Neutron Detection Equipment: Principles and Innovations
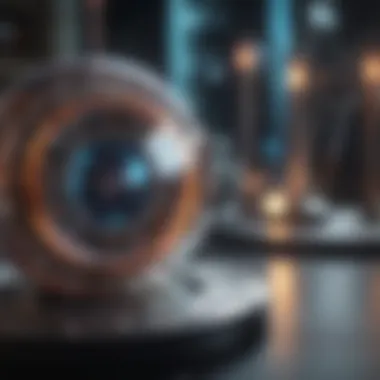
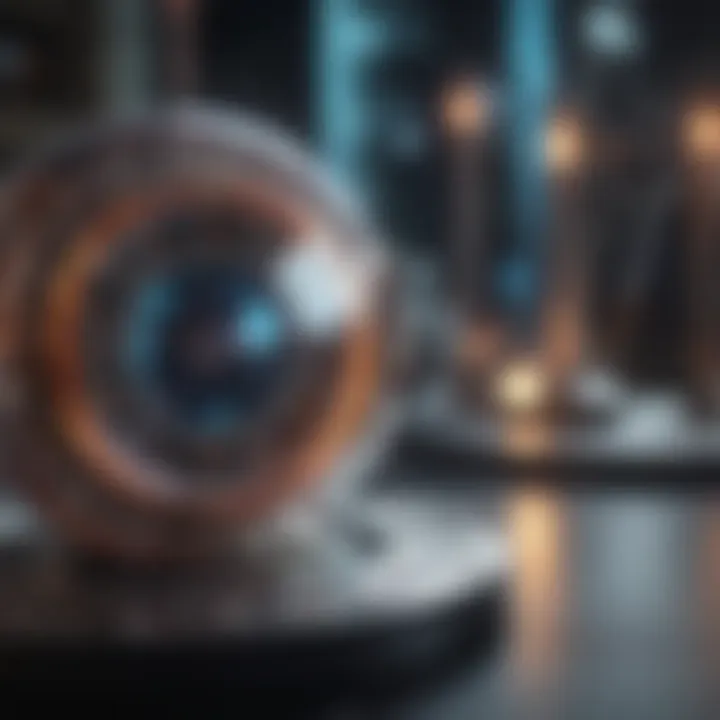
Intro
Neutron detection equipment plays a pivotal role in a wide range of scientific and industrial applications. The significance of neutrons, being neutral particles, often gets overlooked when compared to their charged counterparts—protons and electrons. However, these subatomic particles are crucial for nuclear reactions and have diverse applications from power generation to medical therapies.
In understanding neutron detection, it’s essential to grasp the fundamental principles that govern how these devices operate. The basic premise revolves around the interaction of neutrons with matter, leading to the generation of secondary particles that can be measured. With innovations over recent years, the equipment used for these processes has become increasingly sophisticated and accurate.
The scope of this article stretches from the foundational principles of neutron detection equipment to its varied applications. It also touches on the current landscape of technological advancements in this field while addressing the challenges faced by researchers and practitioners. Additionally, it looks ahead at future research directions that could shape the development of neutron detection methods, ensuring they remain relevant in an ever-evolving scientific context.
"Neutron detection is not merely a scientific curiosity; it is a cornerstone of safety in nuclear facilities and an essential tool in advancing research capabilities across many domains."
With this exploration, readers from academia, industry, and beyond will gain valuable insights into how these tools contribute to significant technological progress and safety measures in nuclear science.
Intro to Neutron Detection Equipment
The realm of neutron detection equipment is vital for a multitude of fields ranging from nuclear science to medicine. Neutrons, despite being neutrally charged, play a significant role in the behavior of atomic nuclei. This includes how they interact with various materials, making neutron detection essential for safe operations in environments where nuclear reactions and radiation occur. Understanding the principles behind neutron detection helps not only in harnessing energy but also in ensuring safety and regulating radioactive materials.
Understanding Neutrons
Neutrons are subatomic particles found in the nucleus of an atom, where they reside alongside protons. Unlike protons, neutrons have no electric charge, which is quite a curious statement of their nature. They, however, contribute to the atomic mass of an element dramatically. An example lies in helium-4, comprised of two protons and two neutrons—giving it a mass that is almost double than if it merely had protons alone. Neutrons undergo various interactions when colliding with other atomic particles, such as scattering or absorption, which serve as the foundational principles for neutron detection.
