Exploring the Role of Protein Sigma in Biology
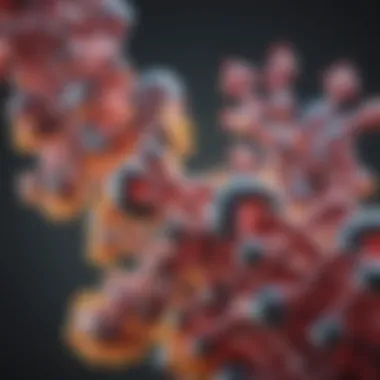
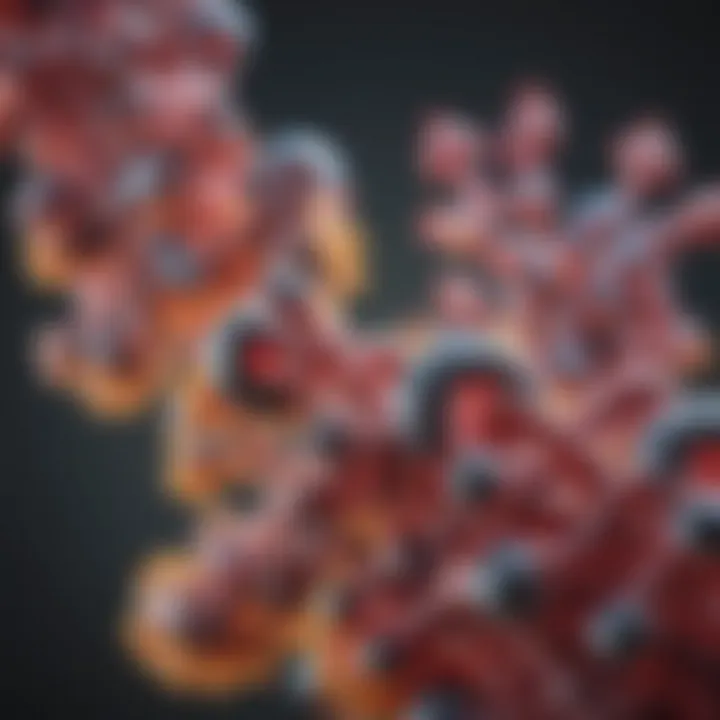
Intro
Protein sigma represents a crucial player within biological systems, manifesting varying roles that significantly impact molecular biology and biotechnology. This article aims to uncover the intricate structure and function of sigma proteins, while highlighting their relevance across different scientific and clinical environments. Given the increasing interest in the implications of these proteins in disease and therapy, understanding protein sigma has never been more pivotal.
This exploration will begin with a comprehensive methodology section, laying the groundwork for the subsequent discussion that follows. By diving into the genetic foundations and interactions within cellular ecosystems, we will present a thorough examination of sigma proteins and their extended significance.
Methodology
Study Design
The study on protein sigma is designed with a multi-faceted approach, integrating both qualitative and quantitative research methods. This comprehensive framework allows for an in-depth analysis of existing literature alongside new experimental findings. The goal is to derive a coherent understanding of how sigma proteins function at both genetic and cellular levels.
Data Collection Techniques
Data collection relies on various robust techniques, including:
- Literature Review: An exhaustive review of peer-reviewed articles, textbooks, and relevant biological databases ensures a well-rounded perspective.
- Experimental Studies: By conducting laboratory experiments, researchers observe sigma protein interactions and their effects on cellular behavior. This involves techniques such as Western blotting and microscopy to visualize protein expression.
- Bioinformatics Tools: Analyzing genetic sequences and protein structures adds a computational element, revealing insights into functional genomics associated with sigma proteins.
Discussion
Interpretation of Results
Findings derived from the methodology reveal that sigma proteins are vital for cellular processes. Their role often extends beyond mere structural functions; they are involved in gene regulation and cellular signaling pathways. This suggests a complexity in function that warrants further exploration.
Limitations of the Study
Despite insightful findings, certain limitations must be acknowledged. Variabilities in experimental conditions can affect outcomes. Additionally, the growing body of literature may harbor conflicting results, raising questions about the consistency in sigma protein behaviors across species.
Future Research Directions
Future research should consider:
- Exploring the role of sigma proteins in less-studied organisms, providing a broader evolutionary context.
- Investigating the implications of sigma proteins in various diseases, notably their potential as therapeutic targets.
- Employing newer technologies, such as CRISPR, to modify sigma protein expression and study resultant phenotypic changes.
