Exploring the Genetics of Sickle Cell Disease
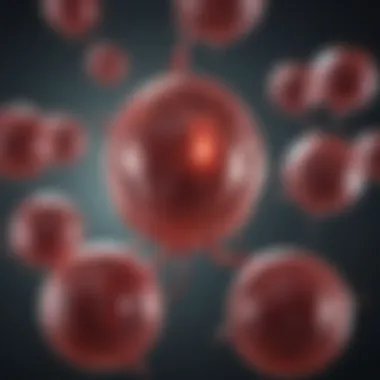
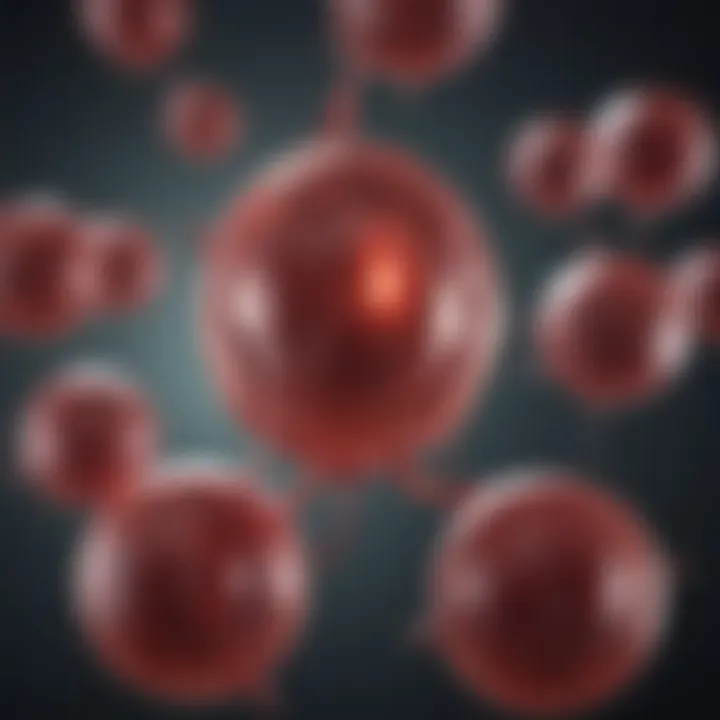
Intro
Sickle cell disease represents a significant public health challenge worldwide. It is a genetic disorder that manifests primarily through the alteration of hemoglobin, the protein responsible for oxygen transport in red blood cells. The disease illustrates how a single mutation in the HBB gene can lead to a range of clinical outcomes and complications. Understanding the mechanisms underlying this condition is essential for both clinicians and researchers alike.
This article provides a in-depth exploration of the genetic foundation of sickle cell disease. It highlights the specific mutations and the molecular processes that lead to abnormal hemoglobin synthesis. Additionally, it examines how these genetic aspects affect health outcomes, patterns of inheritance, and the latest research efforts in treatment and prevention.
Methodology
Study Design
The approach to understanding sickle cell disease encompasses studies ranging from biochemical assessments to genetic sequencing. These methods allow scientists to observe mutations at the DNA level and how these relate to physical symptoms and lab results.
Data Collection Techniques
Data collection varies across studies and may include:
- Genetic Testing: Utilizes methods such as whole genome sequencing to identify mutations in the HBB gene.
- Clinical Evaluations: Patients undergo tests to assess the frequency of sickle cell crises and other complications.
- Longitudinal Studies: Collection of data over extended periods, providing insight into the disease trajectory.
The identification of genetic mutations in sickle cell disease not only aids in diagnosis but also holds promise for future therapies.
Discussion
Interpretation of Results
Results from various studies consistently show that mutations in the HBB gene lead to the production of abnormal hemoglobin known as hemoglobin S. These mutations disrupt normal red blood cell function, converting flexible cells into rigid, sickle-shaped ones. This deformation significantly impacts blood flow and oxygen delivery, leading to various complications.
Limitations of the Study
Despite advancements in research, challenges remain. Many studies focus on specific populations, which can limit the generalizability of findings. Moreover, the complexity of the disease and its interactions with other health conditions can obscure direct relationships between genotype and phenotype.
Future Research Directions
Ongoing research is crucial to enhance our understanding of sickle cell disease. Key areas include:
- Gene Therapy: Investigating potential methods to correct the underlying genetic mutation.
- Targeted Treatments: Developing drugs that can specifically address the challenges posed by sickle-shaped cells.
