Exploring Ion Semi-Permeable Membranes: Mechanisms & Uses
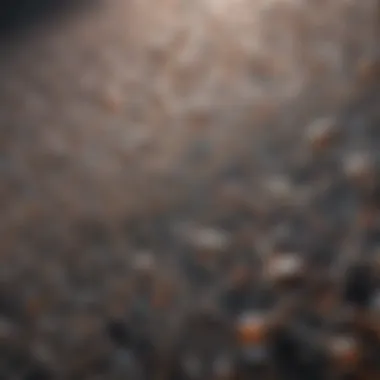
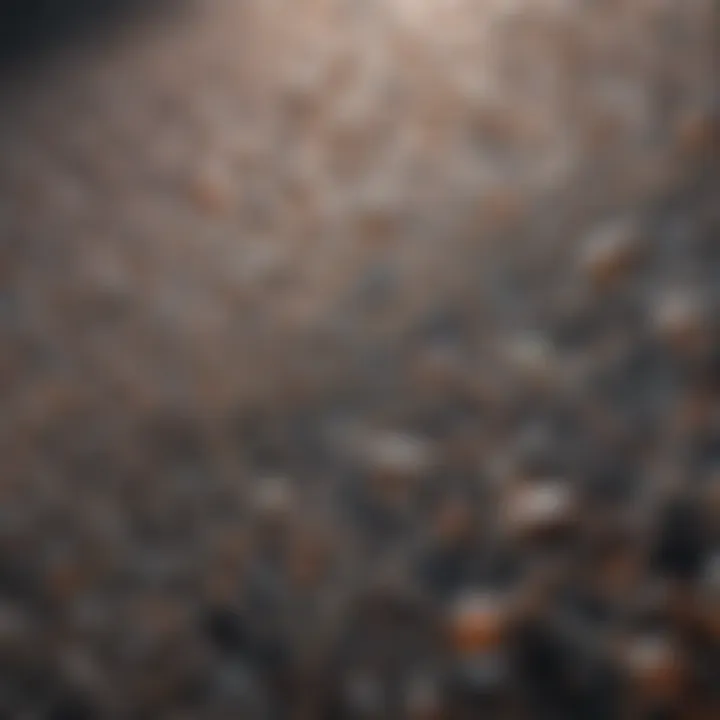
Intro
In the intricate world of materials science and biophysics, ion semi-permeable membranes play a pivotal role. These membranes are not just structures; they are gateways that facilitate the controlled passage of ions, fostering essential interactions between different environments. In essence, they allow certain ions to traverse while blocking others, creating an optimal balance necessary for various biological and industrial processes.
The significance of understanding such membranes lies in their versatility. They are ubiquitous in industries ranging from pharmaceuticals to wastewater treatment, and they also mirror crucial functions in nature, like the way our cells control their internal environments. With the ongoing advancement in membrane technology, the quest becomes clearer: how can we refine these materials to meet the demands of contemporary challenges?
This article aims to peel back the layers of ion semi-permeable membranes, focusing on their mechanisms, structural features, and an array of applications. We will explore the principles governing ion movement, delve into recent innovations, and contemplate future prospects in membrane research. As we embark on this journey, one can appreciate the myriad ways these membranes impact scientific progress and everyday life.
Methodology
Study Design
The approach to studying ion semi-permeable membranes involves a blend of experimental and theoretical frameworks. By employing advanced characterization techniques such as electron microscopy and spectroscopy, researchers can gain insights into membrane morphology and composition. Experiments designed to evaluate ion transport dynamics further elucidate the mechanisms at play.
Data Collection Techniques
Quantitative data regarding ion permeation can be obtained through several methods:
- Conductometric measurement: This technique evaluates changes in electrical conductivity as ions pass through the membrane, enabling researchers to gauge permeation rates.
- Nuclear magnetic resonance (NMR): Utilized to observe the behavior of ions within the membrane's structure, offering a molecular perspective.
- Computer simulations: Modeling and simulations using software like LAMMPS provide a virtual exploration of how ions interact with membrane surfaces under various conditions.
Discussion
Interpretation of Results
The data derived from these methodologies often reveal intricate details of ion behavior and membrane integrity. For instance, the selectivity of a membrane can significantly affect its efficiency in real-world applications. A thorough understanding allows for optimization in particular settings, such as in drug delivery systems, where controlled release of therapeutics is paramount.
