Understanding Gene Defects: Mechanisms and Innovations

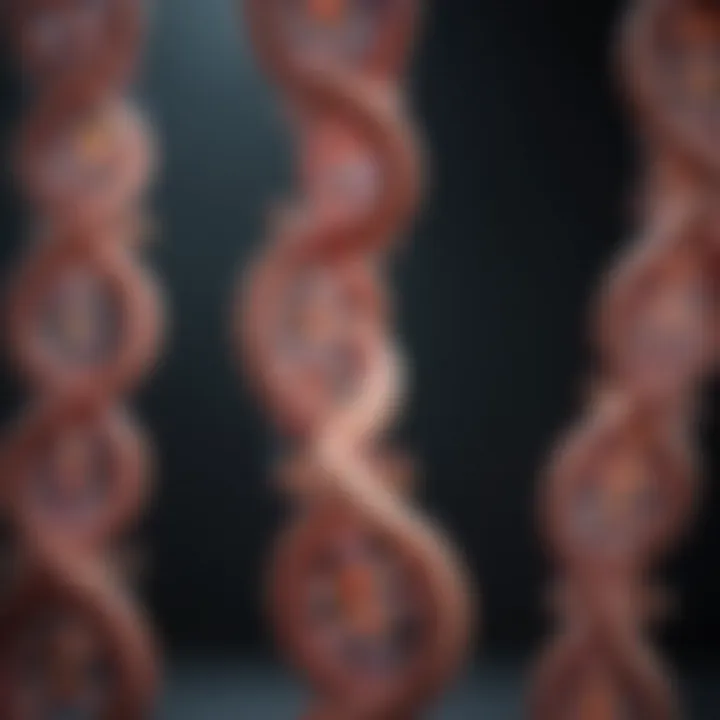
Intro
Gene defects represent a significant aspect of genetics that have profound implications for individual health and the broader population. These defects can be inherited from parents or arise sporadically, contributing to a range of genetic disorders that challenge both individuals and healthcare systems. Understanding the mechanisms behind gene defects is essential for unraveling their complexity and finding effective treatments.
This article aims to explore the various types of gene defects, their implications for human health, and the innovations in research aimed at addressing these issues. It draws attention to the critical nature of studying inherited versus acquired defects, and how they manifest in various phenotypes. Through examining current advancements in genetic research, this article highlights the future directions in improving the understanding of gene defects and developing novel therapies.
In the following sections, a detailed exploration will unfold around the methodologies used to study gene defects, how to interpret findings, the inherent limitations in the research field, and potential future research trajectories.
Preface to Gene Defects
Gene defects are a fundamental component of genetics and medicine. They play a significant role in understanding how genetic anomalies can affect health, development, and overall well-being. The exploration of gene defects leads to a better grasp of various genetic disorders, their causes, and potential treatments.
The importance of studying gene defects extends far beyond academic inquiry. For patients and families, understanding the nature of these defects provides essential insights into inherited conditions and the implications for future generations. Moreover, gene defects can have profound societal implications, influencing healthcare policies and approaches to disease prevention.
All these aspects make the study of gene defects vital, not just for researchers but for anyone interested in the intersection of genetics and health.
Definition and Significance
A gene defect refers to any alteration in the DNA sequence that can disrupt normal gene function. These defects can manifest as mutations, which can lead to improper protein formation and, subsequently, various health issues. Gene defects may be inherited from one or both parents or can occur de novo in an individual’s genome.
The importance of gene defects lies in their direct relationship to countless genetic disorders. Conditions such as cystic fibrosis, sickle cell disease, and Huntington’s disease stem from specific genetic mutations. In this respect, understanding gene defects is crucial for diagnosis, management, and potential therapies.
Historical Context
The history of gene defects dates back to early observations of hereditary diseases. Gregor Mendel's work in the 19th century laid the foundational knowledge of heredity. His experiments with pea plants illustrated how traits are passed down through generations. However, the true understanding of gene defects emerged in the 20th century with the discovery of DNA as the genetic material.