- Epidemiological Studies: Understanding how environmental and genetic factors influence disease severity across diverse populations.
Prologue to Sickle Cell Disease
Sickle cell disease (SCD) represents a significant public health challenge. It is not just a medical condition but a hereditary disorder affecting numerous individuals globally. Understanding SCD encompasses examining various factors, such as its definition, historical context, genetics, and clinical implications. This section aims to highlight the essential aspects of sickle cell disease that are crucial for any further discussion.
Definition and Overview
Sickle cell disease is a group of inherited blood disorders characterized by a specific mutation in the hemoglobin gene. This mutation causes the hemoglobin molecules to form abnormally, leading to a distinctive sickle shape in red blood cells. Unlike normal, flexible red cells, sickle-shaped cells can become rigid and sticky, causing blockages in blood vessels. This obstruction leads to pain, organ damage, and recurrent infections, all typical clinical manifestations of the disease. Understanding this definition is fundamental to grasp the consequences and challenges faced by those living with sickle cell disease.
Historical Context
The history of sickle cell disease is rich and complex, with roots traced back to ancient times. The first description of sickle-shaped red blood cells was made in 1910, but it wasn't until the early 1940s that scientists identified the genetic basis of the disease. This delayed understanding kept many patients unaware of their condition for years. With time, research developed more insights into the inheritance patterns and biological mechanisms responsible for this disorder. Moreover, public health efforts have started to educate communities about sickle cell disease, especially in regions where it is prevalent. This historical narrative sets the foundation for ongoing discussions around prevention, treatment, and future research directions in the realm of SCD.
Genetics of Sickle Cell Disease
The genetics of sickle cell disease (SCD) is a vital component of understanding this complex disorder. This section emphasizes the role of genetic mutations, the inheritance patterns, and the implications that these factors have on health outcomes. Recognizing how genetic variations lead to the distinct clinical manifestations of SCD helps clarify the disease process and informs treatment strategies.
Understanding Genetic Mutations
Genetic mutations are changes in the DNA sequence that can lead to the development of various conditions, including sickle cell disease. The specific mutation that characterizes SCD occurs in the HBB gene, which provides instructions for the production of hemoglobin beta chains. This mutation alters the structure of hemoglobin, resulting in the polymerization that forms crescent-shaped red blood cells under certain conditions. Understanding these mutations is critical because they are the foundation for the pathology of sickle cell disease.
Types of Genetic Mutations
Point Mutations
Point mutations are the most common type of genetic alteration. They involve the substitution of a single nucleotide in the DNA sequence. In the context of sickle cell disease, a point mutation occurs at the sixth codon of the HBB gene, where adenine (A) is replaced with thymine (T). This single nucleotide change transforms the amino acid residue from glutamic acid to valine. Point mutations are significant because they can lead to catastrophic effects in the protein functionality, as seen in the altered hemoglobin structure in SCD. A key characteristic of point mutations is this simple yet profound impact on the protein's function, making them a central focus of genetic studies regarding SCD.
Insertions and Deletions
Insertions and deletions refer to the addition or removal of one or more nucleotides in the DNA sequence. These types of mutations can also lead to significant alterations in the protein produced, often resulting in a substantial change in function or instability of the molecule. In relation to sickle cell disease, while not directly causing the typical hemoglobin mutation, these genetic events can complicate the disorder. They can lead to various forms of thalassemia if they occur in related genetic regions. Their complexity adds depth to the genetic understanding of sickle cell disease, even though they may not be the direct cause.
Chromosomal Mutations
Chromosomal mutations involve alterations in the structure or number of chromosomes, which can disrupt the normal functioning of genes. These mutations can lead to a loss of genetic material or an abnormal gene dosage. Although chromosomal mutations are not directly responsible for sickle cell disease, certain chromosomal abnormalities can increase the risk of complications. The presence of additional trisomies or deletions may exacerbate the condition's symptoms or lead to other hematologic issues. Understanding these mutations contributes to a holistic view of the genetics behind sickle cell disease, highlighting their potential implications during clinical assessments.
Genetic mutations play a crucial role in determining the clinical trajectory of sickle cell disease, shaping both health outcomes and treatment strategies.