Limitations of the Study
While advancements in membrane research are promising, certain limitations persist. Sample variability, environmental influences, and the scale of study can affect reproducibility. Additionally, many studies may focus on ideal conditions that don't represent real-world scenarios, leading to challenges in practical implementations.
Future Research Directions
Moving forward, researchers are called to expand their inquiry into hybrid membranes that combine various materials, or those with enhanced selectivity for specific ions. The development of smart membranes capable of adapting to changing conditions presents a fresh frontier. Furthermore, addressing issues related to membrane fouling and longevity remains crucial as industries depend increasingly on these materials to operate efficiently.
The quest for innovation in membrane technology often mirrors the broader challenges in science: navigating through complexities to unearth solutions that are sustainable and effective.
Intro to Ion Semi-Permeable Membranes
Ion semi-permeable membranes play a crucial role in various scientific and industrial applications, acting as selective barriers that allow specific ions to pass while blocking others. This selective permeability is vital for maintaining the integrity of biological systems, facilitating chemical processes, and addressing environmental challenges. Understanding the mechanisms that underpin these membranes can unlock new approaches in technology, improve water purification methods, and enhance medical therapies.
Definition and Concept
At its core, a semi-permeable membrane is a barrier that permits the passage of certain ions while restricting others. This selective property is determined by multiple factors, including the membrane's structure, charge, and size. For instance, consider the role of a cell membrane: it dictates which substances can enter or exit the cell, thus maintaining homeostasis. The term "semi-permeable" suggests that while the membrane allows the flow of some ions through it, others are, in effect, filtered out. This is essential in processes such as osmosis and diffusion, where substances move according to their concentration gradients.
The concept of semi-permeability evolves with advancements in materials science. With the introduction of synthetic membranes designed for specific applications, the reliance on natural membranes has decreased. These new materials can be engineered to mimic or enhance the properties of biological membranes while enabling more precise control over ion transport. In applications ranging from desalination to drug delivery, the precise understanding of this semi-permeable nature can significantly improve efficiency and effectiveness.
Historical Context
The journey into understanding ion semi-permeable membranes can be traced back hundreds of years. The initial curiosity can be linked to the study of osmosis, with notable contributions from scientists like Aristotle, who pondered the movement of water in plants. However, a major leap occurred in the late 19th century when the groundwork for membrane technology began. Researchers like Thomas Graham introduced concepts of diffusion, laying the groundwork for future explorations.
In the 20th century, the development of synthetic membranes marked a pivotal moment. With breakthroughs in polymer science, such as the creation of polysulfone and polyamide membranes, applications expanded rapidly. Their utilization in reverse osmosis and wastewater treatment systems revolutionized the way societies manage resources and waste.
"The understandings and technologies built around ion semi-permeable membranes have had profound impacts on environmental sustainability and health."
As we continue to innovate and refine these materials, the historical context provides a scroll of insights, underscoring both the scientific maturation and the pressing need for smart technologies in addressing modern challenges, such as water scarcity and energy production. By delving into our past, we also illuminate the pathways forward in enhancing these membranes for the future.
Fundamental Principles of Ion Semi-Permeability
The topic of ion semi-permeability is crucial in understanding how membranes function in various contexts—from biological systems to industrial applications. By evaluating the fundamental principles underlying ion transport, researchers and practitioners can appreciate how different materials can selectively allow or block ions. This selective permeability governs vital processes such as nutrient uptake in cells, water purification methods, and even energy generation.
