Transfection in Genetics: Mechanisms and Applications
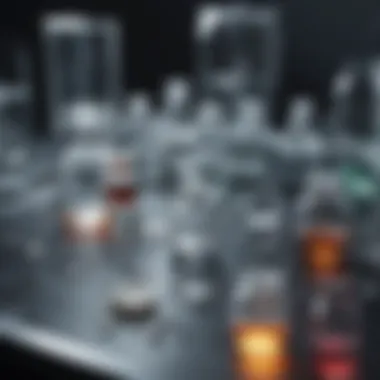
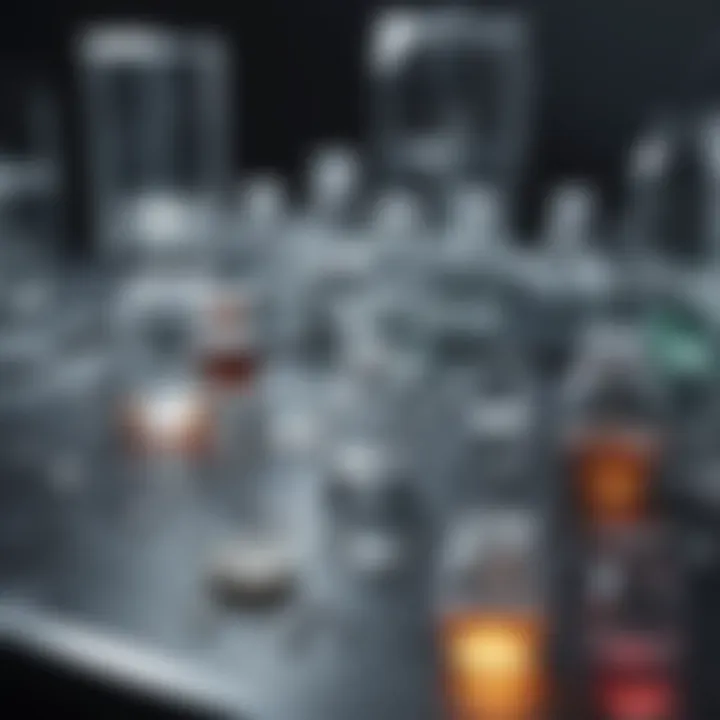
Intro
Transfection stands as a pivotal process in genetics, serving as a gateway for manipulating cellular function by introducing foreign nucleic acids into cells. Whether it be for research purposes, such as studying gene function, or for therapeutic applications, like gene therapy, understanding transfection’s mechanics is crucial. The journey of transfection involves various methodologies and delivery systems that have evolved significantly over the past decades. With a focus on bridging theoretical insights and concrete applications, this article ventures into the intricate world of transfection, detailing its relevance and influence in biotechnology and molecular biology.
Methodology
Study Design
The study of transfection techniques necessitates a structured approach to dissecting methodologies. Researchers often employ laboratory experiments to evaluate both the efficiency and effectiveness of various methods. Experimental designs may include comparative studies that analyze the performance of different transfection agents such as liposomes, electroporation, and viral vectors. These setups allow for a direct observation of variables like transfection rate and cell viability, providing a clearer picture of the nuances involved in each technique.
Data Collection Techniques
The collection of data in transfection studies typically involves utilizing several techniques tailored to the particular aspect of transfection being analyzed. A few methods include:
- Quantitative PCR (qPCR) for measuring the expression levels of transfected genes.
- Fluorescence microscopy to visualize cells that have successfully taken up the transfected material.
- Flow cytometry for assessing cell populations that were effectively transfected.
By combining these techniques, researchers are better equipped to evaluate not just the transfection rates but also the downstream effects on gene expression and cell behavior.
Discussion
Interpretation of Results
Examining the outcomes from various transfection experiments reveals not only the success rates but also informs researchers about the optimum conditions for each method. For instance, certain cell types may exhibit significantly higher transfection efficiencies when specific lipid-based delivery systems are employed. This interpretation is vital for tailoring future experiments and applications in therapeutics.
Limitations of the Study
Despite the advancements in transfection technology, there are inherent limitations that can affect study outcomes. Factors such as cell type, transfection agent used, and environmental conditions can skew results. Moreover, some methods may lead to unintended cellular responses, complicating the interpretation of data. Recognizing these limitations aids in fostering a more nuanced understanding of results.
Future Research Directions
As the field of molecular biology burgeons, there is a continuous push for refining transfection techniques. Future research could focus on developing less toxic and more efficient delivery systems, enhancing precision in targeting specific cell populations. In addition, the integration of advanced imaging methods could provide deeper insights into the real-time processes during transfection, making it an exciting area for ongoing exploration.
"The adaptability of transfection approaches reinforces the dynamic nature of genetic research, often paralleling advancements in technology."
In summary, this section outlines the important methodologies employed in transfection studies, including the strategies for data collection and the vital discussions surrounding interpretation, limitations, and future directions. A clear grasp of these components not only heightens the overall understanding of transfection but also underpins its broader applications in genetics.
Prelims to Transfection
Transfection has emerged as a cornerstone in genetics and molecular biology, serving a multitude of purposes in both research and therapeutic domains. It’s not merely a technique; it’s a pivotal process that allows scientists to manipulate genetic material with precision. Understanding transfection is like having the key to unlock layers of cellular mechanisms—whether it's for developing gene therapies or advancing vaccine research. Grasping its significance not only enriches one’s knowledge but also bridges theoretical genetics with tangible, real-world applications.