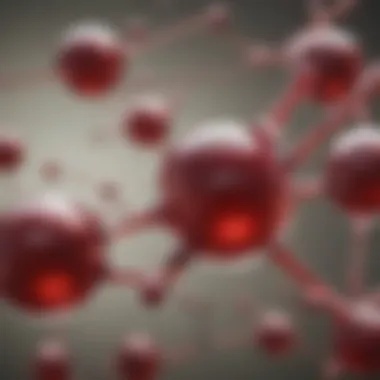
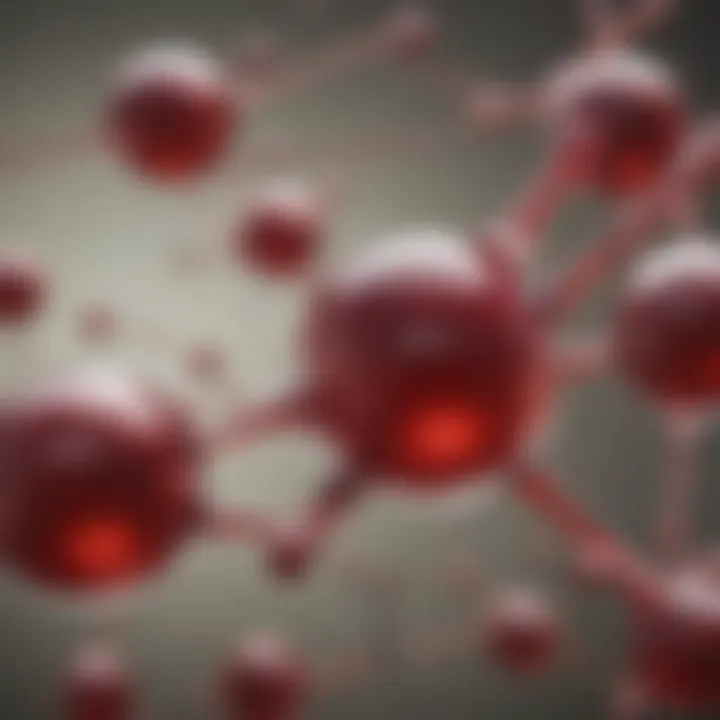
The exploration of genetic mutations, especially point mutations, gives insight into the mechanics of SCD. This understanding can help inform future therapeutic approaches aimed at correcting or compensating for these mutations. The interplay between various mutation types extends the conversation about this disease, facilitating a deeper comprehension of the conditions that affect patients and guiding ongoing research efforts.
The Specific Mutation Causing Sickle Cell Disease
Sickle cell disease is fundamentally linked to a specific genetic mutation within the HBB gene. Understanding this mutation is vital as it helps illustrate the disease's biological mechanisms and informs potential treatment avenues. By examining the mutations' role in shaping hemoglobin, we can better grasp the implications for patient health and management. Moreover, this knowledge can pave the way for innovations in both diagnostic testing and therapeutic research.
Hemoglobin and Its Structure
Hemoglobin is a protein located in red blood cells that is essential for oxygen transport throughout the body. This protein is composed of two types of polypeptide chains - alpha and beta chains. Each hemoglobin molecule consists of four chains, forming a tetramer. The proper structure of hemoglobin is critical to its function because only a correctly assembled molecule can effectively bind and release oxygen. When mutations occur within this protein, it can alter how hemoglobin behaves, impacting overall health and leading to complications typical of sickle cell disease.
Mutation in the HBB Gene
The mutation responsible for sickle cell disease occurs specifically in the HBB gene, which encodes the beta chain of hemoglobin.
Location and Function of the HBB Gene
The HBB gene is located on chromosome 11. It plays a crucial role in coding for the beta-globin subunit of hemoglobin. A key characteristic of the HBB gene is its involvement in regulating normal hemoglobin production. Given this function, alterations in the HBB gene can lead directly to the formation of abnormal hemoglobin S. This abnormality can have significant implications for individuals with sickle cell disease, as it is the blueprint that dictates the properties of the hemoglobin produced. The unique feature of the HBB gene's mutation underlines its relevance in sickle cell pathology, making it a central focus for both clinical and research contexts in terms of diagnosis and potential therapies.
Impact of the Mutation on Hemoglobin Structure
The mutation in the HBB gene substitutes adenine for thymine at the sixth codon, resulting in the replacement of glutamic acid with valine. This seemingly small change induces significant structural alterations in hemoglobin. The key characteristic of this mutated form is its tendency to polymerize under low oxygen conditions, causing the red blood cells to assume a rigid sickle shape. This structural change disrupts normal blood flow and oxygen transport. The unique aspect of this mutation is that it manifests in both symptomatic and asymptomatic individuals, which emphasizes the need for comprehensive understanding of its impact. Furthermore, the mutation's drawbacks make it a prime target for gene therapy research, as there is potential to reverse the effects of sickle cell disease by modifying the hemoglobin structure itself.