Structure of Semi-Permeable Membranes
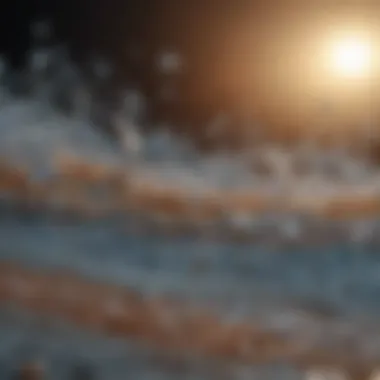
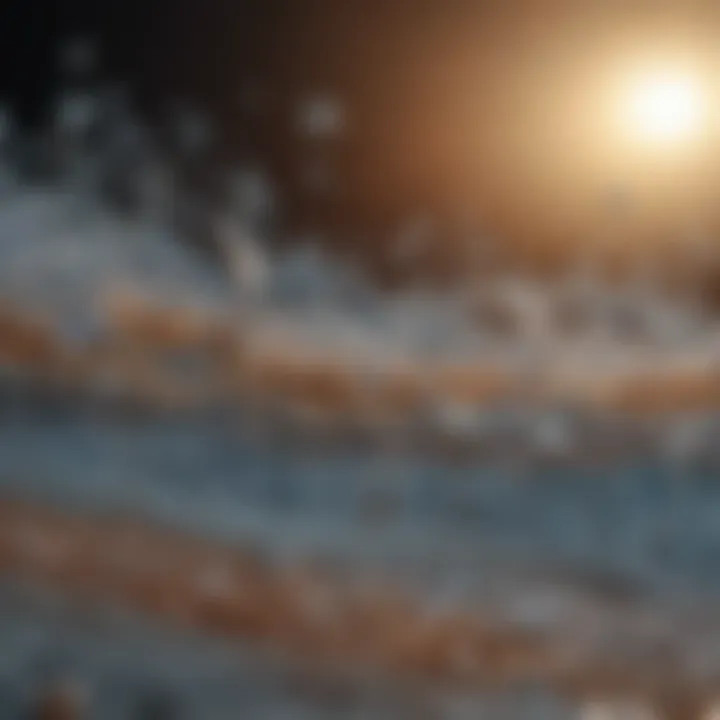
The structure of semi-permeable membranes is foundational to their function. These membranes typically consist of a lipid bilayer or a polymeric matrix that exhibits selective permeability to ions. For example, biological membranes are often composed of phospholipids, which form a barrier that allows certain ions to pass while restricting others based on size and charge.
Key aspects of the structure include:
- Hydrophobic core: The core of the membrane repels water-soluble ions, making it necessary for ions to pass through specific channels or transport proteins.
- Protein channels: Integral proteins embedded in the membrane facilitate the transport of ions. These can be selective for particular ions like sodium or potassium, critical for cellular activities.
- Surface charge: The membrane's surface can carry a net charge, influencing the electrostatic forces at play during ion transport.
This structural intricacy allows for a finely-tuned system where the movement of ions is both regulated and efficient, reflecting the evolutionary adaptations seen in nature.
Mechanisms of Ion Transport
Understanding how ions traverse these membranes is essential for fields ranging from biology to engineering. There are mainly two mechanisms involved: passive transport and active transport.
- Passive Transport: This process relies on diffusion, where ions move along their concentration gradient without the need for energy. An example is potassium ions moving out of a cell to maintain charge balance.
- Active Transport: Unlike passive transport, active transport requires energy, often from ATP, to move ions against their concentration gradient. For instance, sodium-potassium pumps are vital for regulating intracellular conditions in neurons and muscle cells.
In both cases, the effectiveness depends on membrane composition and the presence of specific proteins, making the understanding of these mechanisms not just an academic exercise but an essential part of technology development as well.
Factors Influencing Permeability
Several factors can influence how permeable a membrane is to different ions:
- Ionic size and charge: Larger ions or those with multiple charges may find it harder to penetrate the membrane compared to smaller, neutral ions.
- Temperature: Increasing temperature generally enhances permeability, as higher kinetic energy allows more ions to traverse the membrane.
- Membrane composition: Certain materials have properties that either enhance or inhibit ion flow. For instance, polymer-based membranes can be engineered to optimize flux rates for specific applications in industry.
Understanding these factors helps in designing better membranes for specific applications, whether for desalination plants, fuel cells, or biomedical devices.
"The careful design of membrane materials can lead to breakthroughs in areas like water treatment and energy efficiency, showcasing the importance of understanding ion semi-permeability principles."
In summary, grasping the fundamental principles of ion semi-permeability is more than mere academic inquiry; it's a gateway to innovations that can address some of the most pressing challenges in our society today.
Types of Ion Semi-Permeable Membranes
The study of ion semi-permeable membranes plays a crucial role in various fields such as biology, medicine, and engineering. These membranes are critical components that facilitate the selective transport of ions and molecules across barriers, thereby influencing numerous processes. When we categorize these membranes into distinct types—natural, synthetic, and composite—we can understand better their unique properties, applications, and challenges. Each type offers its own set of benefits and considerations, impacting how they are utilized in both practical and theoretical contexts.