Definition and Importance
At its core, transfection refers to the introduction of nucleic acids—such as DNA or RNA—into cells. This process enables the expression of target genes and allows the study of gene function and regulation. It’s especially vital in the age of personalized medicine, where tailored treatments are becoming more prevalent.
The importance of transfection can’t be overstated:
- Gene Therapy: One of the most exciting applications is in gene therapy, where it can potentially correct genetic defects.
- Research Advancements: In basic research, it’s used to dissect pathways and understand disease mechanisms.
- Vaccine Development: With the advent of mRNA vaccines, the role of transfection has taken on new significance, emphasizing its relevance in public health.
Moreover, successful transfection can lead to the expression of reporter genes, providing a clear indicator of whether the nucleic acids have been effectively introduced into the target cells. Thus, the ability to measure this success is fundamental for both research and clinical practices.
Historical Context
Transfection techniques have a rich and evolving history that mirrors advancements in genetics. The term itself dates back to the 1960s when it was first coined in the context of introducing foreign DNA into cells. In those early days, the process was often rudimentary and largely experimental.
- 1960s - 1980s: Initially, methods such as calcium phosphate precipitation facilitated the inclusion of DNA into cells. This paved the way for more sophisticated techniques.
- 1990s: The introduction of liposomes marked a watershed moment. It represented a significant leap forward in facilitating the delivery of nucleic acids into cells effectively.
- 2000s and beyond: The rapid development of viral vectors and newer methodologies—like electroporation—continued to push the boundaries of transfection capabilities.
Today, this field is witnessing a surge of innovation with the emergence of technologies like CRISPR, which relies on efficient transfection approaches for genome editing. This historical context not only highlights the evolution of genetic manipulation techniques but also underscores the growing recognition of transfection's pivotal role in shaping modern biology and medicine.
Mechanisms of Transfection
Understanding the mechanisms of transfection is crucial for both researchers and practitioners in the field of genetics. This section aims to elucidate how transfection works and the various methods employed to facilitate this process. Appreciating these mechanisms allows for the optimization of gene delivery techniques that are fundamental in genetic research, therapeutics, and biotechnology.
Prolusion to Delivery Mechanisms
Delivery mechanisms serve as the backbone of transfection, allowing genetic material to penetrate cellular barriers effectively. The choice of delivery method can significantly impact not only the efficiency of gene transfer but also the cell type being targeted. Each mechanism has its own set of advantages and challenges that researchers must consider when devising their experimental setups.
These mechanisms can vary widely; they can be as gentle as liposomes encapsulating DNA or as forceful as electroporation, which uses electrical pulses to create transient pores in the cell membrane. The right choice could mean the difference between a successful experiment and a dead end.
Types of Transfection Methods
Delving deeper into the specifics of transfection methods, we identify three primary categories: chemical, physical, and biological methods. Each plays a unique role in the overall transfection process, and understanding their characteristics is essential for informed choices.
Chemical Methods
Chemical methods involve using various reagents or chemicals to facilitate the introduction of nucleic acids into cells. One of the most common techniques here is lipofection, where liposomes encapsulate and deliver DNA into cells. The key characteristic of chemical methods lies in their versatility and user-friendliness. They can be utilized on different cell types, making them a popular choice for many laboratories.
- Unique Features: They often involve minimal equipment needs, making them highly accessible.
- Advantages: Cost-effective, lower skill requirement, and adaptable for various research scenarios.
- Disadvantages: Some chemical methods can lead to cytotoxicity or variable transfection efficiency across different cell types, requiring careful optimization and validation.
Physical Methods
Physical methods utilize mechanical or physical force to enhance gene transfer. Techniques such as electroporation and microinjection fall into this category. The standout aspect of physical methods is their efficacy, especially in hard-to-transfect cell types.
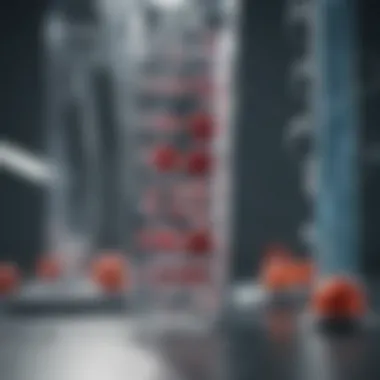
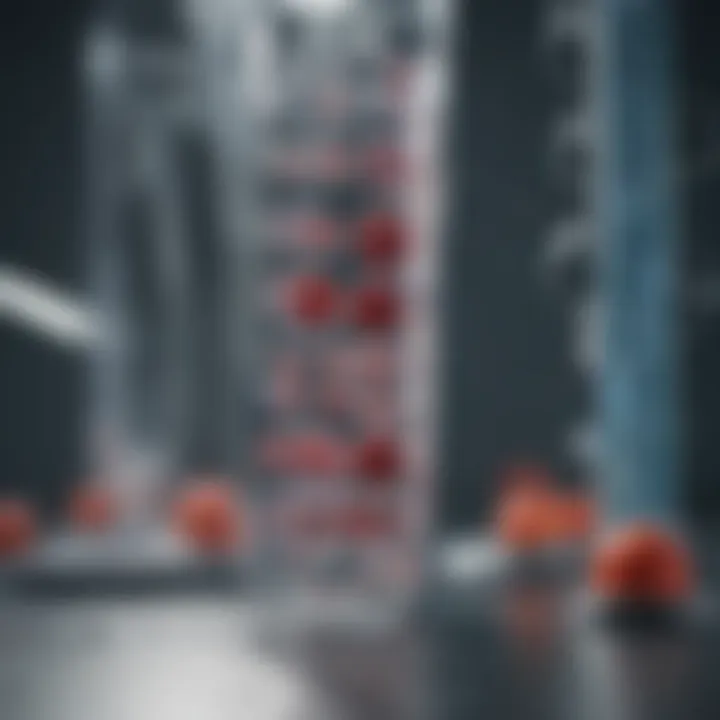
- Unique Features: They can achieve higher levels of transfection when other methods falter.