In summation, the insights gathered through this exploration mark a significant stride towards understanding protein sigma. With continuous advancements in research methodologies, the landscape of sigma protein study promises to evolve, unlocking further secrets that lie within these essential biological entities.
Prelude to Protein Sigma
The role of protein sigma is fundamental in various biological processes, particularly regarding transcription in prokaryotic and eukaryotic organisms. Understanding sigma proteins allows researchers to comprehend gene regulation, cellular response mechanisms, and even implications in health and disease. This section will introduce the significance of protein sigma, breaking down its core characteristics and history to provide a solid foundation for further exploration of its functions and applications.
Definition and Basic Overview
Protein sigma, commonly referred to in the context of sigma factors, is a vital component of RNA polymerase in prokaryotic cells. These proteins are essential for initiating transcription, which is the first step in expressing genes. Sigma factors bind loosely to RNA polymerase and promote the enzyme's attachment to specific promoter regions on DNA. This process is crucial because it determines which genes are expressed at any given time, thus playing a key role in cell function and adaptation.
In terms of structure, sigma factors are distinct proteins characterized by their modular architecture. They typically contain multiple domains essential for their interaction with both the RNA polymerase and DNA. The unique amino acid sequences and structural configurations found in these proteins allow for the specificity required in recognizing promoter regions. Sigma proteins are diverse, with various types known to be responsive to environmental signals, facilitating the organism's adaptation.
Historical Context
The discovery and understanding of sigma proteins can be traced back to the mid-20th century when researchers began unraveling the complexities of genetic material and its transcription. Initial studies in bacteria, specifically E. coli, led to the identification of sigma factors as critical elements in gene regulation. Such discoveries paved the way for later research aimed at exploring the distinct functions of sigma proteins across different species.
As scientists delved deeper into molecular biology, the focus shifted toward eukaryotic organisms. This is where sigma proteins' functions gained attention, shedding light on their significance beyond prokaryotic systems. The historical evolution of research on sigma factors illustrates their increasing relevance in understanding cellular processes, as well as their implications in various fields, including genetics and biotechnology.
The present-day study of protein sigma continues to expand, bridging gaps across disciplines. Advancements in techniques like CRISPR and next-generation sequencing further enhance comprehension of these proteins, thus reinforcing their critical role in biological sciences.
Structural Characteristics
The structural characteristics of sigma proteins are integral to their diverse roles within biological systems. These unique structural features determine how sigma proteins interact with other molecules, influence gene expression, and participate in various cellular processes. Understanding the structural aspects of these proteins enhances our comprehension of their functionality and significance in research and clinical applications.
General Structure of Sigma Proteins
Sigma proteins generally have a well-defined structure that allows them to perform their functions. They are typically composed of several domains, each contributing to specific activities. The core component of sigma proteins is the region responsible for binding to RNA polymerase. This interaction is essential for the initiation of transcription, making the structure of sigma proteins crucial.
Additionally, sigma proteins vary among different species. For instance, Escherichia coli has several distinct sigma factors, each adapted to specific environmental conditions. This adaptability showcases the flexible structural nature of sigma proteins, enabling them to effectively govern transcription in response to various physiological demands.
Domain Architecture
The domain architecture of sigma proteins highlights their specialized roles. Each domain typically correlates to a particular function, such as DNA binding or interaction with RNA polymerase. The combination of these domains within a sigma factor shapes its ability to recognize promoter sequences in DNA, which is critical for the accurate initiation of transcription.
For example, in E. coli, the sigma70 factor features a conserved core domain that interacts with the RNA polymerase. This domain allows for the selective recognition of promoters. The presence of additional domains can further enhance its function, such as providing stability or facilitating interactions with regulatory proteins.