A critical aspect of understanding neutrons is recognizing how they can traverse materials without much resistance compared to charged particles. Therefore, materials used for neutron detection must capitalize on this unique property, leading to the development of specialized detectors.
Importance of Neutron Detection
Detecting neutrons is not just a theoretical exercise; it holds practical significance across various domains. Primarily, in the nuclear energy sector, neutron detection provides safety protocols and monitoring systems to maintain secure nuclear reactions within reactors. Moreover, it plays a vital role in nuclear safeguards, preventing unauthorized access or proliferation of nuclear materials. This focus on security cannot be understated as it relates to global safety.
In addition to the nuclear industry, neutron detection plays a pivotal part in medical imaging and treatments. For instance, neutron radiography allows clinicians to obtain images of objects that contain dense materials, spanning from anatomical structures in a patient's body to the integrity of mechanical parts in industrial applications. Similarly, in targeted cancer therapies, neutrons offer a unique way to destroy malignant cells while sparing surrounding tissues by exploiting the interaction of neutron radiation with certain isotopes used in treatment.
"The applications of neutron detection are as vast as they are critical, influencing both safety in nuclear operations and advancements in medical technologies."
Furthermore, environmental monitoring requires neutron detection to assess radiation levels, particularly in areas with a history of nuclear activity or accidents. This vigilance aids in ensuring public health safety and contributing to broader environmental protection initiatives.
In sum, the importance of neutron detection spans multiple disciplines, each leveraged by different aspects of neutron interactions. From safeguarding nuclear facilities to enhancing medical treatments and keeping an eye on our environment, comprehending the principles, contexts, and methodologies of neutron detection equipment is essential for anyone involved in these sectors.
Basic Principles of Neutron Detection
Understanding the basic principles of neutron detection is vital since it lays the groundwork for comprehending how these devices operate effectively in various settings. Neutrons, being neutral particles, interact with matter differently than charged particles like protons or electrons. This section delves into two fundamental aspects: neutron interaction mechanisms, and the detection thresholds and sensitivity of various detection systems.
Neutron Interaction Mechanisms
Neutrons interact with matter primarily through two mechanisms: elastic scattering and inelastic scattering. In elastic scattering, a neutron collides with a nucleus, resulting in a change of direction and energy of the neutron while the nucleus recoils. This type of interaction allows neutrons to transfer some energy to the nucleus, making it a key mechanism for energy loss in detection processes.
On the other hand, inelastic scattering occurs when neutrons collide with a nucleus and cause it to become excited. The excited nucleus may emit gamma rays or other particles during its transition back to a lower energy state. This ability to excite nuclei is what informs the function of detectors, turning a seemingly invisible interaction into measurable data.
Additionally, neutron capture emerges as another essential interaction mechanism. In this case, a neutron is absorbed by a nucleus, which may lead to the emission of gamma rays or other particles; this is particularly important for certain types of neutron detection devices.
Understanding these interaction mechanisms is crucial, as they not only determine the efficiency of the detectors but also influence safety and effectiveness in various applications such as reactor monitoring and radiation protection.
"In neutron detection, the interaction mechanisms are the very bones of the process, directly shaping what devices can achieve and how they're used in real-world scenarios."
Detection Thresholds and Sensitivity
Detection thresholds and sensitivity refer to the minimum levels at which a detector can register neutron interactions. It's not just about detecting a neutron when it passes by; it's about making sure that every interaction above a certain energy level is effectively accounted for.
The detection threshold varies significantly depending on the type of detector in use. For instance, gas-filled detectors typically have lower thresholds and can detect thermal neutrons. They rely on ionization processes, where neutrons ionize the gas, leading to detectable electric signals. Conversely, scintillation detectors are often more sensitive and can register higher energy neutrons due to their mechanism of converting neutron energy into flashes of light.