Natural Membranes
Natural membranes are the building blocks of life, found in all living organisms. The most renowned example is the cell membrane, often described using the fluid mosaic model. These membranes are composed of lipid bilayers with embedded proteins, allowing them to control the passage of ions and nutrients.
Some key characteristics and significance of natural membranes include:
- Selectivity: They allow certain ions to pass while blocking others, ensuring cellular integrity.
- Dynamic Nature: Natural membranes can change their composition in response to environmental cues, aiding in processes like cellular signaling.
- Biocompatibility: These membranes are essential for maintaining homeostasis and supporting cellular functions.
Natural membranes are also pivotal in biological processes such as osmosis and diffusion. For instance, the selective permeability of the cell membrane helps in maintaining the essential concentration gradients of ions like sodium and potassium, which are critical for nerve impulse transmission and muscle contraction.
Synthetic Membranes
In contrast, synthetic membranes are man-made and designed to replicate or enhance the functions of natural membranes. These membranes are often created from polymers and can be tailored for specific applications, notably in filtration and separation processes.
Advantages of synthetic membranes include:
- Versatility: They can be engineered to possess particular properties, making them suitable for diverse applications, from desalination to medical devices.
- Durability: Synthetic membranes often exhibit longer lifespans and resistance to fouling compared to their natural counterparts.
- Scalability: The manufacturing processes can be scaled to meet the demands of various industries.
Common synthetic membranes include reverse osmosis membranes used in water purification systems and ultra-filtration membranes utilized in food and beverage processing. The design of these membranes allows for controlled permeability, optimizing efficiency in removing unwanted ions or particulates.
Composite Membranes
Composite membranes, as the name suggests, combine elements from both natural and synthetic categories. They typically consist of layers of different materials, which help to achieve desired properties such as increased strength, selectivity, or permeability.
Some notable aspects of composite membranes are:
- Enhanced Performance: By layering various materials, these membranes can outperform single-material membranes in selective ion transport.
- Customization: The combination can be tailored for specific applications, such as energy generation or wastewater treatment, leading to more efficient processes.
- Cost-Effectiveness: Many composite membranes can be produced at a lower cost while still offering high performance.
An example is the development of thin-film composite membranes, where a selective layer is applied to a porous support layer. This design has found applications in both environmental remediation and energy recovery systems.
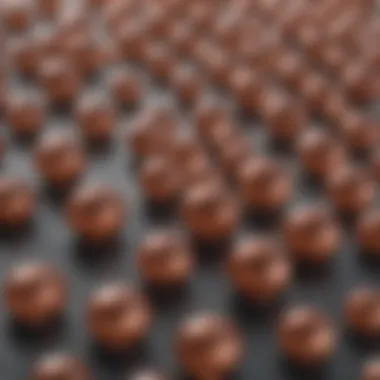
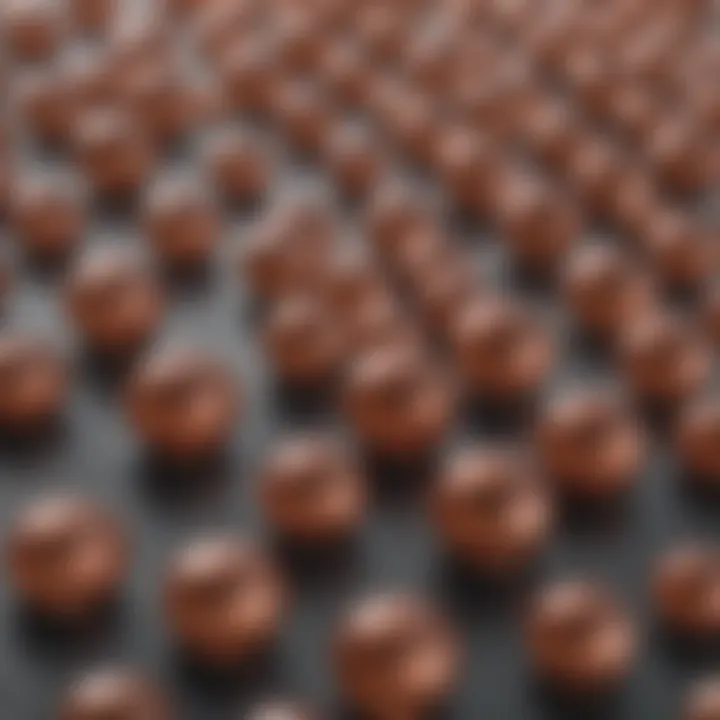
"By understanding the unique advantages and applications of different types of ion semi-permeable membranes, researchers and industry professionals can make informed decisions that lead to innovative solutions in their fields."