The advent of molecular biology enabled the identification of specific mutations linked to various diseases. Techniques such as DNA sequencing and genetic mapping have advanced the field considerably, allowing scientists to pinpoint exact gene defects with greater accuracy. This historical progression has paved the way for innovations in genetic research and treatments, enhancing the understanding of gene defects throughout time.
Mechanisms of Gene Defects
Understanding the mechanisms of gene defects is crucial for deciphering how genetic anomalies lead to various disorders. This section provides a deep dive into the molecular basis of gene defects and the types of mutations that can occur. It is essential to grasp these concepts as they lay the foundation for the implications gene defects have on health and the potential for research innovations.
Molecular Basis of Gene Defects
The molecular level of gene defects involves alterations in the structure and function of DNA. These alterations can be caused by various factors including environmental influences, errors during DNA replication, or inherited genetic predispositions. At their core, gene defects arise from changes in nucleotide sequences. These changes might affect the production of proteins that are vital for cellular functions. Understanding these molecular mechanisms allows for better insight into the nature of genetic disorders. As a result, it can guide effective therapeutic approaches.
Types of Mutations
Mutations are the primary means through which gene defects manifest. They can be broadly classified into three categories: substitution, insertion, and deletion mutations.
Substitution Mutations
Substitution mutations involve the replacement of one base pair with another in the DNA sequence. This type of mutation can lead to three potential outcomes: a silent mutation that does not change the amino acid sequence, a missense mutation where the amino acid is changed, or a nonsense mutation that introduces a premature stop codon. This mutation type is significant because it can directly alter protein functions. Their simplicity and common occurrence make substitution mutations a critical aspect of genetic analysis within this article.
Insertion Mutations
Insertion mutations occur when one or more nucleotides are added into the DNA sequence. This can disrupt the reading frame of the gene, potentially leading to a completely different translation of subsequent amino acids. The notable feature of insertion mutations lies in their ability to result in significant functional changes in proteins. Understanding this mutation type is essential, as it can provide insights into how certain genetic disorders develop and progress.
Deletion Mutations
Deletion mutations involve the removal of nucleotides from the DNA sequence. Similar to insertion mutations, deletions can also disrupt the reading frame, resulting in altered protein synthesis. They are often associated with serious genetic disorders, as they frequently disrupt essential genes, leading to a loss of function. Deletion mutations highlight the fragility of the genetic code and their implications for health and disease.
Epigenetic Factors
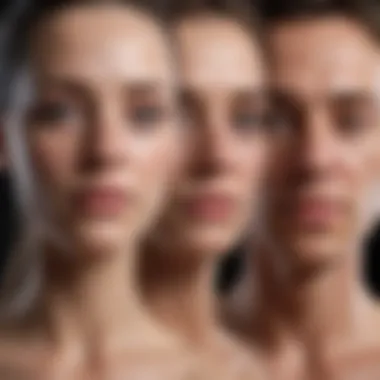
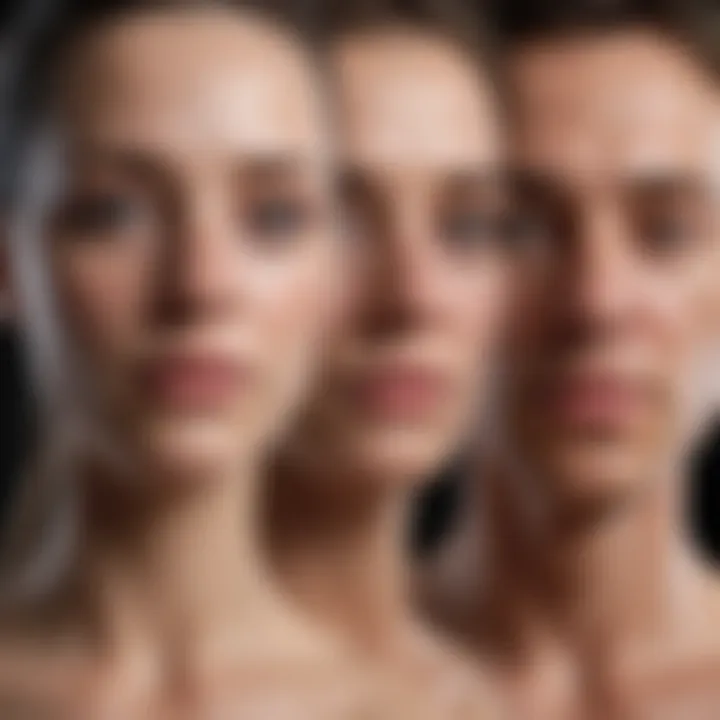
Epigenetic factors refer to modifications that affect gene expression without altering the underlying DNA sequence. These modifications can be influenced by environmental factors such as diet and stress. They can play a significant role in the expression of genetic disorders and can also result in transgenerational effects. Understanding these factors enhances our comprehension of how gene defects manifest in complex ways. Intelligence in addressing epigenetic changes can pave the way for innovative therapies targeting gene defects at multiple levels.
