play a critical role in enhancing energy efficiency. The primary characteristic of heat exchangers is their ability to maximize surface area while minimizing resistance to fluid flow. This quality enhances the rate of heat transfer, thereby optimizing energy consumption.
The unique feature of heat exchangers lies in their design, which can vary widely from simple shell-and-tube designs to complex plate designs. This adaptability allows them to be configured for specific applications, improving overall performance. One disadvantage, however, can be maintenance complexity, especially in large systems where cleaning and inspection are challenging.
Everyday Examples
Cooking Appliances
Cooking appliances like ovens and stovetops rely heavily on thermal conduction for their function. These appliances convert energy into heat, which is then transferred to the food being prepared. A key characteristic of these devices is their ability to maintain consistent temperatures, which is essential for effective cooking. Materials such as cast iron or stainless steel are designed for optimal heat conduction, ensuring even cooking results.
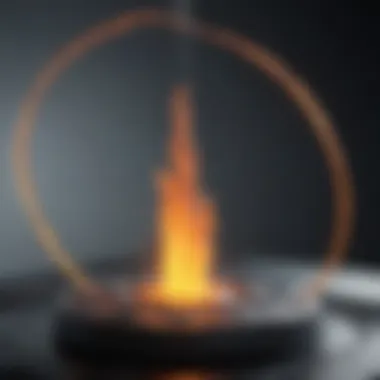
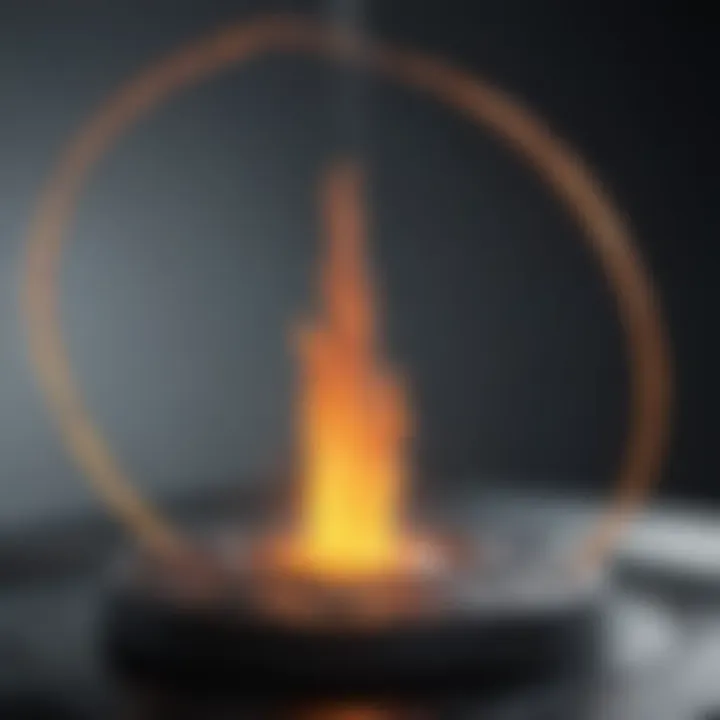
However, the ability to conduct heat can also lead to challenges. For instance, rapid heat loss can occur if appliances are not designed with adequate insulation. This factor may impact energy efficiency over time, highlighting the importance of continued innovations in appliance design.
Building Materials
The choice of building materials directly influences thermal conduction, which affects energy consumption in residential and commercial settings. Materials such as concrete, wood, and brick have distinct thermal properties that dictate how well they conduct heat. A notable characteristic of these materials is their capacity to store heat, which can be beneficial in regulating indoor temperatures.
Additionally, the combination of different materials can enhance or reduce thermal conduction effects in architectural designs. However, the very same properties that allow for good heat conduction can also lead to energy inefficiency if not carefully managed. With rising energy costs and increasing emphasis on sustainability, understanding thermal conduction in building materials is essential for future developments.
Thermal Conduction in Nature
Thermal conduction plays a crucial role in various natural processes. Understanding how heat moves through different mediums helps clarify many phenomena we observe in our environment. This aspect of thermal dynamics is essential not only for academic study but also for practical applications in ecology, geology, and climate science.
Geothermal Heat Transfer
Geothermal heat transfer refers to the movement of heat from the Earth's interior to its surface. This process is primarily driven by thermal conduction through various geological materials. The heat originates from the decay of radioactive isotopes and residual heat from Earth's formation. This geothermal energy can be harnessed for heating and electricity generation.
In regions with volcanic activity, geothermal gradients are particularly high. For example, in Iceland, the geothermal energy is utilized extensively for power generation and district heating. Here, the balance between heat conduction and other forms of heat transfer, such as convection, determines the effectiveness of geothermal systems.
Studies show that geothermal energy is a reliable and sustainable energy source. It can significantly reduce reliance on fossil fuels. However, understanding the thermal properties of rocks and soil formations is critical for efficient geothermal system design.
Thermal Regulation in Animals
Animals adapt to their environments partly through mechanisms of thermal regulation. Thermal conduction assists in how animals maintain their body temperature. For instance, mammals and birds often use conductive heat transfer to regulate their core temperatures. When an animal comes into contact with a cooler surface, it loses heat via conduction. Conversely, if it rests on a warm surface, heat moves into its body, assisting in warming it up.