Sensitivity is further modulated by the material properties in the detectors. Semiconductor detectors, for example, use materials like silicon or germanium, which allow for very precise measurements at low energy levels. The finer the detector can tune into these interactions, the more reliable it becomes in various applications ranging from medical treatments to environmental safety measures.
To sum up, grasping the principles of neutron interaction and the associated detection thresholds is essential. This knowledge is critical for researchers and professionals aiming to enhance neutron detection technology and apply it effectively across various fields.
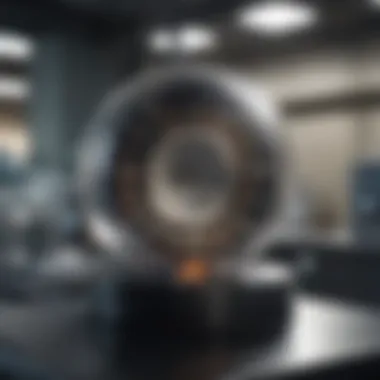
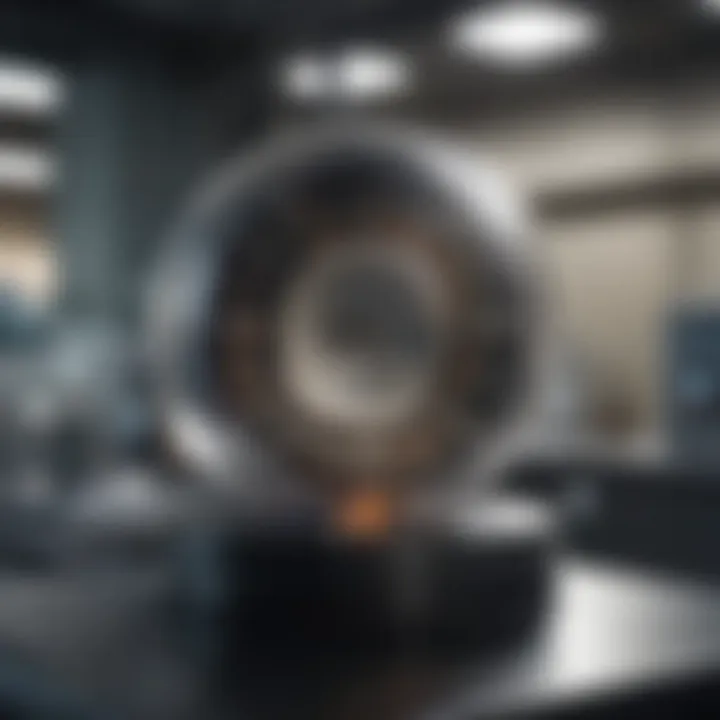
Types of Neutron Detectors
Neutron detectors play a crucial role in various fields, from nuclear safety to medical applications. Understanding the different types of detectors is fundamental for anyone venturing into neutron detection. In this section, we will dissect three main types of neutron detectors: gas-filled detectors, scintillation detectors, and semiconductor detectors. Each type has its own strengths and weaknesses, making them suitable for specific applications, which we aim to clarify in this deep dive.
Gas-Filled Detectors
Working Principles
Gas-filled detectors operate on a pretty straightforward principle—that is, the interaction of neutrons with gas molecules inside a sealed chamber. When a neutron collides with a nucleus of the gas—often helium or argon—it transfers enough energy to ionize it, producing charged particles. These charged particles then create an electric current as they move through the detector, which can be measured. This principle capitalizes on the property of ionization, making gas-filled detectors a popular choice thanks to their simplicity and reliability.
A unique feature of gas-filled detectors is their sensitivity to various neutron energies. They are particularly effective in mixed radiation fields, offering robust results in environments where other detectors might struggle. However, they can have limitations. For instance, they may not perform effectively in high-radiation environments due to potential saturation effects.
Advantages and Limitations
Gas-filled detectors boast several advantages. They're generally more affordable compared to other types, making them accessible for a variety of industrial applications. Their ability to measure the radiation dose makes them popular in nuclear safety settings. Furthermore, their lightweight design allows for portable applications, which can be critical in emergency response scenarios.
On the flip side, one significant limitation is their lower resolution compared to scintillation or semiconductor detectors. This means they may struggle with precise measurements in certain scenarios. Also, to maximize their effectiveness, gas-filled detectors often require careful calibration, adding an extra layer of complexity to their use.