Classification of Gene Defects
Classifying gene defects is crucial for understanding their underlying mechanisms and impacts on health. This classification helps in recognizing specific genetic disorders and tailoring individual treatment strategies. Knowing the type of gene defect can aid researchers and clinicians in predicting disease progression, severity, and even response to therapies.
Different types of gene defects can be broadly categorized into two main groups: inherited defects and acquired defects. Each category carries unique characteristics and implications for affected individuals and their families. Understanding these distinctions allows for better public health strategies, genetic counseling, and research focus.
Inherited Defects
Inherited defects arise from mutations that are passed from parents to offspring. These defects can manifest themselves in various ways, leading to different kinds of genetic disorders. Three notable types of inherited defects are autosomal dominant, autosomal recessive, and X-linked disorders.
Autosomal Dominant
Autosomal dominant defects are notable for their pattern of inheritance. A single copy of the mutated gene can cause the disorder. This makes it easier for the trait to be passed on to the next generation, leading to a higher prevalence in the population. One key characteristic of autosomal dominant disorders is that they often appear in every generation, affecting males and females equally.
A specific example is Huntington's disease, which presents symptoms typically in mid-adulthood. The unique feature here is the clear inheritance pattern, which can be beneficial for genetic counseling. However, the disadvantage is the high probability that children of an affected individual are at risk to inherit the condition.
Autosomal Recessive
In contrast, autosomal recessive defects require two copies of the mutated gene for the disorder to manifest. This means that both parents must pass on the defective gene for a child to be affected. A prominent characteristic of autosomal recessive disorders is that they can appear unexpectedly within a family tree, potentially skipping generations.
Cystic fibrosis serves as a prime example. It illustrates the unique aspect of carriers—individuals who possess one mutated gene but do not show symptoms. This characteristic can be beneficial for population screening, identifying at-risk couples. However, the main disadvantage is the often severe health implications for affected individuals, who may struggle with chronic illness.
X-Linked
X-linked defects, on the other hand, are connected to genes located on the X chromosome. These defects often disproportionately affect males, who have only one X chromosome. In contrast, females can be carriers and may or may not exhibit symptoms. The unique characteristic of X-linked disorders is this gender susceptibility, which is important when discussing inheritance patterns.
Hemophilia is a classic example of an X-linked disorder, impacting blood clotting. The advantage here lies in potential gene therapies that specifically target the defective gene on the X chromosome. However, the disadvantage is that affected males may face significant health issues without timely treatment.
Acquired Defects
Acquired defects, unlike inherited ones, develop at some point during a person’s life due to environmental factors, lifestyle choices, or other external influences. These may include exposure to radiation, chemicals, or infections that lead to mutations. While they do not follow a predictable inheritance pattern like inherited defects, understanding them is equally important for public health and prevention strategies.
A clear distinction is that acquired defects are not generally passed down to future generations. This aspect emphasizes the necessity for education and awareness of environmental triggers and lifestyle factors that can lead to mutations.
Understanding both inherited and acquired defects provides a well-rounded view of the complexities involved in gene defects. By classifying them, we can better inform research efforts and treatment strategies.