- Advantages: High efficiency and the ability to deliver larger DNA constructs.
- Disadvantages: They often require specialized equipment and could damage cells if not properly calibrated.
Biological Methods
Biological methods leverage naturally occurring biological processes, primarily through viral vectors. These methods are characterized by their specificity and efficiency in delivering genetic material into host cells.
- Unique Features: Utilization of viruses allows for targeted delivery and stable integration of genetic material into the host genome.
- Advantages: High levels of expression and the capability to transduce non-dividing cells.
- Disadvantages: There are concerns about safety, ethical implications, and potential immune responses to viral components, which warrant thorough consideration.
"In the field of genetics, no single approach fits all. Each transfection method must be tailored to the specific requirements of the given study or application."
By appreciating these mechanisms and their respective nuances, researchers can make informed decisions to enhance the success of their transfection endeavors. The exploration of these methods highlights the breadth of possibilities available in genetic manipulation and underscores the importance of method selection in achieving desirable outcomes.
Chemical Transfection Techniques
Chemical transfection techniques play a pivotal role in the landscape of genetic engineering, offering a means to introduce foreign nucleic acids into cells. This method is particularly valuable for research and therapeutic purposes. Chemical methods are not only effective but also often more economical when compared to other approaches. Understanding these techniques can shed light on their applications in gene therapy, vaccine development, and cancer research.
Lipofection
Lipofection is a popular method of chemical transfection that utilizes liposomes—small vesicles made of lipid bilayers—to deliver genetic material into cells. This method hinges on the principle that these liposomes can merge with cellular membranes, allowing the payload of DNA or RNA to enter the cytoplasm. One of the key benefits of lipofection is its versatility; it can be used across various cell types, ranging from adherent to suspension cells.
The mechanism is relatively straightforward, and it boils down to a few essential steps. First, the nucleic acids are mixed with liposomes to form lipoplexes. These complexes are then added to the target cells. Importantly, the efficiency of lipofection can be influenced by several factors, such as the lipid composition, the nucleic acid cargo, and even the cell type being transfected.
When it comes to practical considerations, lipofection allows users to fine-tune their transfection conditions. For instance, one might adjust the ratio of lipids to nucleic acid, which could enhance transfection efficiency without incurring cellular toxicity. This method has a sterling record in not only laboratory but also applied settings, having been successfully utilized to deliver plasmids for gene expression in therapeutic explorations.
Polymer-Based Transfection
Another noteworthy technique in the realm of chemical transfection is polymer-based transfection, which employs cationic polymers to facilitate the uptake of genetic material. These synthetic polymers, such as Polyethylenimine (PEI), can form electrostatic complexes with negatively charged nucleic acids. This interaction fosters cellular uptake of the genetic material, thanks to the positive charge of these polymers attracting them to the cell membrane.
Polymer-based methods have distinct advantages. For one, they offer a high payload capacity, allowing larger fragments of DNA or RNA to be transferred into cells. This attribute makes them particularly appealing for applications requiring substantial genomic alterations or for delivering multiple payloads simultaneously. Moreover, these methods are often adjustable according to the specifics of the target cells, enhancing their utility.
Nevertheless, one has to weigh potential challenges against the benefits. For instance, the cytotoxicity of certain polymers may be a concern, demanding careful calculation of the optimal polymer concentration for effective gene delivery without adversely affecting cell viability.
In sum, chemical transfection techniques—whether through lipofection or polymer-based methods—stand to significantly enrich the toolbox available to researchers and practitioners in genetics. With continued advancements and refinements, these methods are likely to bolster the development of groundbreaking applications in gene therapy and beyond.
Physical Transfection Approaches
Physical transfection methods play a crucial role in genetics, particularly in their ability to introduce nucleic acids into cells using non-chemical means. Unlike chemical approaches that rely on reagents to facilitate the uptake of genetic material, physical methods allow for more direct manipulation of the cellular environment. This direct method is imperative for certain challenging cell types and applications where chemical methods may fall short.
These methods can be particularly beneficial in scenarios requiring high delivery efficiency or targeting specific cell types that are otherwise difficult to transfect. The two primary forms discussed here—electroporation and microinjection—have unique advantages and considerations, making them essential tools in both research and therapeutic development. Their precision and effectiveness can lead to significant advancements in gene therapy, vaccine development, and other areas of biotechnology.
Electroporation
Electroporation is a physical transfection technique that uses electrical fields to create temporary pores in cell membranes, allowing for the entry of nucleic acids. This method has garnered much attention for its versatility, being suitable for a variety of cell types, including those that are typically resistant to transfection, such as primary cells and stem cells.