Scintillation Detectors
Functionality Overview
Scintillation detectors utilize a different approach altogether. These detectors use materials that emit light when they absorb neutron energy. When neutrons collide with the atoms of a scintillation material—like sodium iodide or plastic scintillators—they produce photons, which can be counted to provide a measurement of neutron flux. The emitted light is then translated into an electrical signal, typically via photomultiplier tubes. This method allows scintillation detectors to boast high sensitivity and can detect a wide range of neutron energies.
A key characteristic of scintillation detectors is their ability to provide real-time measurements. This immediacy can be incredibly useful in fields where rapid decision-making is critical, such as in nuclear facilities or during environmental assessments.
Common Applications
Scintillation detectors find their place in various applications, for instance, in border security where detecting illegal smuggling of radioactive materials is paramount. They are also widespread in research applications, including neutron scattering experiments.
One of the unique benefits they offer is their lower energy threshold for detection, allowing for greater versatility across different safety scenarios. However, these detectors may require regular maintenance to keep them functioning optimally, which could be a drawback for some users.
Semiconductor Detectors
Materials Used
Semiconductor detectors primarily utilize silicon or germanium as their base materials. These materials are adept at converting absorbed neutron energy into electron-hole pairs, effectively enabling precise measurements of neutron interactions. The process typically involves the interaction of neutrons with the nuclei of the semiconductor material, producing a measurable signal.
The ability of semiconductor detectors to function at room temperature is a significant advantage, making them easier to deploy without elaborate cooling systems.
Performance Criteria
When discussing performance criteria, semiconductor detectors excel in aspects like energy resolution and timing accuracy. They can provide detailed spectral information, allowing researchers to differentiate between various radiation sources. This feature is particularly beneficial in laboratory settings where accurate data is critical.
However, semiconductor detectors can be more expensive to produce and maintain. They require a clean environment to avoid contamination, which sometimes limits their deployment in rugged conditions.
In summary, the choice among gas-filled, scintillation, and semiconductor detectors largely depends on the specific application, budget constraints, and the required sensitivity. Understanding these various types allows professionals in the field to select the right tools for their needs, thereby leveraging neutron detection technology effectively in diverse environments.
Applications of Neutron Detection
The significance of neutron detection lies in its diverse applications across various sectors, making it a critical field in science and technology. From ensuring safety in nuclear facilities to aiding in medical diagnostics, neutron detectors serve pivotal functions. These tools allow for precise measurements, effective monitoring, and innovative solutions to complex challenges. Their role cannot be understated when discussing advancements in nuclear technology, medicine, and environmental stewardship.
Nuclear Industry Applications
Nuclear Security
One of the most pressing uses of neutron detection is in the realm of nuclear security. In an age where global safety is paramount, safeguarding nuclear materials from theft or illicit use is critical. Neutron detectors can identify unusual nuclear signatures and provide real-time data to security personnel, helping to mitigate potential threats. The key characteristic of neutron detection in this context is its ability to discern between benign and suspicious activities, making it an essential tool for national and international security.
Furthermore, neutron detectors offer a unique feature: they can operate effectively in mixed radiation environments, providing valuable data even when other methods fall short. This adaptability is a significant advantage, allowing for enhanced monitoring of sensitive sites. However, the trade-off often lies in the high initial costs of implementing such systems, coupled with the need for specialized training to operate them effectively.