Examples of Genetic Disorders
Genetic disorders are critical in understanding gene defects, as they provide clear examples of how specific mutations can result in significant health impacts. Studying these disorders allows for insights into underlying mechanisms of gene function and the corresponding consequences when these functions are disrupted. This section will explore notable examples of genetic disorders, highlighting their unique characteristics, genetic basis, and broader implications for affected individuals and society.
Cystic Fibrosis
Cystic fibrosis is an autosomal recessive disorder caused by mutations in the CFTR gene, which encodes a protein responsible for regulating salt and water transport across cell membranes. The most common mutation is the deletion of phenylalanine at position 508, referred to as F508del.
Patients with cystic fibrosis typically experience respiratory challenges due to thick mucus accumulation in the lungs, leading to recurrent infections and chronic inflammation. In addition to pulmonary complications, this condition can affect the digestive system, leading to nutrient malabsorption and challenges in maintaining a healthy weight.
Management strategies primarily focus on maintaining lung function and improving quality of life. This includes physical therapy, inhaled medications, and potential lung transplantation in severe cases. Research advancements have led to the development of CFTR modulators, such as ivacaftor and lumacaftor, which aim to correct the malfunctioning protein.
Sickle Cell Disease
Sickle cell disease is an inherited blood disorder resulting from a mutation in the HBB gene, which produces the beta-globin subunit of hemoglobin. Individuals with this disorder typically have red blood cells that assume a sickle shape, particularly when oxygen levels are low.

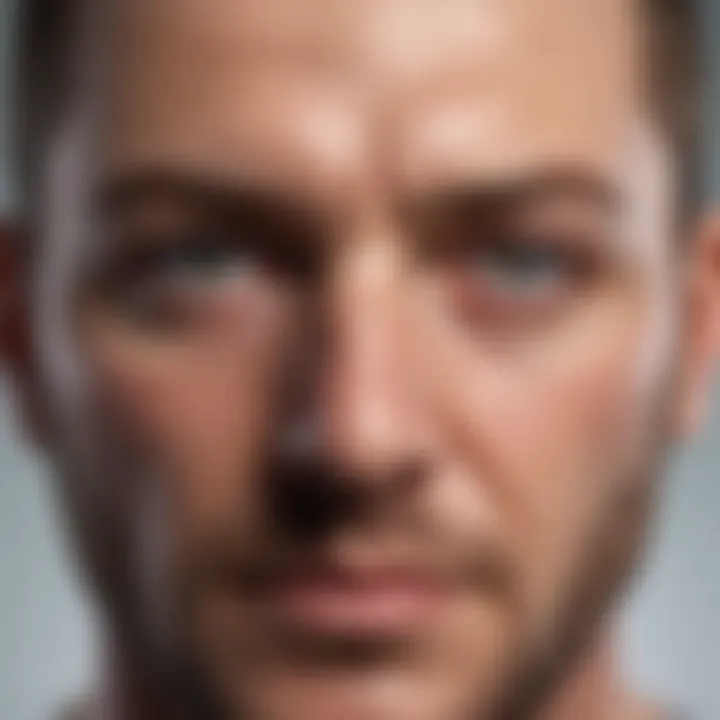
The sickle-shaped cells can lead to various complications, including anemia, pain crises, and increased risk of infections. These complications arise from the cells' tendency to block blood flow, which can damage tissues and organs over time.
Management includes pain management, hydration, and preventive care, such as vaccinations. Hematopoietic stem cell transplantation offers a potential cure for certain patients, but it requires careful selection and availability of compatible donors. Increasing research focuses on gene therapy approaches, which aim to correct the underlying genetic defect directly.
Huntington's Disease
Huntington's disease is a rare hereditary neurodegenerative disorder caused by an expansion of CAG repeats in the HTT gene. This leading to the production of a toxic protein that progressively damages neurons in specific brain regions, particularly those involved in movement and cognition.
Symptoms usually appear in mid-adulthood and include chorea, cognitive decline, and psychiatric issues. As the disease progresses, individuals lose the ability to carry out daily activities and require significant care.
Currently, there is no cure for Huntington's disease, but advancements in genetic counseling and supportive therapies can help manage symptoms. Ongoing research into potential disease-modifying therapies continues to offer hope for future treatments aimed at halting or reversing the progression of the disease.