Biological Significance of Ion Semi-Permeable Membranes
Ion semi-permeable membranes play a pivotal role in the biological landscape, guiding numerous essential processes that sustain life. These membranes, found within cellular structures, are not mere barriers but are sophisticated interfaces that regulate the movement of ions in and out of cells. The functionality they offer is crucial for maintaining homeostasis, transmitting signals, and conducting vital biochemical reactions. This section delves into the significance of these membranes concerning cellular functions and provides concrete examples that illustrate their critical roles in biological systems.
Role in Cellular Functions
Semi-permeable membranes are the gatekeepers of cellular dynamics. They enable selective permeability, allowing specific ions to enter or exit the cell based on concentration gradients and electrical charges. This selectivity aids in maintaining osmotic balance and is vital for:
- Nutrient Uptake: Essential ions like sodium and potassium are absorbed, facilitating key metabolic reactions and energy production.
- Signal Transduction: Ion channels in membranes are crucial for the perception of environmental signals, ultimately leading to cellular responses. For instance, calcium ions play various roles in processes ranging from muscle contraction to hormone release.
- Membrane Potential: The differential concentration of ions across membranes generates electrical potentials that are essential for nerve impulse transmission and muscle contraction. This phenomenon is a foundation for neuronal communication and heart function.
In broader terms, disruptions in the functioning of semi-permeable membranes can lead to disorders. For example, over-activity of ion channels might result in conditions like epilepsy, where nerve cells fire excessively. Clearly, the biological significance of these membranes is not limited to structural aspects; they are fundamentally intertwined with the very workings of life itself.
Examples from Biological Systems
The biological world is rich with examples that showcase the significance of ion semi-permeable membranes. Some notable instances include:
- Neurons: In the nervous system, the movement of ions such as sodium, potassium, calcium, and chloride across neuronal membranes is how signals are propagated along nerves. The action potential, which underlies the rapid communication in the brain, is chiefly driven by the opening and closing of ion channels.
- Synapses: When neurons communicate at synapses, neurotransmitters are released in response to ion influxes, an essential process for signal relay.
- Muscle Cells: The contraction of muscles is driven by the movement of calcium ions into muscle cells, triggering a cascade that ultimately leads to muscle contraction. Dysfunctional ion channels in muscle cells can lead to conditions like myotonia.
- Kidneys: In the renal system, maintaining body homeostasis relies heavily on the selective reabsorption of ions like sodium and chloride in nephron membranes. This balances water retention and electrolyte levels, which are crucial for overall bodily function.
"Without those semi-permeable membranes, the complex dance of ions would falter, leading to chaos within the cellular machinery."
- Plant Cells: Ion semi-permeable membranes are also crucial in plants, where they manage the uptake of essential nutrients like potassium for processes such as photosynthesis and growth. Their regulation allows plants to respond dynamically to their environments, maintaining stability.
Understanding the biological significance of ion semi-permeable membranes gives insight into their roles in health and disease. The interplay between these membranes and cellular functions reveals how life, in all its complexity, hinges on the selective movement of ions.
Applications in Environmental Science
The realm of environmental science has benefited significantly from the study and usage of ion semi-permeable membranes. These specialized structures play a key role in addressing pressing challenges related to water quality and resource management. The intricacies of these membranes allow for selective permeability, enabling the removal of specific ions or contaminants while allowing clean water to flow through. This capability is crucial in our efforts to ensure a sustainable, plentiful water supply.
Water Purification Techniques
Water purification is one of the most vital applications of ion semi-permeable membranes. These membranes are utilized in various techniques, such as reverse osmosis, ultrafiltration, and nanofiltration.