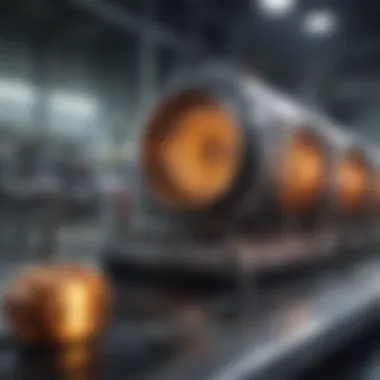
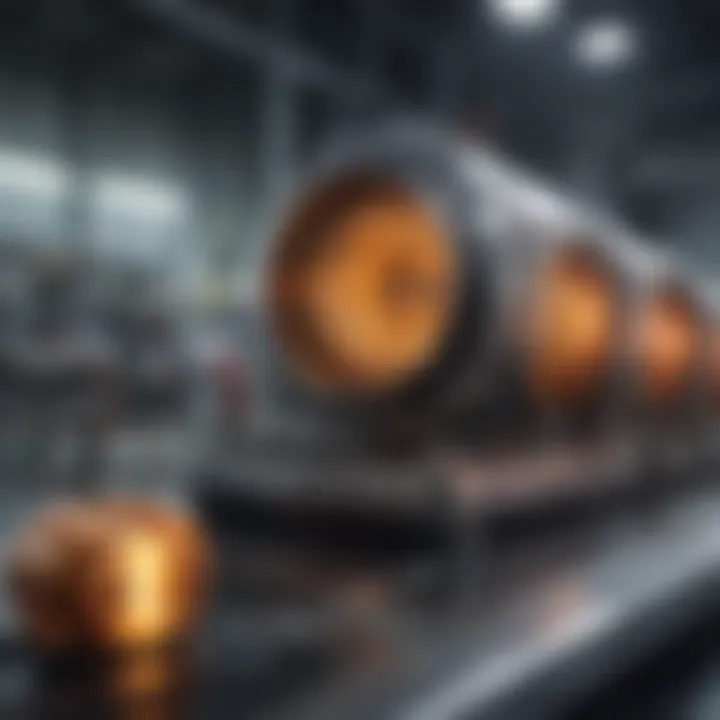
Reactor Monitoring
Another critical application is in reactor monitoring. In nuclear power generation, maintaining optimal safety and operational efficiency is non-negotiable. Neutron detectors provide insights into the reactor's nuclear reactions, enabling the precise balancing of fuel and control rods. The primary advantage of utilizing neutron detection in this area is the immediate feedback it provides, helping operators swiftly adjust parameters to prevent any unsafe conditions.
The unique feature here is the capability to detect neutron flux, which is instrumental in sustaining continuous power generation while ensuring safety protocols. Nevertheless, like the aforementioned application, the technology for reactor monitoring can be expensive and requires rigorous upkeep, posing a barrier for smaller facilities trying to enhance their operational safety measures.
Medical Imaging and Treatment
Neutron Radiography
In medical applications, neutron radiography emerges as a powerful imaging technique. By employing neutrons, this method allows for high-contrast imaging of soft tissues and other biological materials. The key characteristic of neutron radiography is its ability to visualize structures that traditional X-ray methods might overlook, particularly in examining complex organ systems. This makes it particularly valuable for diagnosing conditions in oncology and other fields.
The unique aspect of this application is that neutrons interact differently with various elements, enabling clearer images of hydrogen-rich tissues. While this technology is innovative, it does have its drawbacks; neutron radiography requires specialized facilities and can introduce a different type of exposure to patients, which necessitates careful consideration by healthcare providers.
Targeted Cancer Therapies
Another fascinating application is in targeted cancer therapies. Neutron beams can be harnessed for specific treatments, precisely targeting malignant cells while sparing surrounding healthy tissue. This selectivity is a powerful reason why neutron therapy is gaining traction. The notable characteristic here is that it utilizes neutron capture to induce radiochemical reactions that can destroy cancerous cells.
The benefit of targeted cancer therapies is evident; they can lead to improved treatment outcomes and potentially lower side effects compared to traditional radiation therapies. However, challenges remain, such as the accessibility of neutron therapy facilities and the ongoing need for research to refine treatment protocols and understand the biological implications fully.
Environmental Monitoring
Radiation Protection
Environmental applications of neutron detection are critically important, particularly in radiation protection. The ability to monitor radiation levels in various settings—from nuclear power plants to hospitals—helps minimize exposure risks to workers and the general public. The key characteristic of neutron detectors in this domain is their sensitivity to mixed radiation sources, allowing for comprehensive risk assessments.
The unique feature of neutron detection for radiation protection is its capability to provide real-time data, which is essential in emergencies or environmental assessments post-incident. Nonetheless, the reliance on such technology can be limited by the operational costs and complexities of integration into existing safety systems.