Mechanism of Sickle Cell Formation
Understanding the mechanisms behind sickle cell formation is essential for grasping the broader implications of sickle cell disease. Abnormalities in hemoglobin lead to the sickling of red blood cells. This section will explore how these changes occur, their impact on the body, and the resultant clinical effects. Each section will lay out the specific problems sickle cell disease causes and contribute to a well-rounded understanding of its complexities.
Abnormal Hemoglobin and Cell Shape
Hemoglobin plays a crucial role in oxygen transport within the bloodstream. In sickle cell disease, the abnormal version, known as hemoglobin S, forms due to a point mutation in the HBB gene. When oxygen is released from hemoglobin S, it causes red blood cells to change shape from the usual disc to a crescent or sickle shape.
These sickle-shaped cells are rigid and inflexible. They can block blood flow, which leads to pain and other complications. Red blood cells in their normal form are generally flexible and can easily navigate through small blood vessels. The significance of abnormal hemoglobin cannot be overstated; it is central to the pathology of the disease.
Consequences of Sickle-shaped Cells
Decreased Oxygen Transport
Decreased oxygen transport is a direct result of the distortion of red blood cells. Sickle cells are less efficient at carrying oxygen due to their shape. This low efficiency contributes to severe fatigue and can lead to complications like organ damage over time.
One key characteristic is that the sickle cells do not easily release oxygen to tissues, creating a state of hypoxia. This is a critical point for understanding why patients suffer from pain crises, as tissues starve for oxygen.
Potential for Vascular Blockage
The potential for vascular blockage occurs when sickle-shaped cells stick to blood vessel walls. This adherence leads to occlusions, which can severely restrict blood flow. Areas of the body with higher oxygen demands, such as the brain and the spleen, are particularly vulnerable.
A significant aspect of vascular blockage is its sudden onset. This unpredictability contributes to severe pain emergencies, requiring immediate medical attention. The patterns of blood flow are disrupted, impacting overall health and quality of life for those affected by the disease.
Impact on Organ Function
The impact on organ function results from both decreased oxygenation and vascular blockage. Organs can suffer irreversible damage due to chronic oxygen deprivation. For example, repetitive blocking of blood flow to the spleen can ultimately lead to functional asplenia, increasing the risk for infections.
Furthermore, organs such as the kidneys and liver may also be affected due to poor blood supply. This highlights the systemic nature of sickle cell disease, as complications extend beyond just painful crises; they encompass a myriad of potential organ dysfunctions.
"Understanding the mechanism of sickle cell formation is critical in developing targeted treatments and interventions."
Sickle cell disease is not merely about managing symptoms. It involves a comprehensive understanding of how abnormal hemoglobin influences the body. Recognizing the complexities of sickle-shaped cells and their implications on organ function and oxygen transport can help inform more effective treatment strategies.
Inheritance Patterns of Sickle Cell Disease
The patterns of inheritance for sickle cell disease are crucial for understanding its transmission within families and its broader implications for population health. This section will explore how the disease is passed from parents to children, highlighting significant aspects of genetic inheritance. Gaining knowledge in this area can aid in genetic counseling, early diagnosis, and implementing preventive strategies.
Autosomal Recessive Inheritance
Sickle cell disease follows an autosomal recessive inheritance pattern. This means that an individual must inherit two copies of the mutated HBB gene, one from each parent, to develop the disease. If a person has one normal copy of the gene and one mutated copy, they are considered a carrier and typically do not exhibit symptoms. This phenomenon illuminates the significance of genetic counseling, especially in communities with a high prevalence of the disease.
- Key Characteristics:
- Implications:
- Individuals with sickle cell disease possess two copies of the mutated gene.
- Carriers have one normal and one mutated gene, showing no symptoms.
- The risk for offspring is 25% if both parents are carriers.
- Parents may not know they are carriers, emphasizing the need for genetic testing.
- Understanding inheritance helps in planning families, especially in high-risk populations.
Carrier State (Sickle Cell Trait)
The carrier state of sickle cell disease is referred to as sickle cell trait. This condition is vital to recognize because carriers have one normal hemoglobin gene and one mutated version. While carriers usually remain asymptomatic, they can pass the mutated gene to their children.