Key Advantages of Electroporation:
- High Efficiency: Electroporation often achieves significantly higher transfection rates compared to standard methods, especially in hard-to-transfect cell types.
- Minimal Cellular Damage: When performed correctly, this method results in less damage to cells compared to some viral or chemical methods.
- Scalability: The process can be scaled up for larger systems, which is advantageous in industrial applications, such as vaccine production.
However, the technique does come with its challenges. The parameters of electroporation—such as voltage, pulse duration, and frequency—must be meticulously optimized. Incorrect settings can lead to cell lysis or inefficient transfection. Researchers must also be cautious regarding the cell recovery period post-treatment, ensuring they allow sufficient time for cells to stabilize before analysis or downstream applications.
Microinjection Techniques
Microinjection is another physical transfection approach, where genetic material is directly injected into the cytoplasm or nucleus of a target cell using a fine needle. This technique is particularly valuable for applications requiring precision, such as generating transgenic organisms or studying gene function in single cells.
Notable Features of Microinjection:
- Precision: Allows for personalized delivery of genetic material to specific cells, which can be particularly useful in developmental biology.
- Direct Delivery: Bypasses many barriers associated with other methods, ensuring that targets receive the exact dosage intended.
- Immediate Results: The effects of gene manipulation can often be observed quickly after microinjection, making it an appealing method for researchers.
On the downside, microinjection can be quite labor-intensive, requiring skilled personnel and specialized equipment. The necessity to handle individual cells can bottleneck throughput and increase the complexity of experiments. Moreover, the invasiveness of this method may lead to unintended cellular responses, complicating interpretation of results.
In summary, both electroporation and microinjection serve as powerful physical transfection tools, each with its own strengths and limitations. As research in genetics continues to evolve, refining these methodologies will be essential for unlocking new potentials in various applications, particularly in gene therapy and the development of innovative vaccines.
Biological Transfection Methods
Biological transfection methods are pivotal in the field of genetic research and therapy. They utilize biological agents, such as viruses, or modified biological entities to deliver genetic material into target cells. This approach is particularly significant because it often mimics natural infection processes, allowing for efficient and precise transfection. Among the advantages, biological methods can achieve higher transfection efficiencies compared to synthetic approaches, particularly in cell types that are typically hard to transfect.
Viral Vectors
Viral vectors play a prominent role in biological transfection methods. They are a well-established mechanism for delivering genetic material, often used in gene therapy. The versatility of viral vectors comes from their ability to integrate genetic material directly into host cells.
The key characteristic of viral vectors is their inherent ability to infect host cells efficiently. This makes them quite beneficial for targeting specific cells. For instance, adenoviruses and lentiviruses are widely used due to their capacity to infect dividing and non-dividing cells effectively.
However, there are considerations to keep in mind. Some viral vectors can elicit an immune response, which may limit their application in clinical settings. Additionally, the potential for insertional mutagenesis, where the integration of viral DNA disrupts host genes, presents risks that must be mitigated through careful design and testing.
Cutting-Edge Techniques
CRISPR-Cas Systems
One of the cutting-edge techniques in biological transfection is the CRISPR-Cas system. This technology has revolutionized the genetic engineering landscape by enabling precise gene editing. The unique feature of CRISPR-Cas is its programmability; researchers can design guide RNAs to direct the system to specific DNA sequences. This has made it a popular choice for both research and therapeutic applications.
Besides its precision, CRISPR-Cas systems allow for multiple edits at once, which is a clear advantage when multiple genetic modifications are necessary. However, these systems are not without drawbacks. Off-target effects, although decreasing with advancements, can still pose challenges in generating desired genetic modifications without unintended consequences.
Nanoparticle Technology
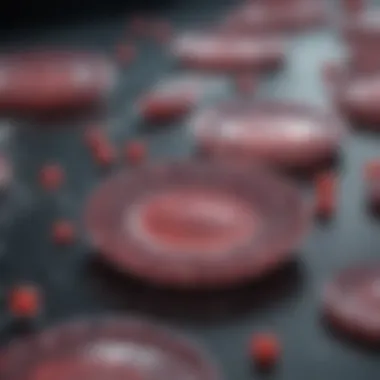
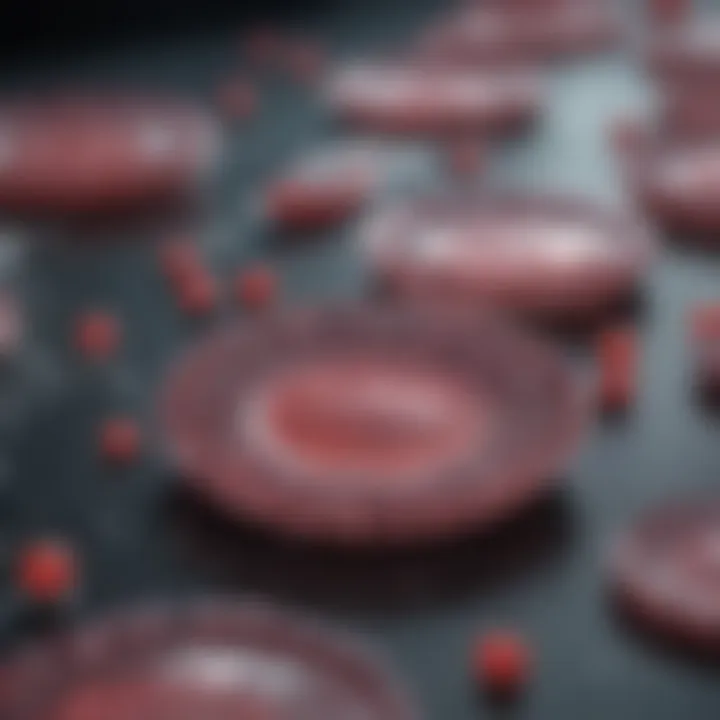
Nanoparticle technology also represents a noteworthy advancement in biological transfection methodologies. This technique involves the use of nanoparticles, often made from natural or synthetic materials, to encapsulate genetic material. The primary merit here is the protection and delivery of nucleic acids directly into the target cells without the issues related to immune responses often seen with viral vectors.