Nuclear Waste Management
Lastly, neutron detection plays a crucial role in nuclear waste management. Effectively managing and monitoring waste is paramount in preventing contamination and ensuring public safety. Neutron detectors can assess the composition of waste materials, aiding in the classification and safe handling of potentially hazardous substances. The significant characteristic here is the ability to detect fissile materials, thus facilitating better waste management practices.
The advantage lies in the technology’s non-invasive nature, which ensures that the integrity of waste samples is preserved while assessing their properties. However, issues like inconsistent operational capabilities in field conditions and the costs associated with maintaining such systems can limit their widespread adoption in this necessary area.
Technological Innovations in Neutron Detection
In the ever-evolving landscape of nuclear science and safety, technological innovations in neutron detection serve as a cornerstone. Not only do these advancements improve the accuracy of neutron detection, but they also broaden the scope of applications across various fields. As the world ramps up its focus on nuclear energy, medical imaging, and environmental safety, the role of cutting-edge detection technologies becomes increasingly prominent. This section explores key innovations, emphasizing materials and the integration of digital systems that mark significant strides in neutron detection.
Advancements in Detector Materials
One of the most exciting frontiers in neutron detection rests in the improvement of detector materials. Traditional materials might have served well in earlier years, yet modern demands require ever-greater efficiency and sensitivity. Recent developments in boron compounds have sparked interest due to their capacity to capture neutrons effectively. For example, the use of lithium-doped glass has emerged as a game-changer, offering a lightweight and durable alternative to heavier detectors.
- Detection Efficiency: New materials like lithium-6 or boron-10 are designed to maximize the likelihood of interactions with incoming neutrons, leading to better detection rates.
- Size and Weight: Innovations focus on producing lighter, more compact devices without sacrificing performance. These advancements facilitate the use of neutron detectors in portable applications, such as field surveys and emergency response scenarios.
- Cost-Effectiveness: Research into synthesized materials can potentially lower production costs. This makes neutron detection technology more accessible to laboratories and organizations that might otherwise shy away due to high expenses.
As the demand for precise neutron detection continues to surge, investments in material science point towards a promising future, unlocking new opportunities in multiple domains of application.
Integration with Digital Systems
With the rapid integration of digital technologies into various sectors, neutron detection systems are not left behind. The merger of traditional detection methods with digital advancements enhances both efficiency and analysis capabilities. The benefits of this integration are manifold.
- Real-Time Data Analysis: Digital systems can process the signals from detectors almost instantaneously. This capability allows researchers to view results in real time, paving the way for quicker decision-making and response protocols.
- Data Storage and Retrieval: The use of digital platforms facilitates efficient data management. Researchers can now archive vast amounts of detection data, making it easier to revisit and analyze previous experiments or readings at a later date.
- Remote Monitoring: Modern detection setups often feature remote accessibility, allowing professionals to monitor neutron activity from a distance. This development is especially significant in hazardous environments where physical presence may be risky.
Integration of digital systems not only streamlines the detection process but also enhances safety protocols, making them vital in high-stakes scenarios.
The intersection of cutting-edge materials and digital technologies is not merely an upgrade; it's a redefinition of how we approach neutron detection in our daily scientific practices.
Challenges in Neutron Detection
Neutron detection, while fundamental to numerous fields, isn't without its hurdles. Understanding these challenges is crucial for advancing both technology and methodology in neutron detection. Two predominant challenges persist in this realm: background radiation interference and the cost and accessibility of technology. Examining these obstacles underlines the need for innovation and better strategies to enhance the efficacy of neutron detection systems.