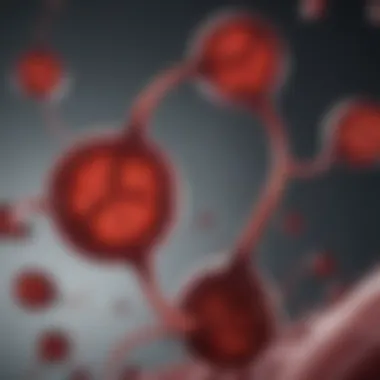
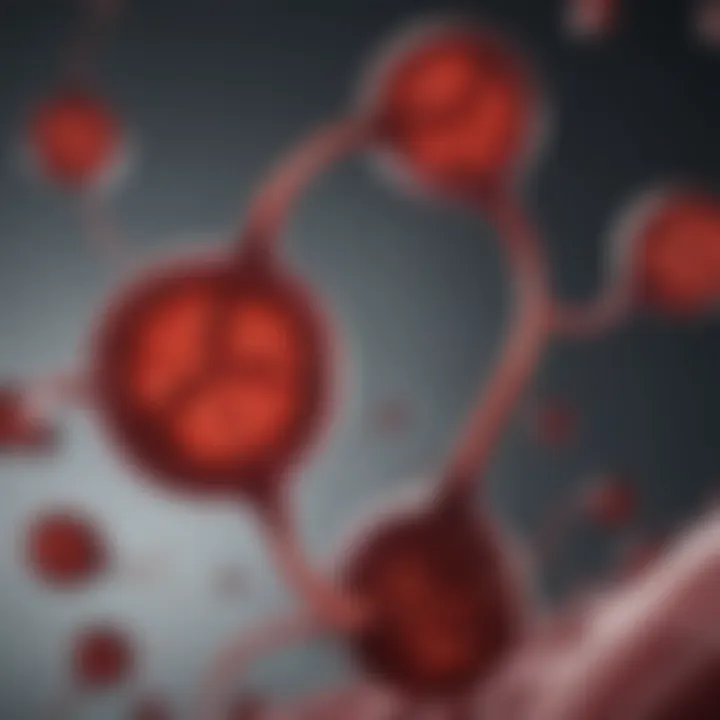
- Prevalence:
- Carrier Testing:
- Impact on Offspring:
- Sickle cell trait affects approximately 1 in 12 African Americans and similar frequencies in other populations.
- Genetic tests can confirm if a person carries the sickle cell trait. Understanding one's carrier status is indispensable for reproductive decisions.
- If two carriers have children, there is a 50% chance of passing the trait and a 25% chance of having a child with sickle cell disease.
“Knowing one's genetic status can drastically alter life decisions and implications for future health.”
In summary, understanding the inheritance patterns of sickle cell disease and the implications involved can empower individuals and families to make informed health decisions. Awareness of autosomal recessive inheritance and the carrier state will aid in decreasing transmission rates and increase community health overall.
Clinical Manifestations of Sickle Cell Disease
Understanding the clinical manifestations of sickle cell disease is crucial because they illustrate how the genetic mutations within the HBB gene affect patients' lives. These manifestations provide insight into the systematic impact of sickle cell disease on the human body. Knowledge of these symptoms helps in diagnosing and managing the illness effectively, aiding healthcare professionals in providing tailored care to improve quality of life for those affected.
Common Symptoms
Pain Crises
Pain crises are one of the hallmark symptoms of sickle cell disease. They occur when sickle-shaped cells obstruct blood flow in small blood vessels, leading to severe pain in various body parts. This symptom is a direct consequence of the abnormal hemoglobin structure. Pain crises can last from hours to days and significantly affect a patient's daily activities. Its unpredictable nature makes it particularly burdensome, resulting in emergency room visits and hospitalizations. Recognizing the commonality and the triggers of pain crises is essential as it sets the stage for preventative measures and effective pain management strategies.
Anemia
Anemia is another prominent symptom, marked by a deficit of healthy red blood cells to supply oxygen to the body's tissues. In sickle cell disease, red blood cells break down faster than they can be produced, leading to chronic anemia. This causes fatigue, weakness, and a general decline in physical performance. Understanding the connection between anemia and sickle cell disease underscores the need for regular blood tests to monitor hemoglobin levels. Addressing anemia can significantly improve the overall well-being of patients, allowing them to lead more active and productive lives.