Conformational Variability
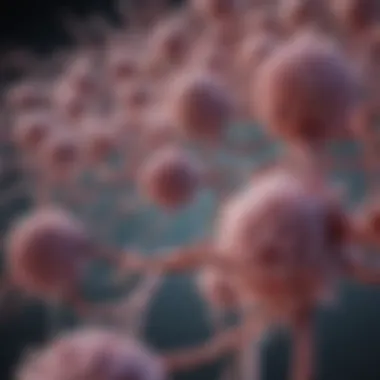
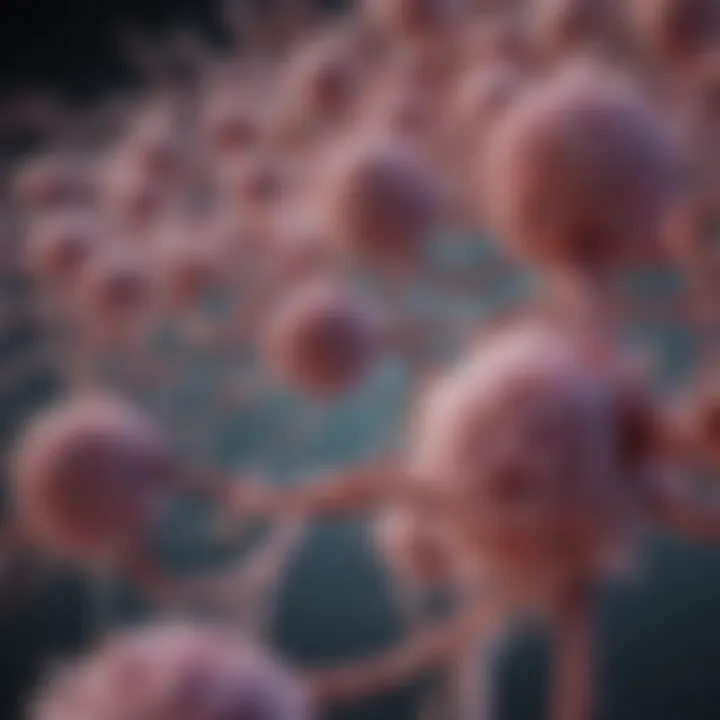
Conformational variability plays a significant role in the functionality of sigma proteins. These proteins must adapt their structures to bind effectively to different DNA sequences and polymerase complexes. This dynamic nature allows sigma proteins to switch conformations in response to environmental signals or cellular needs.
For instance, sigma factors can undergo significant conformational changes upon binding to different core RNA polymerases. This flexibility enables the sigma factors to fine-tune their binding affinities and adapt to various transcriptional landscapes. Variability also allows for fine modulation of activity, essential for responding to cellular stress or alterations in growth conditions.
"Understanding the structural characteristics of sigma proteins is key to unraveling their diverse functions in transcriptional regulation."
In summary, the structural characteristics of sigma proteins provide foundational knowledge that supports their overarching role in cellular function. Their general structure, domain architecture, and conformational variability collectively contribute to the complexity and adaptability of sigma proteins in biological systems.
Functional Roles of Sigma Proteins
Sigma proteins play a crucial role in cellular processes by regulating gene expression and facilitating transcription. Their influence extends beyond basic cellular mechanisms, into areas such as stress response and regulatory framework within various organisms. This section details the specific roles of sigma proteins that are critical for understanding their importance in both prokaryotic and eukaryotic systems.
Transcription Initiation
Transcription initiation is perhaps the most well-characterized function of sigma proteins. They are essential for RNA polymerase to properly start the transcription of specific genes. Sigma factors bind to the core RNA polymerase, forming a holoenzyme that is necessary for recognizing promoter regions of DNA. The binding occurs at consensus sequences, which are short conserved sequences, allowing precise initiation of transcription.
The interaction between sigma factors and promoters is selective. Each sigma factor recognizes distinct promoters, which reflects how genes are regulated differently in response to environmental signals. For instance, in Escherichia coli, the principal sigma factor, Ο70, facilitates the transcription of housekeeping genes. Alternatively, Ο54 is involved in nitrogen metabolism under specific conditions, showcasing the adaptability of sigma proteins to changing environments.
Regulatory Functions
Beyond initiating transcription, sigma proteins also participate in regulatory functions that fine-tune gene expression. They enable cells to respond to a variety of stimuli, such as nutrient availability or stress. This adaptive capability is critical in maintaining homeostasis and allowing efficient cellular responses.
Some regulatory functions include:
- Transcriptional activation and repression: Sigma factors can interact with other proteins to regulate the transcriptional machinery, enhancing or silencing gene expression as needed.
- Response to environmental stress: Certain sigma factors are activated under stress conditions, such as heat shock or oxidative stress, ensuring the expression of specific protective genes.
- Coordination of cellular processes: By regulating multiple genes, sigma proteins contribute to significant cellular processes like sporulation in bacteria, allowing for survival in unfavorable conditions.
Interactions with RNA Polymerase
The interactions between sigma proteins and RNA polymerase are fundamental for the execution of transcription. The formation of the RNA polymerase-sigma complex is necessary for promoter recognition and binding. This relationship allows for a highly controlled process where sigma proteins dictate which genes are transcribed based on the cellular context.