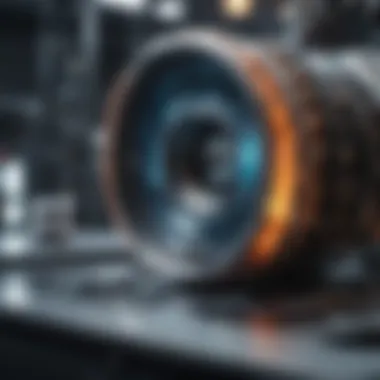
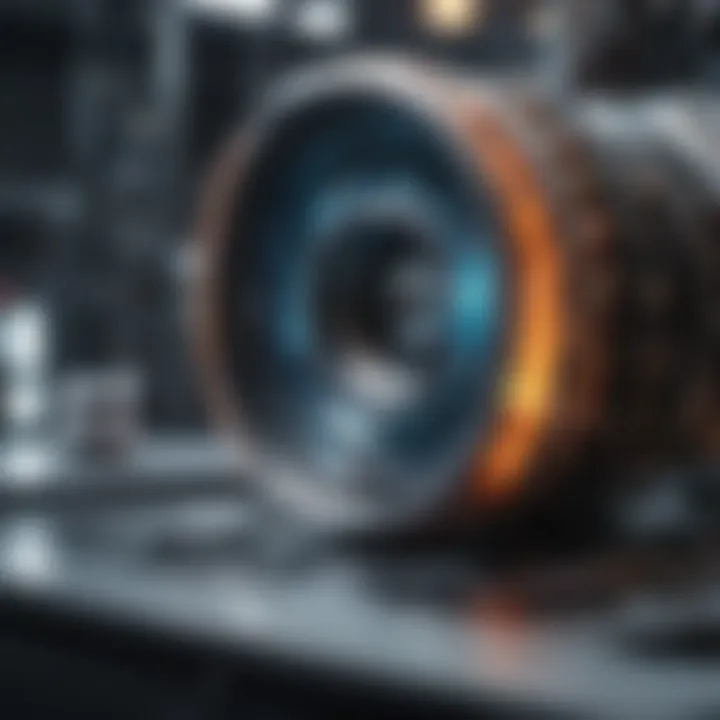
Background Radiation Interference
One of the most significant issues hindering neutron detection efforts is the interference from background radiation. This naturally occurring radiation can muddy the waters when trying to detect neutrons, making it difficult to distinguish between signal and noise. The sources of such background radiation can be varied—cosmic rays, terrestrial sources, and even artificial sources can contribute to this noisy environment.
To mitigate this interference, researchers explore various techniques:
- Shielding: Using dense materials, like lead or polyethylene, can help reduce the presence of unwanted radiation.
- Advanced Filtering Techniques: Employ algorithms and statistical methods to filter out the noise from relevant signals.
- Selective Detection: Building detectors specifically designed to respond to neutrons while ignoring other types of radiation.
Despite these methods, the challenge is continually evolving as new background sources are identified, and detection technologies advance. For scientists and engineers, overcoming this obstacle is not just a technical necessity; it's critical for ensuring the safety and accuracy of neutron measurements in applications ranging from nuclear security to medical imaging.
"The true challenge in neutron detection often lies not in the detection of neutrons themselves but in filtering the chaos that surrounds them."
Cost and Accessibility of Technology
Another substantial challenge that cannot be ignored is the cost and accessibility of neutron detection technologies. The sophisticated materials and technologies required for effective neutron detection often come with hefty price tags. This can be a significant barrier for smaller research institutions or emerging companies looking to engage in neutron-related projects.
The financial implications of developing and maintaining neutron detection systems often lead to the following concerns:
- Limited Funding: Many projects struggle to secure adequate funding for purchasing state-of-the-art detectors, which can stunt innovation and application.
- Economies of Scale: Producing neutron detectors in small quantities raises costs, thereby limiting accessibility even further.
- Training and Maintenance: Beyond the initial purchase price, there are ongoing costs associated with training personnel to use, maintain, and upgrade these technologies.
As the demand for neutron detection equipment continues to rise across various sectors, tackling the issues of cost and accessibility becomes paramount. Initiatives to find alternative, more cost-effective solutions or to improve existing technologies while reducing costs will play a vital role in enhancing the broad use of neutron detection in scientific research and industry alike.
Future Directions in Neutron Detection Research
The realm of neutron detection is ever-evolving, spurred by technological developments and increasing demands across various sectors. As we look towards the future, it’s crucial to keep an eye on the emerging research topics and how these innovations can transcend traditional uses of neutron detection technology. This segment aims to illustrate the significance of these future directions in neutron detection, emphasizing the potential benefits, considerations, and the broader implications for both science and industry.