A distinct feature of nanoparticles is their ability to be modified for enhanced targeting, which allows for specific delivery to desired cells or tissues. They can carry larger DNA fragments or RNA, making them very versatile. Even though advantageous, challenges still persist, such as the complexity of scaling production and ensuring stable delivery without degradation in biological systems.
Biological transfection methods, from viral vectors to cutting-edge techniques like CRISPR and nanoparticles, continuously evolve, reflecting the intricate dance of innovation and discovery in genetics.
In summary, biological transfection methods offer powerful tools in genetics, each with unique characteristics and considerations. The right choice often hinges on the specific goals of the research and the characteristics of the target cells.
Applications of Transfection
Transfection stands as a cornerstone technique in modern genetics, serving a multitude of functions across various fields. Its importance springs from the potential to deliberately introduce nucleic acids into cells. This ability opens doors to numerous applications in research, biotechnology, and clinical settings. Understanding transfection involves not only the technical aspects but also the broader implications for advancements in medicine and science.
Transfection in Gene Therapy
Gene therapy aims to correct defective genes or to replace them with healthy ones to treat diseases. Transfection plays a vital role in this process, as researchers often need to deliver therapeutic genes into a patient’s cells. Techniques such as lipid-mediated transfection and viral vectors have become the backbone of this methodology.
For instance, consider the case of cystic fibrosis, a genetic disorder caused by mutations in the CFTR gene. In this context, transfection enables the delivery of a correct copy of the CFTR gene into the affected epithelial cells in the lungs. This corrective strategy is a significant leap toward managing the disease, potentially transforming patients' lives.
The success of gene therapy depends heavily on the efficiency of transfection methods. This includes not just the selection of the best technique but also fine-tuning it according to the specific cell type. Some cells are more amenable to transfection than others, making personalized approaches necessary.
Role in Vaccine Development
Transfection's influence extends to vaccine development, especially highlighted by the pandemic response seen with mRNA vaccines for COVID-19. Here, transfection facilitates the introduction of mRNA that encodes for specific antigens, prompting an immune response. The ease and speed of developing such vaccines have redefined our approach to infectious diseases.
Specifically, the way mRNA vaccines leverage transfection means they can be produced quicker than traditional vaccine methods, allowing for a more rapid response to emerging pathogens. This game-changing approach could set the stage for vaccines against a range of diseases, all thanks to the fundamental principles of transfection.
"Transfection techniques simplify the rapid development of vaccines, turning theoretical frameworks into actionable solutions."
Use in Cancer Research
Cancer research benefits significantly from transfection techniques, enabling studies on gene function and cancer pathways. It allows scientists to introduce oncogenes or tumor-suppressor genes to assess their role in cancer progression. For instance, by transfecting cells with specific oncogenes, researchers can identify alterations in cellular behavior that indicate cancerous traits.
Moreover, transfection methods are crucial in developing targeted therapies. By manipulating genes involved in cancer growth, researchers are gaining insights into tailored treatment options that are more efficient and less harmful than standard chemotherapy.
The intricate dance between transfection, genetic understanding, and cancer treatment represents a promising frontier in oncology. Finding ways to enhance transfection efficiency in cancer cells could lead to monumental breakthroughs in personalized medicine, leading to better patient outcomes.
In summary, applications of transfection permeate various domains in genetics. The implications for gene therapy, vaccine development, and cancer research are profound, signaling how this technique helps guide the future of biotechnology and medicine.
Monitoring and Analyzing Transfection Efficiency
Understanding how effective transfection is plays a crucial role in genetics research and therapeutic applications. Monitoring and analyzing transfection efficiency helps researchers assess whether their methods are yielding the desired outcomes, which can ultimately guide them in refining or adapting their techniques. It can influence big-picture decisions, such as whether to proceed with a particular gene therapy approach or utilize specific transfection reagents.
Several factors come into the play when measuring efficiency, including the choice of method, the type of cells being used, and even the specific genetic material being delivered. Each of these aspects can skew results, making it vital to apply reliable techniques for analysis. This step is not merely a bureaucratic measure; it establishes the foundation for further experimentation, ensuring that valuable time and resources are not wasted on ineffective approaches. The ramifications of these analyses extend beyond just immediate projects; they can inform practices across the field of genetic research.
Techniques for Efficiency Assessment
In analyzing the effectiveness of transfection, two primary techniques are often utilized: Fluorescent markers and Quantitative PCR. Each of these methods holds its own unique merits and considerations that merit exploration.