During transcription, sigma factors can dissociate from the RNA polymerase after the initiation phase, while some remain associated for further regulatory roles. The dynamics of these interactions are essential for the timely response of the cell to varied signals.
Additionally, sigma proteins can influence the recruitment of other transcription factors and co-regulators, thereby enhancing or inhibiting the transcriptional activity of RNA polymerase. This interplay is a hallmark of how gene regulation is intricately controlled in biological systems.
In summary, sigma proteins serve as critical players in transcription initiation, regulatory functions, and their interactions with RNA polymerase, shaping the landscape of gene expression in diverse biological contexts.
Understanding these roles provides valuable insights into the molecular underpinnings of gene regulation, with implications for both basic research and clinical applications.
Sigma Proteins in Gene Regulation
Gene regulation is a complex and vital component of cellular function, impacting how genes are expressed in response to different environmental conditions. Sigma proteins play a significant role in this regulatory framework. They serve as key components in the initiation of transcription, guiding RNA polymerase to specific promoters, which are the regions of DNA where transcription begins. This capability highlights the importance of sigma proteins in the precise and timely expression of genes.
Mechanisms of Regulation
The mechanisms through which sigma proteins exert their regulatory effects are multifaceted. Generally, they recognize and bind to specific DNA sequences called promoter elements. This binding enables the recruitment of RNA polymerase, leading to the formation of the transcriptional complex. The ability to distinguish between various promoters is particularly crucial for cellular adaptability, allowing organisms to respond efficiently to environmental changes.
Additionally, the regulation by sigma proteins can be modulated by alternative sigma factors. These factors can compete for binding to the same core RNA polymerase, thus influencing which genes are transcribed at a given moment. This competition allows for intricate control over gene expression. Moreover, some sigma factors are activated in response to specific signals, such as stress conditions, further illustrating their role in environmental adaptation.
"The reliance on sigma factors for transcription initiation underscores the complexity of gene regulation processes in living cells."
These mechanisms significantly influence cellular behaviors, such as development, differentiation, and emergency responses. From the perspective of evolution, the diversity in sigma factors across species presents a fascinating area of study. This diversity can be traced back to gene duplication events and provides insight into how organisms can evolve different regulatory strategies.
Role in Stress Responses
Stress responses in organisms are critical for survival. Sigma proteins are notably involved in mediating these responses. Under conditions of stress, such as heat shock or nutrient deprivation, specific sigma factors become active to ensure the transcription of genes that help the cell cope with these unfavorable conditions.
For example, in Escherichia coli, the sigma factor Ο^32 is activated under heat stress to induce genes associated with heat-shock proteins. These proteins assist in protein folding and repair, protecting the cell from damage. Similarly, in other organisms, distinct sigma factors activate stress-responsive genes that help promote survival under extreme conditions.
Furthermore, the interplay between sigma proteins and other cellular signaling pathways adds another layer of complexity. For instance, sigma factors can be influenced by signaling molecules that signal environmental conditions, causing changes in gene expression according to the situation.
Understanding the role of sigma proteins in gene regulation and stress responses is essential for both basic and applied sciences. This knowledge can offer insights into how cells maintain homeostasis, adapt to new challenges, and evolve.
In summary, the role of sigma proteins in gene regulation goes beyond mere transcription initiation; it encompasses a spectrum of mechanisms and interactions that are fundamental to cellular function and adaptability.
Sigma Proteins Across Different Organisms
Sigma proteins play a crucial role across various biological kingdoms, impacting gene expression and cellular stress responses. Understanding the diversity of sigma proteins in both prokaryotic and eukaryotic organisms reveals the evolutionary importance and functional flexibility of these proteins. They act not just as transcription factors but also as agents of adaptation to environmental changes. This section focuses on key differences and similarities in sigma proteins across various organisms, providing a holistic view of their biological significance.
Prokaryotic Sigma Factors
In prokaryotes, sigma factors are integral to the process of transcription initiation. These proteins guide RNA Polymerase to specific promoter regions of the DNA. The most well-known sigma factor in bacteria is sigma 70, which is involved in the transcription of housekeeping genes.