Different species have evolved unique adaptations for thermal regulation. For example, elephants possess large ears filled with blood vessels. The thin skin allows heat to dissipate effectively, maintaining a stable internal temperature. In contrast, small mammals may burrow underground where the earth maintains more constant temperatures, minimizing heat loss to the ambient environment.
In understanding thermal regulation, researchers examine behavioral adaptations as well. Some animals, like reptiles, will bask in sunlight to absorb heat through conduction, optimizing their body temperature for energy efficiency. This behavioral aspect emphasizes the diverse strategies living organisms use to navigate thermal challenges.
"Thermal conduction is not only vital in geology but crucial in understanding the biological adaptations of organisms to their environment.β
Innovations and Future Directions
Innovations in thermal conduction technology have significant implications for energy efficiency and climate sustainability. As the world focuses on reducing energy consumption and mitigating environmental impacts, advancements in thermal management are becoming crucial. New materials and approaches can enhance heat transfer processes, leading to more effective systems in various fields, such as construction, electronics, and energy production.
Nanomaterials and Thermal Management
Nanomaterials have emerged as a transformative force in thermal conduction. Their unique physical characteristics enable them to exhibit enhanced thermal properties compared to traditional materials. This is largely due to their high surface area-to-volume ratios, which facilitate more effective heat transfer. Among these nanomaterials, graphene and carbon nanotubes are particularly notable. They demonstrate exceptional thermal conductivity, making them suitable for applications ranging from electronics cooling to thermal insulation in building materials.
The integration of nanomaterials into existing systems can result in significant performance improvements. For instance, in electronic devices, improved thermal management can help dissipate heat more effectively. This not only enhances performance but also prolongs the lifespan of these devices. Moreover, when blended with polymers, nanomaterials can create composites that maintain light weight while improving strength and thermal efficiency.
However, the application of nanomaterials in real-world scenarios is still being explored. Challenges remain, particularly concerning the scalability of production and the long-term stability of these materials. Regulators are also evaluating the environmental impact of manufacturing and disposing of nanomaterials. Solutions to these problems will dictate the pace of adoption.
Sustainable Heating Solutions
With the pressing need for sustainable energy practices, innovations in heating technologies are essential. New solutions focus on using renewable resources more effectively. For instance, the integration of phase change materials can store and release thermal energy, optimizing heating applications in buildings. Materials like paraffin wax can absorb heat and gradually release it, contributing to a more balanced indoor climate.
Likewise, advancements in heat pump systems allow for greater efficiency in temperature regulation. These systems can be fitted with smart technologies that optimize operation based on demand, thus reducing unnecessary energy consumption. As energy costs rise and environmental concerns grow, these sustainable heating methods represent a positive shift.
"The future of thermal conduction innovation lies in balancing efficiency with environmental stewardship."
Culmination
The conclusion section holds significant importance in this article as it encapsulates the core insights gathered on thermal conduction. Summarizing key points allows readers to gain a clear perspective on the importance of understanding thermal conduction in various applications. This knowledge contributes to better designs in engineering, advancements in technology, and an enhanced understanding of natural processes.
Firstly, it is essential to recognize how thermal conduction serves as a primary mechanism for heat transfer. The effective management of heat is crucial in many contexts, including manufacturing and everyday utilities. Engineers and designers rely on knowledge of thermal properties to optimize material choices, which aids in developing more efficient systems.
Moreover, a thorough understanding of thermal conduction can lead to innovations in sustainable technologies. This can impact areas like energy efficiency in homes or heat management in electronic devices. Recognizing these principles gives rise to better solutions that cater to modern-day challenges, such as climate change and resource conservation.
In summary, the conclusion brings together the fundamentals discussed, reinforcing their relevance and implications.
Summarizing Key Insights
In this article, we have explored several critical insights about thermal conduction:
- Definition: Thermal conduction is the transfer of heat through materials due to a temperature gradient.
- Mechanisms: It operates primarily through conduction, convection, and radiation, with conduction being fundamental in solids.
- Material Properties: The thermal conductivity, specific heat capacity, and density of materials significantly influence the conduction efficiency.
- Mathematical Models: The heat equation and distinctions between steady-state and transient models provide frameworks for understanding thermal behavior.
- Applications: Various fields leverage thermal conduction principles, from engineering designs to everyday appliances.
- Natural Processes: Natural systems, including geothermal activity and animal behavior, exhibit the principles of thermal conduction in action.
These insights serve not only as a reminder of the key concepts but also pave the way for further discussions on the practical implications of thermal conduction.
Implications for Future Research
The study of thermal conduction remains relevant, with several avenues for future exploration. Here are some areas worth considering:
- Nanomaterials: Innovations in nanotechnology hold the potential for enhanced thermal management solutions. Research on how nanomaterials can manipulate heat conduction properties could lead to significant advancements in various industries.
- Climate Impact: Investigating thermal conduction in natural environments may reveal deeper insights into climate dynamics. Understanding how different materials interact thermally with their surroundings can help address environmental challenges.
- Smart Materials: The development of smart materials that respond to thermal changes could revolutionize heating and cooling systems. Exploring how these materials can integrate with current technologies might lead to more efficient thermal management.
- Energy Sources: Future research can also delve into thermal conduction's role in renewable energy systems. Assessing how heat transfer affects solar panels or geothermal systems could enhance energy efficiency.
Overall, these implications point toward significant opportunities to enrich the existing body of knowledge around thermal conduction, fostering advancements that benefit science and society.