Fluorescent Markers
Fluorescent markers are vital tools in visualizing transfected cells and assessing how many successfully integrated the intended genetic material. The bright luminescence emitted by these markers allows researchers to observe biological processes in real-time. One key characteristic of fluorescent markers is their ability to provide immediate feedback. This offers researchers a glimpse into the transfection process as it unfolds, highlighting successful integrations almost as soon as they occur.
Researchers often favor fluorescent markers not only for their ease of use but also for their versatility. They can be paired with various cell types and used in multiple experimental contexts. However, a unique feature to consider is the potential for cellular artifacts. Sometimes, cells may fluoresce due to background noise or nonspecific staining, which can lead to inaccurate conclusions about efficiency. Despite this drawback, the advantages they present, such as real-time monitoring and visual clarity, often outweigh the downsides.
Quantitative PCR
On the other hand, Quantitative PCR (qPCR) serves as a rigorous analytical method that quantifies the amount of specific DNA or RNA in a sample, providing a more nuanced understanding of transfection efficiency. One of its key strengths lies in its precision. By amplifying the target genetic material, researchers can determine the exact quantity present in a cell, which in turn reveals much about the efficacy of the transfection process. This method is particularly beneficial for understanding variations across different cell lines, allowing researchers to tailor their approaches accordingly.
The unique feature of qPCR is its ability to discern subtle differences in expression levels, which can be crucial for fine-tuning gene therapy strategies. However, it's important to acknowledge that qPCR demands more resources and expertise than fluorescent markers do. The complexity of the protocol may not be suitable for all situations, especially in initial exploratory studies. Yet, when a precise measurement is needed, qPCR remains indispensable for monitoring transfection success.
"Accurate assessment of transfection efficiency can dramatically shape the trajectory of genetic research, laying groundwork for future success."
End
Both fluorescent markers and Quantitative PCR are essential techniques for monitoring transfection efficiency, each contributing uniquely to our understanding. As researchers navigate through the complexities of transfections, the right choice of methodology can meaningfully impact outcomes and push the boundaries of genetic research and therapy.
Challenges in Transfection
Transfection is a powerful method in genetics, but it doesn’t come without its hurdles. Understanding the challenges is essential, especially considering the implications they carry for both research and therapeutic applications. When diving into the intricacies of transfection, one must pay close attention to specific factors affecting cell type interactions and limitations in delivery methodologies. This section will elucidate these challenges, offering insights that cater to the needs of students, researchers, and professionals interested in this field.
Cell Type-Specific Responses
Not all cells are created equal; this adage rings particularly true in the realm of transfection. Different cell types exhibit varying degrees of responsiveness to transfection methods. For example, while some cells, like HEK293 or HeLa cells, are notoriously easy to transfect, others, such as primary cells or stem cells, can be fairly resistant. This variation can be attributed to several factors:
- Cell membrane composition: Differences in lipid and protein profiles can greatly affect how easily a cell can take up new genetic material.
- Endosomal processing: Once genetic material is inside a cell, it often travels through endosomes. Some cells may have faster endosomal degradation, which could hinder successful expression.
- Cell cycle stage: The timing at which transfection occurs can make or break the process. For instance, cells in mitosis may be more receptive to certain transfection reagents.
These cell-type-specific responses need to be meticulously considered when selecting a transfection approach. A trial-and-error process may end up wasting precious resources, making it vital to conduct preliminary screenings that pinpoint the right methods for specific cell lines.
Delivery Limitations
Delivery restrictions present another hurdle in the world of transfection. The methodologies available, whether chemical, physical, or biological, all have their own unique challenges. Some key limitations include:
- Efficiency vs. toxicity: Many of the more effective transfection methods come with a downside. While electroporation might yield high transfection rates, it can also induce significant cellular stress, which can lead to cell death. Striking a balance here is crucial.
- Size and type of genetic material: Larger plasmids or larger RNA molecules might not easily fit through the cellular gate—think of it like trying to fit an oversized suitcase through a tiny door. Some methods can't accommodate larger constructs.
- Stability of the delivered material: Once genetic material is inside the cell, its stability becomes a prime concern. If it's too fragile, it may not survive long enough to achieve the desired effect.