Key characteristics of prokaryotic sigma factors include:
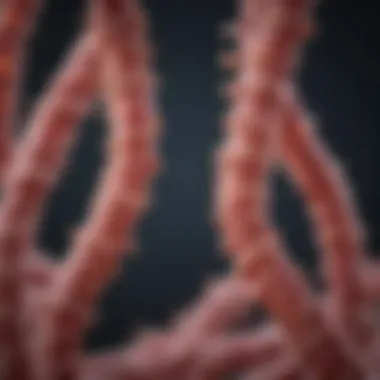
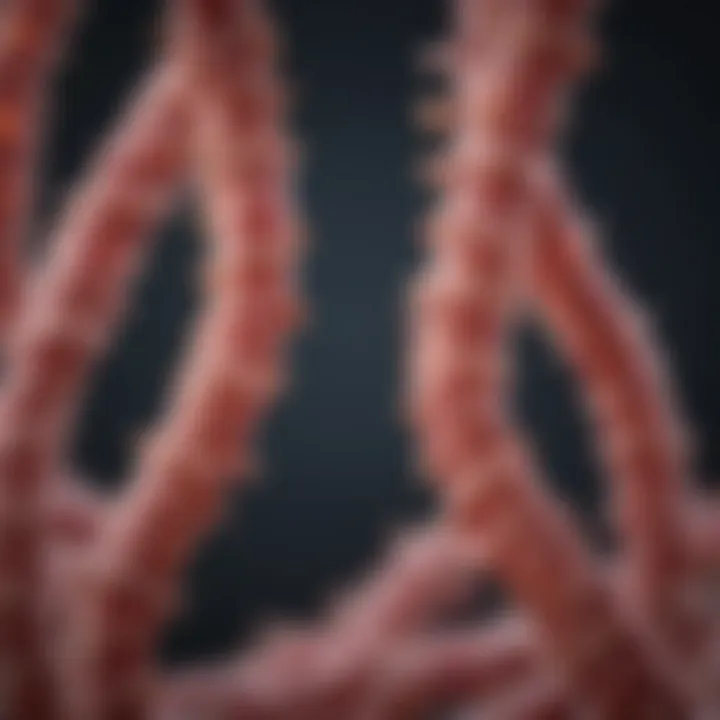
- Diversity: Bacteria can express multiple sigma factors, each recognizing different promoters. This provides adaptability in transcription according to environmental changes.
- Interaction with Core RNA Polymerase: Sigma factors associate with the core RNA polymerase, forming the holoenzyme necessary for transcription.
- Regulatory Roles: Some sigma factors are specifically induced under stress conditions, such as RpoS, which responds to stationary phase and stress treatment including heat and starvation.
These features underline the importance of sigma factors in maintaining cellular integrity and responding to fluctuations in environmental conditions in prokaryotes.
Eukaryotic Variants
Eukaryotic sigma proteins show greater complexity compared to their prokaryotic counterparts. In eukaryotes, multiple RNA polymerases exist, each with unique sigma factors:
- RNA Polymerase I: Responsible for synthesizing rRNA.
- RNA Polymerase II: Synthesizes mRNA and some snRNA.
- RNA Polymerase III: Produces tRNA and other small RNAs.
Eukaryotic sigma factors also possess distinct domains important for the recruitment of transcriptional machinery. Their interactions are highly regulated allowing greater flexibility and specificity in gene expression.
Notable points about eukaryotic sigma proteins include:
- Complex Regulation: They are involved in complex regulatory mechanisms that include enhancers and silencers, enabling precise control over gene transcription.
- Functional Diversification: Eukaryotic sigma factors often have multiple isoforms, adapted to different tissues and developmental stages.
This complexity allows eukaryotic organisms to respond not only to internal cues but also to external signals in a more nuanced way than prokaryotic systems.
Comparison Between Species
The divergence of sigma proteins reflects evolutionary adaptations within various species. Comparing prokaryotic and eukaryotic sigma proteins reveals both conserved and unique features.
Some key differences are:
- Number of Sigma Factors: Prokaryotes typically have fewer sigma factors than eukaryotes, illustrating simpler transcriptional regulation early in evolution.
- Mechanistic Complexity: Eukaryotic sigma proteins integrate with a wider array of regulatory elements, allowing more intricate control over gene expression.
Key similarities include:
- Role in Transcription: Both types of sigma factors are essential for the initiation of transcription.
- Responsiveness to Stress: Both can modify expression patterns in response to stress, demonstrating an element of evolutionary conservation.
Understanding these comparisons fosters insight into not only the functional roles sigma proteins play in living organisms but also into the broader principles of molecular biology and evolutionary adaptation. This helps underline the critical role sigma proteins have played in shaping the genetic response mechanisms across life forms.