- Reverse Osmosis (RO): In this process, water is forced through a semi-permeable membrane under high pressure. The membrane acts as a barrier, removing dissolved salts and impurities. This method is particularly effective for desalting seawater and producing clean drinking water.
- Ultrafiltration (UF): Here, the membrane pores are larger compared to RO. It's designed to block larger molecules, bacteria, and suspended solids while allowing smaller ions and water to pass. This technique is useful in ensuring that water is free from contaminants without removing essential minerals.
- Nanofiltration: This method applies to the filtration of substances in the 1 nanometer range and is capable of rejecting divalent ions while letting monovalent ions pass. It strikes a balance between the efficiency of reverse osmosis and the less stringent requirements of ultrafiltration.
Each of these methods demonstrates the versatility and effectiveness of ion semi-permeable membranes in improving water quality. As public awareness grows regarding environmental issues, the demand for such techniques will likely remain high.
Role in Wastewater Treatment
In wastewater treatment, ion semi-permeable membranes contribute significantly to minimizing environmental impact while recycling valuable resources. The characteristics of these membranes allow for selective removal of contaminants, making them indispensable in modern treatment processes.
- Membrane Bioreactors (MBR): This technology integrates biological treatment with membrane filtration, effectively removing pathogens and large organic molecules. The result is treated water that can often be reused for irrigation or industrial processes.
- Electrodialysis: In this process, membranes are used to separate ions in wastewater in response to an electric field. This results in the desalination of waste streams and recovery of valuable minerals, promoting resource recovery in addition to pollution control.
- Foam Fractionation: This technique employs ion-selective membranes to concentrate dissolved organic substances, improving the quality of treated water and enabling the recycling of valuable materials.
Overall, ion semi-permeable membranes not only help in treating wastewater but also foster a sustainable approach by recovering resources and reducing the overall environmental footprint.
Industrial and Technological Advances
Advancements in industrial and technological applications of ion semi-permeable membranes have opened the door to a plethora of new possibilities. These membranes are not only pivotal in separating substances but also play a vital role in various processes that support modern-day industries. Their importance lies primarily in their ability to selectively allow the passage of ions while filtering out unwanted substances. This selective permeability is a game changer, especially when it comes to efficiency and sustainability in industrial processes.
In sectors ranging from water purification to pharmaceuticals, semi-permeable membranes enhance efficiency and reduce costs. These innovations significantly improve processes, driving better outcomes in product quality and environmental sustainability. Manufacturers have increasingly looked to these technological innovations, as they see potential benefits in reducing waste and improving resource utilization.
For example, in the chemical industry, membrane separation processes significantly cut energy consumption compared to conventional methods, such as distillation or evaporation. This not only lessens the environmental footprint but also reduces overall operational costs. Moreover, the adaptability of these membranes means that they can be tailored to meet specific industry needs, thereby fostering innovation and development in various technologies.
Membrane Separation Processes
The term membrane separation processes encompasses a range of techniques that utilize ion semi-permeable membranes. These processes are commonly employed in various industries, including food and beverage, pharmaceuticals, and environmental science. Essentially, they function based on the size and charge of different ions, allowing for selective separation.
- Reverse Osmosis: This widely used technology employs semi-permeable membranes to remove ions and contaminants from water. It has a significant role in desalination, enabling access to fresh water from saline sources.
- Ultrafiltration: This process uses membranes that reject larger solutes while allowing smaller ions and molecules to pass through, making it useful in dairy and juice industries for the concentration of products while maintaining quality.
The efficiency of these methods hinges on the membrane's characteristics, such as pore size and surface chemistry. Innovations in materials science lead to the development of membranes with enhanced performance, allowing industries to cater to more complex requirements.