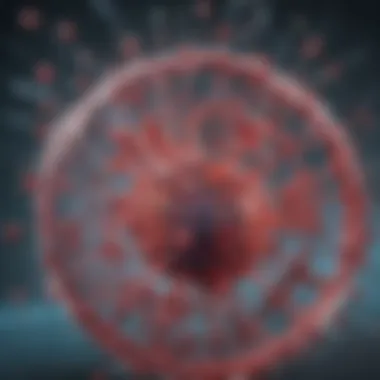
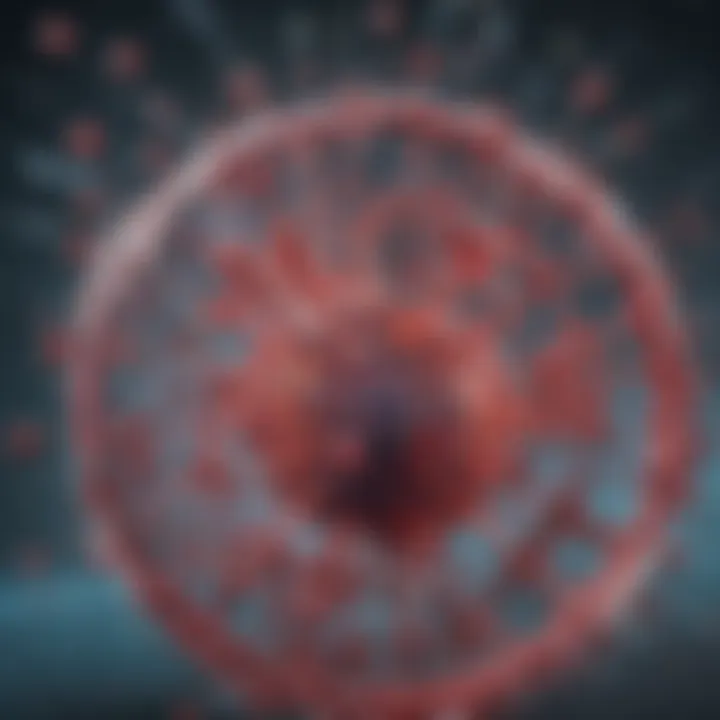
In light of these challenges, researchers are urged to explore novel avenues for overcoming the limitations inherent to outdated and conventional techniques. > "Innovative approaches are the linchpin for progressing in gene therapy and cutting-edge research,” highlights a study on evolving transfection methods.
Navigating these challenges equips one with a deeper understanding of transfection’s complexities. A comprehensive grasp of the various responses and limitations enables researchers to tailor their approach more effectively. As transfection continues to evolve, so does the potential for groundbreaking applications in genetics and biomedical research.
Future Perspectives in Transfection Genetics
Transfection technology, a cornerstone in genetic research and therapy, is rapidly evolving, with numerous exciting pathways emerging. As scientists grapple with the challenges of effectively delivering genetic material into cells, the future of transfection promises innovative solutions that can enhance the precision and efficacy of genetic engineering. This section highlights some key areas that hold immense potential for advancements in the field.
Emerging Technologies
The landscape of transfection methods is shifting with the advent of novel technologies. Here are some noteworthy innovations:
- Nanoparticle Systems: These tiny particles can efficiently carry genetic material into cells. By modifying their surface properties, researchers can control how they interact with target cells, minimizing potential side effects while maximizing delivery success.
- CRISPR Advances: While CRISPR is best known for gene editing, its delivery mechanisms, including its integration with viral vectors and nanoparticles, are being fine-tuned to allow for more targeted and effective transfection.
- RNA Delivery Methods: As the use of RNA-based therapeutics grows, new techniques are being developed specifically for delivering RNA molecules, including modified oligonucleotides that enhance stability and cellular uptake.
With these technologies, we may see transfection efficiency improve significantly, paving the way for breakthroughs in various applications, including new treatments for genetic disorders.
Transfection in Personalized Medicine
Personalized medicine aims to tailor treatments to individual patients based on their genetic makeup. Transfection plays a critical role in this paradigm shift. Specifically, here’s how transfection technologies can enhance personalized approaches:
- Customized Gene Therapies: By employing patient-specific cells for therapies, researchers can use transfection to introduce corrective genes, providing a more customized solution to genetic diseases.
- Tailored Vaccine Platforms: With transfection, vaccines can be designed to match specific viral strains or mutations. This adaptability allows for rapid development in response to emerging health threats.
- Biomarker Discovery: Transfection can aid in the identification of biomarkers by facilitating the introduction of genes that can illuminate disease pathways in patient-derived cells, paving the way for targeted interventions.
In exploring these avenues, it is crucial to remain cognizant of the ethical implications and regulatory challenges. The balance of innovative research with careful consideration of safety and efficacy remains paramount.
"The future of transfection in genetics holds the promise of not just new techniques, but transformative impacts on how we diagnose and treat diseases, tailoring therapies in unprecedented ways."
In summary, the horizons of transfection research are expanding, driven by advances in technology and the need for personalized approaches in medicine. As we look forward, the intersection of these innovative tools with genetic research may redefine our understanding and treatment of various conditions, linking molecular biology marvels with real-world applications.
Ethical Considerations in Transfection Research
The realm of transfection in genetics opens a Pandora's box of ethical dilemmas and considerations. The advancements in this field, while promising, also bring about crucial discussions regarding safety, regulation, and the implications of genetic manipulation. The importance of addressing these ethical considerations cannot be overstated, as they shape public perception and influence the direction of future research and applications.
Regulatory Framework
In the landscape of genetic research, the regulatory framework plays a pivotal role in guiding transfection practices. Different countries have their distinct laws and guidelines that dictate how transfection can be performed, especially when it comes to human subjects. For example, in the United States, the Food and Drug Administration (FDA) oversees many aspects of genetic research, ensuring that any transfection methodologies meet safety standards before they can be trialed.
Moreover, guidelines from organizations such as the National Institutes of Health (NIH) help in managing the ethical implications by requiring comprehensive risk assessments. These guidelines ensure that transparency is maintained, allowing both researchers and the public to be informed. Consequently, it becomes a balancing act to foster innovation while keeping safety at the forefront.
Public Concerns and Misunderstandings
The public perception of transfection research is often mixed, fueled by misunderstandings about what genetic manipulation entails. Misunderstandings can lead to panic or resistance, underlining the need for clear communication from researchers. Many people still equate genetic editing with the fearsome specter of designer babies or unintended consequences that could arise in the ecosystem.