Down Syndrome
Down syndrome, also known as trisomy 21, is a chromosomal disorder caused by the presence of an extra copy of chromosome 21. This genetic condition commonly leads to various developmental changes and health challenges, including physical, cognitive, and behavioral adaptations.
Individuals with Down syndrome typically exhibit characteristic facial features, development delays, and an increased risk of certain medical conditions, such as congenital heart defects and thyroid disorders. Early intervention, including educational and therapeutic support, can significantly enhance development and quality of life for those affected.
The implications of Down syndrome extend beyond health, influencing societal perceptions and support systems for affected individuals and their families. Advances in prenatal screening have also shifted both ethical discourse and family planning considerations surrounding this condition.
Understanding these disorders not only underscores the complexity of genetic defects but also highlights the ongoing need for research and innovative approaches in genetics.
Implications of Gene Defects on Health
The analysis of gene defects holds deep significance for understanding human health. The mechanisms of gene defects can result in a variety of health issues, from mild to severe. Understanding these implications goes beyond the biological effects; it also encompasses the psychosocial aspects, the impact on family dynamics, and broader epidemiological trends. This multi-dimensional viewpoint allows researchers and healthcare practitioners to address not only the physical manifestations of these defects but also the emotional and social consequences they entail.
Phenotypic Consequences
Gene defects can lead to distinct phenotypic outcomes. These outcomes are visible traits that arise from the interaction between an individual's genetic makeup and environmental influences. For instance, the presence of a mutation in the cystic fibrosis transmembrane conductance regulator (CFTR) gene leads to cystic fibrosis, characterized by respiratory complications and pancreatic issues. Other disorders, such as sickle cell disease, provide altered red blood cell shapes, leading to various health complications.
The severity of phenotypic consequences depends on factors like the type of mutation and the gene involved. Some defects may result in observable characteristics, while others may remain latent or manifest later in life. This variability further complicates diagnosis and treatment strategies. It is essential to understand these phenotypic expressions to provide targeted therapeutic interventions.
Psychosocial Considerations
The implications of gene defects extend into the psychosocial realm. Individuals with genetic disorders may experience significant emotional distress. There are challenges such as stigma and discrimination, which can impact their social interactions and overall quality of life. Families also bear the burden of adjustment to the diagnosis, which can strain relationships and lead to feelings of isolation.
Support systems play a crucial role in mitigating these challenges. Genetic counseling can help in navigating the psychological and emotional processes involved. Providing education and resources about specific disorders can empower affected individuals and families. Building community support networks can also foster a sense of belonging and understanding among those facing similar challenges.
Epidemiological Insights
Gene defects carry implications that can be observed at the population level. Understanding these insights enhances the comprehension of public health. For instance, certain genetic disorders exhibit higher prevalence in specific populations due to historical or geographical factors. This awareness enables more effective screening and prevention strategies tailored to at-risk groups.
Furthermore, tracking the epidemiology of genetic disorders allows researchers to identify trends and the effects of environmental factors on gene expression. Data from large-scale studies can guide policy and healthcare decisions aimed at managing genetic disorders more effectively.
Epidemiological studies inform us that knowledge of genetic defects can lead to improved healthcare strategies and interventions, helping target at-risk populations efficiently.
Through the integration of these various perspectives, the implications of gene defects on health reveal a complex tapestry of biological, emotional, and social dimensions. Recognizing these interconnected elements is essential for advancing both scientific inquiry and practical healthcare approaches.
Research Advances in Gene Defects
Research into gene defects is vital for unraveling the complex interactions that lead to various genetic disorders. Delving into this field provides a more profound understanding of the underlying causes of these conditions. Moreover, the advancements focus not only on identifying gene defects but also on developing effective therapies to mitigate their impact. This section examines key technologies and methodologies that represent significant progress in this area.