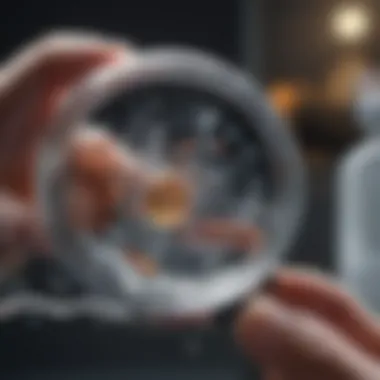
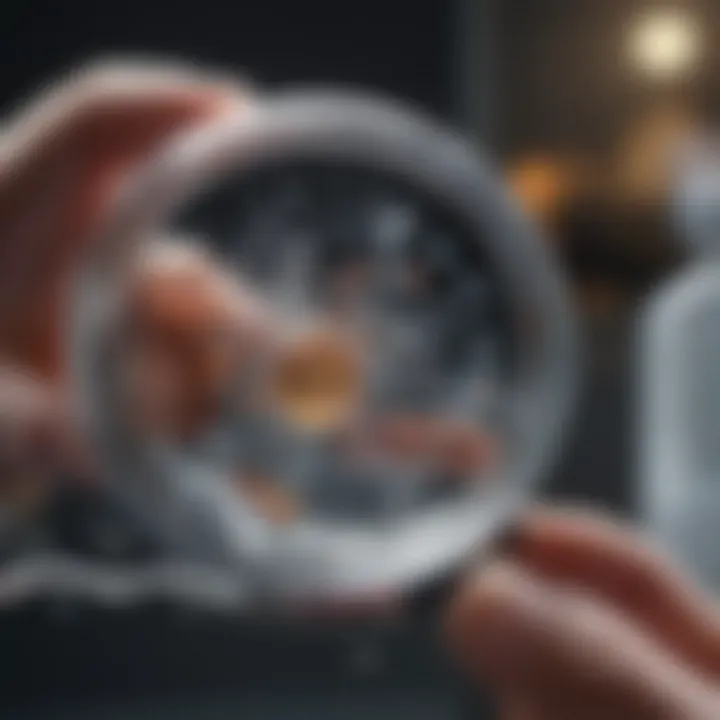
Energy Generation from Ion Transport
An exciting avenue within industrial applications is the energy generation potential of ion transport via semi-permeable membranes. This aspect of ion semi-permeability holds promise for sustainable energy solutions. When ions move through membranes, they create ion gradients which can be harnessed in various ways.
- Salinity Gradient Power: This concept utilizes the difference in salt concentration between seawater and freshwater to generate energy through a process called osmotic power. It's a remarkable method that takes advantage of naturally occurring phenomena to produce renewable energy.
- Fuel Cells: Membrane technology is also integral to proton exchange membrane fuel cells. These fuel cells convert chemical energy into electricity through the movement of ions across the membrane, showcasing how semi-permeable membranes can be harnessed for clean energy production.
Progress in this area not only boosts energy efficiency but aligns well with global sustainability goals. Investments in research and development are vital to further explore and optimize energy generation processes related to semi-permeable membranes.
Challenges and Limitations
When discussing ion semi-permeable membranes, it's crucial to address the challenges and limitations associated with their use. These hurdles can significantly impact their efficiency, longevity, and applicability across various fields. Understanding these issues not only helps in improving existing technologies but also paves the way for innovative solutions that could optimize membrane performance. Let's delve into the two critical aspects: membrane fouling and durability issues.
Membrane Fouling
Membrane fouling is one of the primary obstacles encountered in the utilization of ion semi-permeable membranes. This phenomenon refers to the accumulation of unwanted materials on the surface or within the pores of the membrane. It can be precipitated by a variety of factors: organic compounds, inorganic particles, and biological organisms can all contribute to this sticky situation.
Fouling does not come without consequences. It can lead to a marked reduction in membrane permeability, creating a bottleneck in processes such as water purification and wastewater treatment. Moreover, it requires increased energy for pumping and additional maintenance interventions, both costly in terms of time and resources. The constant need for cleaning or even membrane replacement poses a significant financial burden on industries relying heavily on these membranes.
Strategies to combat membrane fouling often include the use of various pre-treatment techniques, membrane surface modifications, and the deployment of anti-fouling agents. However, developing a foolproof solution remains tricky, as different applications will face unique fouling pressures based on local conditions and the specific pollutants involved.
"Membrane fouling is like a snowball rolling downhill: it starts small but can grow into a significant problem, impacting performance and costs."
Durability and Longevity Issues
Another major challenge relating to ion semi-permeable membranes is their durability and longevity. Simply put, how long these membranes will last in operational settings can greatly determine their efficiency, cost-effectiveness, and environmental impact. Several factors can influence the lifespan of these membranes, such as the material used, the conditions of the operating environment, and the ion concentration of the solutions they handle.