Clinical Relevance of Sigma Proteins
Sigma proteins play a critical role in biological processes, and their relevance extends into clinical settings as well. Understanding how these proteins function can pave the way for advancements in disease treatment and the development of therapeutic strategies. As researchers continue to uncover the intricate ways in which sigma proteins interact with cellular machinery, their potential implications in health and disease become more evident.
Role in Disease Mechanisms
Sigma proteins are integral to various cellular processes, and their dysregulation can lead to several diseases. For instance, certain sigma factors have been implicated in cancer progression. Alterations in sigma protein expression can disrupt gene expression patterns, contributing to tumorigenesis. This correlation suggests that studying sigma proteins could reveal biomarkers for early detection of cancers.
Additionally, sigma proteins also have a substantial role in bacterial resistance. Understanding how prokaryotic sigma factors help bacteria survive under stress provides insights into infectious diseases. The ability of these proteins to adapt under hostile conditions can inform the development of new antibiotics targeting sigma factors, allowing for more effective treatments against resistant bacterial strains.
Examples of diseases related to sigma protein malfunction include:
- Cancer: Altered sigma factor levels contribute to tumor growth.
- Infectious diseases: Bacteria utilizing sigma factors for stress resistance.
"The study of sigma proteins is essential not only for understanding basic biology but also for developing new avenues for treatment of various diseases."
Thus, addressing the mechanisms involving sigma proteins could provide new understandings of pathophysiology in several conditions, enhancing both diagnostics and treatment.
Potential Therapeutic Targets
The distinct roles of sigma proteins in a range of biological processes highlight their potential as therapeutic targets. If researchers can manipulate sigma factors effectively, it may be possible to alter disease outcomes. For instance, selectively inhibiting specific sigma factors associated with cancer could reduce tumor growth or even promote cancer cell death.
Moreover, the unique structural aspects of sigma proteins make them an attractive target for drug design. By focusing on the points of interaction between sigma factors and RNA polymerase, scientists can develop small molecules that modulate activity without disrupting essential cellular functions.
Therapeutic strategies involving sigma proteins could include:
- Inhibition: Reducing the activity of sigma factors in cancer cells.
- Activation: Enhancing sigma factor function to improve antibacterial responses.
Approaching treatment from the angle of sigma protein manipulation opens new pathways for research and therapeutic development. The integration of sigma proteins in clinical research could reshape our understanding of disease and enhance intervention techniques.
Techniques in Sigma Protein Research
Understanding sigma proteins requires sophisticated techniques that enable researchers to investigate their structure and function. These methods not only provide insight into the biological roles of sigma proteins but also facilitate the development of therapeutic approaches targeting these proteins in various diseases. As the field evolves, staying current with advancements in methodologies is essential. This section discusses critical techniques in sigma protein research, their benefits, and key considerations.
Molecular Cloning Methods
Molecular cloning is a fundamental technique used in sigma protein research. This method involves isolating a specific gene encoding a sigma protein and inserting it into a vector, which can then replicate inside a host organism. The cloning of sigma genes allows researchers to study their expression and function in a controlled environment.
The basic steps involved in molecular cloning include:
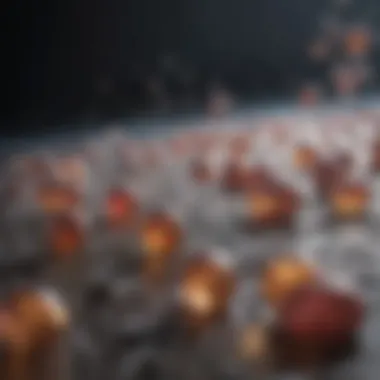
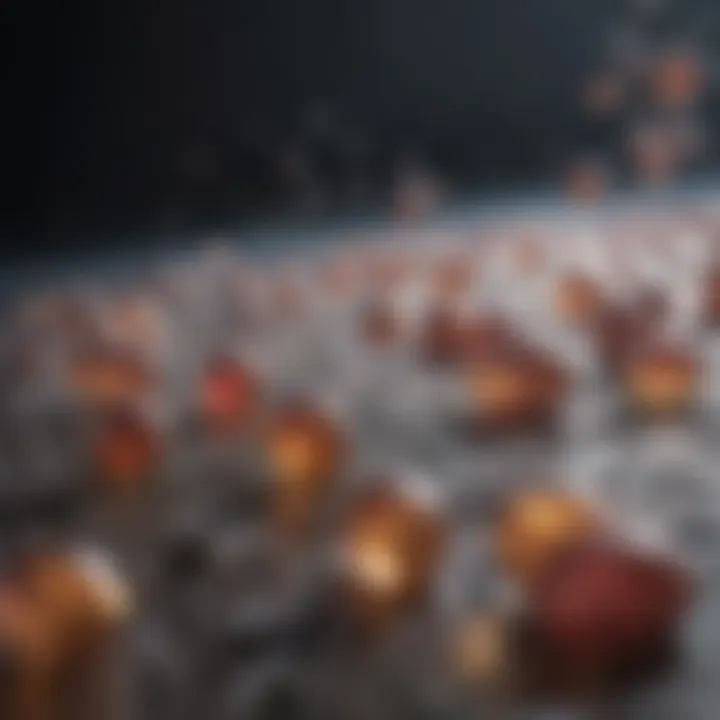
- Isolation of DNA: This step involves extracting DNA from an organism or a plasmid.