Genome Editing Technologies
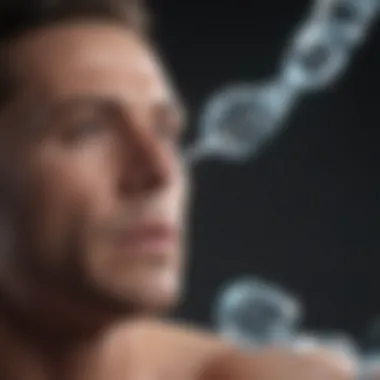
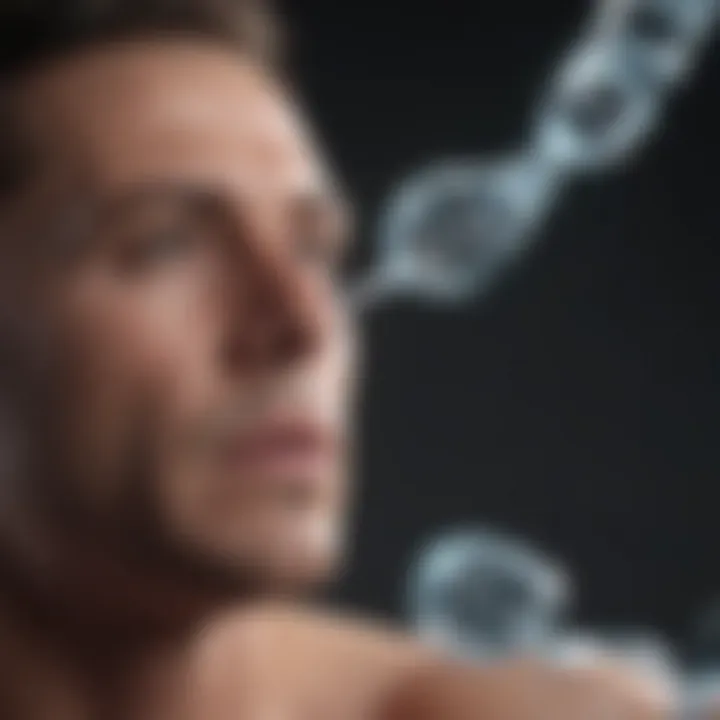
CRISPR-Cas9
CRISPR-Cas9 is a revolutionary tool in genetics that has transformed the landscape of gene editing. This method stands out due to its simplicity, efficiency, and accuracy in modifying genes within living organisms. The specific aspect of CRISPR-Cas9 that is noteworthy is its ability to target precise locations in the genome, allowing for specific changes to DNA sequences. It has gained popularity for its ease of use and the potential to treat genetic disorders at the source.
A critical characteristic of CRISPR-Cas9 is its guiding RNA, which directs the Cas9 protein to the exact site in the DNA to be edited. This feature makes it distinct from other gene editing technologies, providing a more direct approach to genetic modification. However, no technology is without its limitations. One disadvantage of CRISPR-Cas9 is the potential for off-target effects, where unintended changes can occur in the genome, leading to unforeseen consequences. Thus, while it holds promise, careful evaluation of its application is necessary.
TALENs
TALENs (Transcription Activator-Like Effector Nucleases) represent another powerful approach to gene editing. This method is particularly noted for its ability to create targeted double-strand breaks in DNA, which can lead to gene knockout or insertion. TALENs provide a high level of specificity and may be preferred in scenarios where accuracy is paramount.
One notable aspect of TALENs is their flexibility in design, which allows researchers to construct nucleases for numerous gene targets. This flexibility contributes to their appeal as a beneficial choice in gene editing research. However, they require a more complex synthesizing process compared to CRISPR-Cas9, making them somewhat less accessible. Another challenge with TALENs is their efficiency compared to CRISPR, which can affect the rate of successful gene modifications.
Gene Therapy Approaches
Gene therapy aims to treat or prevent disease by manipulating the genes involved. This approach has shown promise for addressing both inherited and acquired gene defects. Methods include introducing a healthy copy of a gene, repairing or disabling a problematic gene, or even replacing missing genes. Recent advancements in this area have led to treatments for conditions previously deemed untreatable.
Predictive Genetic Testing
Predictive genetic testing has emerged as a crucial tool for assessing the risk of genetic disorders. This testing involves analyzing an individual’s DNA to identify gene mutations linked to specific diseases. The implications are profound, as they can guide healthcare decisions and preventative strategies. However, it also raises ethical concerns regarding privacy and the psychosocial impact on individuals and families. As research continues, understanding these advances remains imperative.