Frequent Infections
People with sickle cell disease are more susceptible to frequent infections. This is largely due to spleen dysfunction, as sickle-shaped cells damage the spleen, an organ that plays a vital role in filtering bacteria from the blood. Frequent infections can lead to serious complications, demanding urgent medical attention. The importance of vaccinations and prophylactic antibiotics emerges from this facet of the illness. By focusing on prevention, healthcare providers can reduce the risk of infections, which ultimately enhances the quality of life for those living with sickle cell disease.
Complications Associated with the Disease
Spleen Dysfunction
Spleen dysfunction is a critical complication stemming from sickle cell disease. As mentioned, the spleen is essential for fighting infections. In this condition, repeated episodes of sickling can damage the spleen over time, leading to its reduced ability to function effectively. This dysfunction can result in conditions like functional asplenia, increasing the risks of severe infections. Recognizing spleen dysfunction is important for proper medical guidance and the implementation of preventive measures.
Stroke Risk
The risk of stroke is significantly heightened in individuals with sickle cell disease. Strokes can result from blocked blood vessels in the brain due to sickled cells, especially in children. This serious complication emphasizes the need for regular medical check-ups and monitoring. Understanding the increased stroke risk highlights the necessity for early interventions, such as the use of blood transfusions or other therapies aimed to maintain healthy blood flow and reduce the risks associated with sickle cell disease.
Acute Chest Syndrome
Acute chest syndrome is a severe lung-related complication that can arise in patients with sickle cell disease. It is characterized by chest pain, fever, and difficulty in breathing. This syndrome may be triggered by infections or vaso-occlusive events, posing a serious threat to patient health. Recognizing the symptoms and timely intervention are vital, as acute chest syndrome can lead to critical health outcomes. Understanding its mechanisms can drive further research to improve treatment strategies and patient prognoses.
Diagnosis of Sickle Cell Disease
The diagnosis of sickle cell disease is crucial in managing this hereditary condition effectively. Early detection allows for timely interventions, which can significantly improve patient outcomes and enhance quality of life. Understanding the various diagnostic methodologies is essential for healthcare professionals, patients, and their families.
Sickle cell disease can present with complex symptoms, making diagnosis challenging. However, advancements in genetic testing and screening have facilitated a clearer understanding of the disease. The diagnosis relies on two main categories of tests: screening and confirmatory tests.
Screening Tests
Screening tests serve as initial assessments to detect the presence of hemoglobin abnormalities associated with sickle cell disease. These tests are generally performed on newborns during routine examinations. Key screening methods include:
- Hemoglobin Electrophoresis: This technique separates different types of hemoglobin based on their electrical charge. It helps detect abnormal hemoglobins like hemoglobin S, which is primarily responsible for sickle cell disease.
- Complete Blood Count (CBC): This test evaluates overall health and can identify anemia, which is a common complication of sickle cell disease. Low hemoglobin levels may prompt further testing.
- Newborn Screening Programs: Many countries have implemented universal newborn screening programs to identify sickle cell disease at birth, leading to early interventions and care.
These screening tests are accessible and relatively straightforward. They provide the first layer of defense in diagnosing sickle cell disease, enabling healthcare providers to initiate appropriate management strategies.
Confirmatory Tests
Once screening tests indicate a possible presence of sickle cell disease, confirmatory tests are required to verify the diagnosis. These tests provide definitive evidence of the condition and its specific type. Common confirmatory tests include:
- DNA Analysis: Genetic testing can determine mutations in the HBB gene responsible for sickle cell disease. This type of analysis provides information on whether the disease is present and helps in family planning.
- Sickle Cell Test: In cases where hemoglobin electrophoresis results are inconclusive, a sickle cell test can confirm the presence of sickle cells in the blood.
"Genetic testing not only aids in diagnosis but also supports targeted therapies and counseling for affected families."
Confirmatory tests play a pivotal role in ensuring accurate diagnosis, which is fundamental for therapeutic decisions. Accurate specificity in tests ensures patients receive appropriate treatment, effectively mitigating complications linked to sickle cell disease.
Treatment Options for Sickle Cell Disease
Effective management of sickle cell disease is crucial for improving the quality of life and outcomes for patients. Treatment options consist of various strategies aimed at alleviating symptoms, reducing complications, and modifying disease progression. Understanding these options may empower patients and caregivers in their decisions regarding care and treatment that best suit individual needs.