- Cutting DNA: Restriction enzymes are used to cut DNA at specific sequences, creating fragments that can be ligated.
- Ligation: The desired sigma gene is ligated into a vector, forming a recombinant DNA molecule.
- Transformation: The recombinant vector is introduced into a host cell, such as Escherichia coli, allowing for replication.
This technique is essential in producing large quantities of sigma proteins for further analysis. The ability to create specific alterations in the sigma gene also opens avenues for studying the effects of mutations.
Protein Expression and Purification
After cloning sigma genes, the next essential step is to produce and purify the proteins they encode. Proper expression systems must be chosen based on compatibility and yield requirements, which may involve E. coli, yeast, or mammalian cells.
Key considerations for effective protein expression include:
- Choice of Expression System: Each system has advantages and limitations concerning post-translational modifications and expression levels. For instance, E. coli is often used for high-yield production due to its rapid growth.
- Optimization of Conditions: Factors such as temperature, pH, and nutrient availability can significantly influence protein yield and quality. Careful adjustment of these parameters is necessary for optimal expression.
- Purification Techniques: Once sigma proteins are expressed, purification is critical to obtain homogenous protein samples for functional studies. Techniques such as affinity chromatography are commonly utilized to isolate proteins based on specific tags or properties.
Proper expression and purification processes are vital to obtaining functional sigma proteins for structural and functional assays.
"Thorough techniques in sigma protein research are the backbone of innovations in understanding their roles in biological systems."
Combining these molecular cloning methods with advanced expression and purification techniques paves the way for further discoveries. This seamless integration of techniques is crucial for gaining deeper insights into sigma proteins, paving the path for their potential roles in therapeutic applications.
Current Trends in Sigma Protein Studies
Current trends in sigma protein research delve into various innovative methodologies and multidisciplinary approaches to explore this critical component of molecular biology. As our understanding of sigma proteins evolves, researchers are focusing on their intricate roles in cellular processes. The significance of these trends extends beyond mere academic interest; they are reshaping therapeutic strategies and driving technological advancements in research.
Emerging Research Areas
There is a surge of interest in particular research domains such as synthetic biology and systems biology, which emphasize creating new biological functions through manipulation of sigma proteins. Researchers are investigating sigma factorsβ roles in the context of synthetic circuits. This area aims to design living cells with specific and programmable functions, harnessing sigma proteins to achieve precise control over gene expression.
In addition, the study of tau protein and its relation to sigma proteins opens new possibilities in understanding neurodegenerative diseases. The interactions between sigma factors and alpha-synuclein are being highlighted to comprehend their impacts on cellular health. Indeed, this subclass of sigma proteins may play a pivotal role in combating diseases like Parkinson's, providing insight into novel therapeutic targets.
Emerging literature also showcases advances in ligand-based approaches. Recent developments involve the identification of small molecules that can selectively modulate sigma factors, thus influencing their regulatory activity. This allows for targeted modulation and presents an avenue for drug discovery, particularly for conditions linked to sigma protein dysregulation.
Interdisciplinary Approaches
Interdisciplinary collaboration is becoming essential in sigma protein studies, merging fields such as biochemistry, bioinformatics, and structural biology. By adopting an integrative scientific framework, researchers can leverage advanced computational techniques alongside experimental methods. This synergy enables the modeling of complex sigma protein interactions within various cellular environments.