To address these concerns, researchers need to actively engage with the community. Public forums, transparent research disclosures, and educational outreach can help clarify the reality of transfection techniques and their benefits. It's crucial to highlight that responsible research underscores the notion of informed consent, ensuring participants understand the scope and risks involved.
“Ethics is knowing the difference between what you have a right to do and what is right to do.” — Potter Stewart
Overall, ethical considerations in transfection are not just a regulatory obligation but a moral compass guiding researchers toward responsible innovation. The synergy between regulatory frameworks and community engagement can help demystify transfection and foster a positive environment for future advancements in genetics.
Finale
In wrapping up the discussions presented in this article, it becomes clear that transfection plays a vital role in the landscape of genetics and biotechnology. This process, emphasizing the introduction of nucleic acids into cells, provides numerous benefits that extend beyond basic research. The capacity to modify genetic material opens up pathways for innovative therapies, particularly in the realm of gene therapy, vaccine development, and cancer research.
One of the crucial elements highlighted is how diverse delivery mechanisms enhance the precision of transfection. Methods like lipofection, electroporation, and the use of viral vectors are not just technical jargon but are essential tools that have propelled the field forward. With increasing advancements in technology, the efficiency and specificity of these methods continue to improve, reducing the hurdles previously faced by researchers.
Moreover, the ethical considerations surrounding transfection cannot be overstated. As much promise as the technology holds, it also raises questions about safety, consent, and the long-term implications of genetic modifications. Regulatory frameworks are being adapted continuously to keep pace with rapid advancements, ensuring that the benefits of transfection do not come at an unreasonable risk to human health or biodiversity.
Despite the significant gains made, challenges remain. Cell-type-specific responses and limitations in delivery mechanisms are still obstacles for scientists. The focus on these challenges must persist so that future iterations of transfection techniques can achieve even greater success.
Ultimately, this article does more than discuss transfection in a vacuum. It lays out a roadmap for understanding not just the current state of the field, but also its trajectory moving forward—where it intersects with emerging technologies and personalized medicine, which promise to further reshape healthcare and research landscapes.
The potential applications of transfection techniques are as boundless as the human imagination, thus emphasizing the necessity for continued exploration and ethical oversight.
Summary of Key Findings
- Transfection is an essential mechanism in modern genetics, allowing for the manipulation of genetic material.
- Various delivery methods, including chemical, physical, and biological techniques, play a pivotal role in maximizing transfection efficiency.
- The field is impeded by challenges such as cell-specific responses and regulatory concerns, necessitating ongoing research and ethical consideration.
- Advances in transfection techniques are crucial for breakthroughs in gene therapy, vaccine development, and cancer research.
Implications for Future Research
The future of transfection research is ripe with possibilities.
- Innovation in Delivery Systems: The quest for more efficient and targeted delivery mechanisms is expected to drive forward new methodologies. Exploring nanoparticles and improving viral vectors may offer higher success rates and fewer side effects.
- Personalized Approach: With the rise of personalized medicine, the tailoring of transfection methods to specific patient needs could revolutionize treatment protocols for various diseases.
- Ethics and Regulations: As the field evolves, so too must the frameworks governing it. Addressing public concerns and ensuring the safe application of transfection technologies will play a fundamental role in their acceptance and use in clinical settings.
The horizon is expanding, and as we navigate through it, the integration of ethical principles with cutting-edge science will be necessary to ensure that transfection techniques serve humanity effectively.
Importance of References
The role of references extends beyond simply acknowledging past work; it helps contextualize current research within a broader framework. In the discussion of transfection mechanisms and methodologies, references play an essential part in illustrating how these techniques have evolved over time and how they are substantiated by empirical data. They demonstrate the progression from primitive methods to advanced approaches, reiterating the continuous innovation in the field.
Considerations for Compiling References
When compiling a reference list, adherence to specific guidelines is crucial. The academic community often employs particular styles, like APA or MLA, which dictate how sources should be formatted. Here are several key points to consider:
- Credibility of Sources: Always prioritize peer-reviewed articles, books authored by reputable scientists, and established journals like Nature and Cell.
- Diversity of Sources: Include a mix of recent studies and classic papers to show the foundational theories while acknowledging emerging trends in research. You may want to reference articles from encyclopedic sources like Britannica for foundational knowledge.
- Linking Ideas: Use references strategically to link various points throughout the article, allowing readers to see the connections between different concepts.
Building Trust with Your Audience
Given the growing skepticism about scientific claims, reinforcing your information with solid references creates a foundation of trust. Readers can feel confident that the insights shared are vetted and supported by established scientific discourse. The references in this article allow readers to delve deeper into specific methodologies and results, empowering them to explore topics like chemical methods in transfection or the application of CRISPR-Cas technology.
Moreover, footnotes and endnotes can also provide additional commentary or context, which enriches the reader's understanding without interrupting the article's flow.
"References not only substantiate a claim; they are the bridge between knowledge and exploration. They allow readers to embark on their investigative journeys alongside the authors."
Ultimately, a well-constructed reference section enhances the quality of the entire article. By guiding further inquiry, it strengthens the academic rigor of the writing and invites readers to actively engage with the material presented. In a field as dynamic as genetics, being able to trace findings back to their sources is invaluable and opens the door for future exploration.