Pain Management Strategies
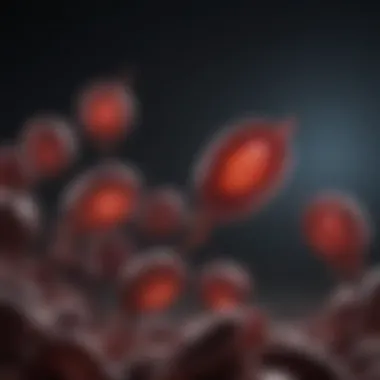
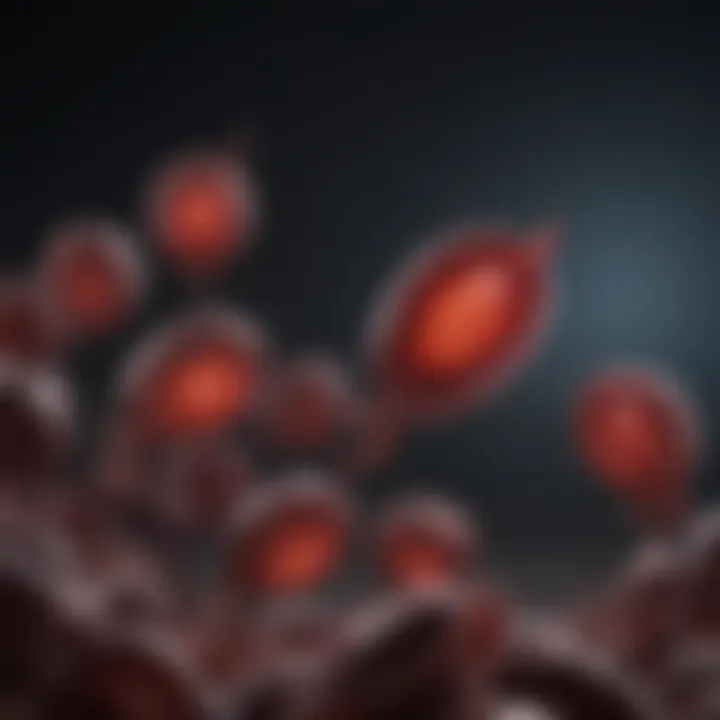
Pain management is a primary focus for individuals with sickle cell disease. Patients frequently experience pain crises due to the blockage of blood flow by sickle-shaped cells. Treatments often start with over-the-counter medications like acetaminophen or ibuprofen. However, stronger medications, such as opioids, may be required for severe pain.
In addition to medication, non-pharmacological interventions play a key role. These may include hydration, warm compresses, and relaxation techniques. Alternative therapies, such as acupuncture and physical therapy, are also found to provide relief to some patients. Regular consultations with healthcare professionals are important to tailor pain management plans to each patient's specific situation.
Disease-modifying Therapies
Disease-modifying therapies aim to change the course of sickle cell disease, potentially reducing the frequency and severity of crises.
Hydroxyurea
Hydroxyurea is a key treatment for sickle cell disease. It works by stimulating the production of fetal hemoglobin, which does not sickle. This can decrease the number of painful episodes and reduce the need for blood transfusions.
The primary benefit of hydroxyurea is its ability to improve the overall health of patients. Clinical studies indicate that it significantly reduces complications associated with the disease. However, some drawbacks to consider include the potential for side effects, such as suppression of bone marrow function. Thus, regular monitoring is crucial when a patient is on hydroxyurea.
Blood Transfusions
Blood transfusions are a well-established method for managing sickle cell disease. Transfusions help by increasing the number of normal red blood cells in circulation, which can alleviate anemia and reduce the risk of stroke.
A notable characteristic of blood transfusions is their immediate effectiveness. They provide rapid relief from symptoms and can be lifesaving in acute situations. However, transfusions must be performed with caution since repeated transfusions can lead to complications such as iron overload. This necessitates monitoring and possibly further treatments to prevent excess iron in the body.
Bone Marrow Transplant
Bone marrow transplant is the only potential cure for sickle cell disease. This procedure involves replacing a patient's bone marrow with healthy marrow from a compatible donor. It effectively addresses the underlying cause of sickle cell disease, potentially leading to a complete resolution of symptoms.
The key characteristic of this treatment is its curative potential. However, it is a complex procedure with significant risks. Finding a suitable donor can also be a challenge. Because of these factors, bone marrow transplant is usually considered for younger patients with severe disease who are otherwise healthy.