For instance, implementing bioinformatics tools contributes to the identification of conserved domains among sigma proteins across species. This comparative analysis enhances our understanding of functional conservation and divergence. The cross-pollination of ideas among scientists from diverse disciplines reinforces the complexity and multifunctionality of sigma proteins.
Moreover, collaboration with engineering disciplines leads to novel biophysical methods for studying protein dynamics. Techniques such as single-molecule imaging and mass spectrometry are now routinely deployed to observe sigma proteins in real-time, allowing for deeper insights into their conformational states and functional mechanisms.
Overall, current trends in sigma protein studies reflect a broader commitment to interdisciplinary research and innovative methodologies. This holistic approach paves the way for significant advancements in our understanding of sigma proteins and their implications in health and disease.
Future Directions in Sigma Protein Research
The exploration of sigma proteins represents a dynamic and rapidly evolving field. Future directions in sigma protein research hold significant importance not only for molecular biology but also for therapeutic applications. Understanding the nuanced roles these proteins play can lead to more effective interventions in disease contexts. Advanced research could illuminate previously unidentified functions of sigma proteins, contributing to both fundamental biology and practical applications.
Potential Innovations
As research progresses, several potential innovations are on the horizon that could change how sigma proteins are perceived and utilized. These innovations are interconnected with advancements in technology and molecular biology. For instance:
- CRISPR Technology: The integration of CRISPR can enhance gene editing techniques specific to sigma protein coding sequences. This allows for precise modifications that can help to study the functions of specific sigma variants in various organisms.
- High-Throughput Screening: Developing high-throughput methods to analyze sigma protein interactions could facilitate the identification of novel modulators. This approach could revolutionize how researchers understand regulatory networks in both prokaryotes and eukaryotes.
- Synthetic Biology: Advances in synthetic biology might allow for the engineering of sigma proteins with novel functionalities. Tailoring these proteins can lead to unique applications in biosensors and metabolic engineering.
These innovations will pave the way for deeper insights and applications, which could establish a new paradigm in the research of sigma proteins.
Challenges and Limitations
Despite the promising future of sigma protein research, researchers face several challenges and limitations that merit careful consideration. The complexity of sigma protein interactions and their roles in various cellular contexts introduces several obstacles:
- Assay Development: Creating robust assays for studying sigma proteins is critical. Many protein-protein interactions are transient, making detection difficult. This can impact the data obtained during experiments.
- Functional Diversity: Sigma proteins display a high degree of functional diversity across different species. Understanding how these proteins evolve and function in specific contexts can be complicated.
- Lack of Standardized Protocols: The absence of standardized protocols in sigma protein studies can lead to variability in results. This variability complicates the comparison of findings across different studies.
Despite these challenges, the ongoing efforts in sigma protein research continue to uncover valuable insights, supporting the notion that perseverance will yield significant rewards in understanding these crucial molecular players.
Closure
The conclusion serves as a pivotal summation of the insights gained through this article. It encapsulates the multifaceted roles of protein sigma and reflects on its structural, functional, and regulatory aspects across diverse biological systems. Each section detailed the importance of sigma proteins, exposing their intricate involvement in transcription initiation, stress responses, and gene regulation.
Summary of Findings
The exploration of sigma proteins revealed critical findings about their roles in various organisms. Key points include:
- Structural Characteristics: Sigma proteins exhibit a unique structural design that varies among different species and types. This variability plays a crucial role in their functions, particularly in transcriptional regulation.
- Functional Roles: They are essential for the initiation of transcription in prokaryotes and eukaryotes, and they regulate responses to environmental stressors, enabling organisms to adapt and survive.
- Clinical Relevance: Sigma proteins are implicated in disease mechanisms, making them potential targets for therapeutic innovation.
This synthesis demonstrates the vital nature of sigma proteins within the molecular landscape and underscores their relevance in health and disease.
Significance of Future Research
Future research on sigma proteins is paramount for several reasons:
- Therapeutic Development: Understanding the nuances of how sigma proteins function can lead to novel treatment methods for various diseases, particularly those with genetic components. Improved knowledge can inspire the design of drugs that target specific sigma factors.
- Biotechnological Applications: Advancements in sigma protein research could bolster biotechnology, enhancing processes such as gene therapy, where precise control over gene expression is necessary.
- Interdisciplinary Collaboration: As research continues, collaboration across disciplines will further deepen our understanding and could lead to unexpected discoveries that advance both academic knowledge and practical applications.
In summary, ongoing exploration into sigma proteins not only sheds light on fundamental biological processes but also opens pathways for transformative scientific and medical advancements.