Ethical Considerations
Ethical considerations in the context of gene defects are vital for several reasons. As advancements in genetic research and technology progress, it becomes essential to address the ethical implications that arise. These considerations help guide the development of policies that ensure responsible use of genetic information, protect individuals, and advance scientific knowledge while considering public sentiment and social norms.
Gene Editing Ethics
The ethics surrounding gene editing are complex and multifaceted. With technologies such as CRISPR-Cas9 emerging, the ability to modify genes opens up possibilities for correcting inherited defects. However, this capability also raises significant ethical questions. For instance, the concept of 'designer babies'—the idea of selecting specific traits for unborn children—is contentious. It poses dilemmas about equity, access, and potential long-term impacts on human evolution.
Additionally, gene editing can lead to unintended consequences. Off-target effects may inadvertently alter other genes, resulting in unforeseen health issues. There is a pressing need for robust ethical frameworks to evaluate when and how gene editing should be performed. Experts from various fields must collaborate to create guidelines that prioritize safety and ethical integrity, ensuring the public can trust the research.
Privacy in Genetic Testing
As genetic testing becomes more accessible, privacy concerns arise. Individuals may undergo genetic testing for various reasons, including predicting susceptibility to diseases. Such information is deeply personal and can significantly affect one’s life, including life insurance and employment prospects. The potential misuse of genetic data by companies and governments creates ethical dilemmas regarding data ownership and consent.
Protecting individuals' right to privacy is necessary to build trust in genetic testing technologies. Policies must ensure that genetic information is kept confidential, and individuals remain informed about how their data is utilized. Public education campaigns can aid in raising awareness about the importance of privacy in genetics, encouraging responsible sharing of information.
"The right to privacy ensures that individuals can control their own genetic information, fostering a safe environment for scientific inquiry."
The End
In the examination of gene defects, the importance of the conclusion section cannot be understated. It encapsulates the critical findings discussed throughout the article. Understanding gene defects not only furthers scientific inquiry but also lays the groundwork for applied research and patient care. Breaking down complex topics into manageable segments reveals how intricate genetic mechanisms operate and how they directly influence human health.
The exploration of mechanisms such as molecular changes and types of mutations offers vital insights into how specific disorders manifest. Moreover, understanding the implications at various levels—biological, psychological, and sociological—speaks to the far-reaching effects of genetic anomalies on individuals and communities.
Future Directions
Looking ahead, future directions in the study of gene defects appear promising. The application of advanced genome editing technologies, such as CRISPR-Cas9, potentially transforms the landscape of treatment for genetic disorders. This technology allows for precise modifications to DNA, providing a pathway for correcting mutations responsible for various diseases. Furthermore, as these techniques mature, ethical guidelines must evolve alongside them to ensure responsible use.
Additionally, the advent of personalized medicine, which tailors treatment based on individual genetic profiles, signifies a shift toward more effective healthcare solutions. Research focusing on understanding varied genetic expressions and responses to therapies can refine approaches in both inherited and acquired conditions. Investigating the role of epigenetics also indicates how environmental factors can influence gene expression and, consequently, health outcomes.
Call for Further Research
A sustained call for further research is essential in this domain. Continuous investigations into gene defects will illuminate the complexities that genetic variations hold. Addressing gaps in knowledge about specific disorders is critical, as is studying broader correlations between genetics and environmental influences.
Emphasis should also be placed on developing diagnostic tools that offer precision in identifying gene defects earlier in life. A proactive approach facilitates interventions that can optimize health outcomes.
Engaging the global research community is key. Collaboration across institutions and countries can lead to comprehensive studies that generate a richer understanding of genetic disorders. Advocating for funding in innovative projects can address the pressing needs within this field.
In summary, the conclusion of this article underscores the significant strides made in understanding gene defects while simultaneously identifying areas ripe for exploration. Continuous efforts in research and investigation in this vital field are not just academic pursuits; they have the potential to improve countless lives affected by genetic disorders.