"While multiple treatment options exist for sickle cell disease, the choice of therapy should be individualized based on specific patient needs and circumstances."
In summary, the management of sickle cell disease encompasses pain management and disease-modifying therapies like hydroxyurea, blood transfusions, and bone marrow transplant. Each treatment has its own benefits and considerations, highlighting the importance of personalized care.
Current Research Directions in Sickle Cell Disease
Research on sickle cell disease is at the forefront of genetic and medical innovation. Understanding the genetic basis of this condition is crucial for developing effective treatments. As scientists delve deeper into the mechanisms behind the disease, they uncover new opportunities to improve patient outcomes.
Gene Therapy Approaches
Gene therapy has emerged as a promising approach in tackling sickle cell disease. This strategy involves altering the genes responsible for the disease. Researchers aim to correct the specific mutation in the HBB gene that causes abnormal hemoglobin production. Techniques like CRISPR-Cas9 have shown significant potential in this area. The precision of this tool allows for accurate editing of genes, potentially correcting the mutation that leads to sickle cell formation.
In clinical trials, gene therapy has shown to achieve remarkable results. Patients treated with these methods often experience a reduction in symptoms and complications associated with sickle cell disease. However, challenges remain. Ensuring the safety and efficacy of these therapies is essential. Long-term outcomes of gene therapy require thorough monitoring and investigation.
- Considerations for Gene Therapy:
- Ethical implications of genetic modifications
- Accessibility of treatment for diverse populations
- Long-term effectiveness and monitoring standards
Innovative Drug Developments
Pharmaceutical research is another key area exploring new treatments for sickle cell disease. New drugs aim to address various aspects of the disease pathology. For instance, medications like voxelotor aim to increase hemoglobin's affinity for oxygen. This can improve overall oxygen transport in patients. Other drugs focus on reducing the frequency of pain crises, which are common in sickle cell patients.
Moreover, extensive research is being conducted to repurpose existing medications for sickle cell disease. Hydroxyurea, traditionally used for other conditions, is finding new roles in managing sickle cell by reducing pain episodes and the need for blood transfusions.
Here are some notable innovative drug developments:
- Voxelotor
- L-glutamine
- Pomalidomide
The combination of pharmacotherapy and gene therapy holds significant promise for the future of sickle cell disease management. Integrating these approaches could lead to holistic treatment regimens aimed at improving patients’ quality of life.
"Progress in understanding the genetic mechanisms behind sickle cell disease paves the way for breakthroughs in gene therapy and drug development."
Continued investment in research is essential. Ongoing studies are needed to unlock new avenues for treatment while improving the lives of those affected by this debilitating condition.
Epilogue and Future Perspectives
Summation of Key Points
In summation, several pivotal points emerge from our exploration of sickle cell disease:
- Definition: Sickle cell disease is a hereditary condition caused by a mutation in the HBB gene, which codes for the beta-globin subunit of hemoglobin.
- Genetic Mechanism: The key mutation leads to the production of abnormal hemoglobin (HbS), resulting in distorted red blood cell shapes.
- Clinical Manifestations: Common symptoms include pain crises, anemia, and the risk of complications such as strokes and acute chest syndrome.
- Diagnosis and Treatment: Techniques such as blood tests confirm the presence of the disease, while treatments vary from pain management strategies to disease-modifying therapies, like hydroxyurea.
- Research Directions: The future looks promising with advancements in gene therapy and innovative drug developments aimed at improving patient outcomes.
These points establish a clearer picture of the disease and its profound impact on individuals and communities.
Implications for Future Research and Treatment
The implications for future research and treatment in sickle cell disease are significant and multifaceted. Further studies are critical to:
- Enhance Understanding: Improved knowledge about the genetic basis can lead to advancements in gene editing techniques, potentially correcting the mutations at their source.
- Develop New Therapies: Continuous efforts are needed to create and refine drug therapies that can manage symptoms more effectively and reduce complications.
- Educate Communities: Public education initiatives focused on genetic awareness can help in early diagnosis and management, particularly in communities at risk.
As research progresses, treatments must become more personalized, focusing on the unique genetic profiles of individuals. This tailored approach could increase the efficacy of therapies, enabling better quality of life for patients.
"The advancement of research in sickle cell disease not only alters the fate of individuals but also reshapes the narrative surrounding genetic disorders fundamentally."