Emerging Research Topics
In recent years, there has been a noticeable surge in research directly targeting the enhancement of neutron detection capabilities. Some emerging topics worth considering include:
- Advanced Materials: Research is focusing on next-generation materials that can improve detection efficiency and lower costs. Innovations in nanostructured materials, for instance, may allow for more sensitive detection methods that were previously not feasible.
- Neutron Imaging Techniques: Developing new imaging methods that integrate neutron detection with other modalities—like X-ray and gamma-ray imaging—could offer a more comprehensive view when studying materials or biological specimens.
- Artificial Intelligence: Machine learning algorithms are being explored to analyze neutron detection data more effectively. By harnessing the power of AI, researchers hope to automate the detection process, allowing for faster and more accurate results.
This research can lead to significant advancements, making neutron detection not only more efficient but also expanding its applicability across various scientific fields. It’s about shifting gears from merely understanding neutrons to innovatively utilizing them.
Potential for Multidisciplinary Applications
The potential for integrating neutron detection technology into various fields cannot be overstated. Here are a few areas where these applications could flourish:
- Environmental Science: Neutron detection can be pivotal in monitoring environmental radiation levels, assessing nuclear waste, and ensuring regulatory compliance. This data can help in maintaining ecological balance and public safety.
- Medical Applications: There is a burgeoning interest in utilizing neutrons for medical imaging and treatment modalities. From neutron radiography to neutron capture therapy in cancer treatment, the medical field stands to benefit immensely.
- Security Sector: In terms of national and global security, neutron detectors can play a key role in detecting illicit nuclear materials. The integration of neutron detection systems with current security measures could enhance our ability to prevent nuclear terrorism.
By unveiling the potential of neutron detection in these other fields, researchers and practitioners can foster a more interdisciplinary approach that promises richer insights and broader applications. Each of these areas reflects just a fraction of how expanding neutron detection capabilities may influence future developments.
"Future innovations in neutron detection may unlock new scientific frontiers and enhance safety protocols in critical industries."
As we continue to probe the potential of neutron detection, it’s essential to maintain an open dialogue among various disciplines. This cross-pollination of ideas and approaches will be key to driving the next wave of innovation in this vital area of research.
End
In this exploration of neutron detection equipment, we have traversed a landscape rich with innovation, applications, and intricate principles that steer this specialized field. The importance of concluding our discussion on neutron detectors cannot be underestimated, as it encapsulates both a synthesis of the previous sections and a forward-looking perspective. Recognizing the significance of neutron detection in contemporary science and industry empowers us to appreciate its multifaceted roles and future potential.
Summary of Key Points
Throughout the article, we highlighted several critical themes and insights related to neutron detection equipment:
- Diverse Applications: Neutron detectors play a pivotal role in various fields, including nuclear security, medical imaging, and environmental monitoring.
- Technological Advancements: Innovations in detector materials and integration with digital systems continue to enhance detector accuracy and application capabilities.
- Challenges Ahead: Addressing interference from background radiation and the cost of advanced detection technology remain key challenges that the industry must navigate.
- Future Directions: Ongoing research into emerging techniques and interdisciplinary applications holds promise for unlocking new potential uses for neutron detectors.
Each of these points underscores not just the past and present value of neutron detection technology but also its promising future as a fundamental component in numerous scientific and industrial endeavors.
The Role of Neutron Detectors in Science and Industry
Neutron detectors are not merely tools; they are the building blocks of safety, efficiency, and advancement across various sectors. Their role is evident in:
- Nuclear Security: Providing security measures through effective monitoring of nuclear materials can prevent unauthorized use and potential catastrophic events.
- Medical Applications: Their function in medical imaging and targeted therapies showcases how neutrons can aid in treating illnesses, thus promoting health and well-being.
- Environmental Monitoring: With their capability to measure radiation levels, these detectors help in maintaining environmental safety and managing nuclear waste, ensuring the protection of ecosystems and human health.
Taking a broader view, neutron detectors empower researchers and professionals by offering data that informs policies, guides research, and ultimately sustains safe practices in powerful technologies. As the quest for knowledge continues to expand, neutron detection equipment will undeniably be at the forefront, adapting and evolving alongside the challenges and opportunities that lie ahead.