The continuous stress from chemical agents, temperature fluctuations, and physical wear can result in membrane degradation over time. This degradation is particularly problematic in industrial applications, where a high turnover of membranes can severely increase operational costs and lead to interruptions in production processes.
Finding a balance between permeability, selectivity, and durability is often a tightrope walk for researchers and industry practitioners alike. Innovations in material science have led to the development of membranes with enhanced chemical resistance and mechanical stability, but there's always room for further research. Improvements in this area not only promise prolonged membrane life but can also lead to more sustainable practices, as reduced waste from discarded membranes is increasingly a priority in contemporary industrial practices.
Research Trends and Future Directions
Research in the realm of ion semi-permeable membranes is not a static field; it’s ever-evolving. As technology and scientific understanding advance, new methodologies and innovative materials are emerging, leading to profound shifts in how we perceive and utilize these essential components across various applications. Here, we delve into the nuance of current research trends and speculate on future directions that could revolutionize this field.
Nanotechnology in Membrane Development
Nanotechnology is proving to be a game changer for the development of ion semi-permeable membranes. Researchers are harnessing the unique properties of materials at the nanoscale to enhance the selective permeability of membranes. For instance, by manipulating the surface properties and pore structures at the nanometer level, scientists create membranes that are not only more efficient but also tailor-made for specific applications.
One tangible benefit of this approach is the potential to reduce energy consumption during the filtration process. With traditional membranes, a significant amount of energy is often required to drive ions through. However, nanostructured membranes can minimize this need, providing a more environmentally friendly alternative.
Moreover, functionalized nanoparticles incorporated into these membranes enable enhanced ion selectivity and rejection rates. This ability is critical in processes such as desalination, where maximizing salt rejection while allowing water to pass through is vital. In this way, the integration of nanotechnology isn't merely an advancement; it represents a paradigm shift in how we develop and employ ion semi-permeable membranes.
Future Applications in Medicine
Looking ahead, the integration of ion semi-permeable membranes has promising implications for the medical field. The ability to control ion flow with precision opens a treasure trove of potential applications in drug delivery systems, biosensors, and tissue engineering. Consider drug delivery: by utilizing membranes that selectively permit the passage of certain ions, medical practitioners could control the release rate of therapeutic agents. This could lead to more personalized medicine, targeting specific conditions with unprecedented accuracy.
Additionally, the development of biosensors utilizing ion semi-permeable membranes can enhance our ability to monitor physiological changes in real-time. For example, using thin-film membranes that are sensitive to specific ions could revolutionize how we diagnose conditions or monitor health metrics, providing immediate feedback to both patients and clinicians.
Another exciting prospect involves tissue engineering. Ion semi-permeable membranes can serve as scaffolds for cell growth, providing a conducive environment for tissue regeneration while regulating the ion exchanges essential for cellular activities. This could transform how we approach healing and recovery in a variety of contexts, from organ transplants to wound healing.
"The future of medicine could very well depend on the nuanced understanding and application of ion semi-permeable membranes, opening doors we haven’t even begun to think about yet."
In summary, the research trends in ion semi-permeable membranes powered by advancements in nanotechnology promise an exciting future not only in industrial applications but also in vital areas like healthcare. By continuing to explore these intersections, we stand at the brink of truly transformative developments that can address some of the most pressing challenges in both science and society.
Ending
In the vast realm of membrane science, the understanding of ion semi-permeable membranes stands out as both complex and vital. These membranes facilitate selective ion transport, which is crucial in numerous applications ranging from biological cellular functions to cutting-edge industrial processes. The significance of delving into this topic lies not only in grasping the fundamental mechanisms of ion permeation but also in recognizing the potential benefits these membranes provide in various fields such as environmental science, medicine, and technology.
Summary of Key Points
- Ion semi-permeable membranes play a foundational role in both biological systems and technology, affecting processes that range from nutrient absorption in cells to energy generation in batteries.
- These membranes are classified into natural, synthetic, and composite types, each serving distinct functions and having unique advantages.
- Key mechanisms governing ion transport include diffusion, facilitated transport, and active transport, which all depend on the specific properties of the membrane, such as pore size and charge.
- Applications in environmental science, particularly in water purification and wastewater treatment, underscore the membranes’ importance in addressing global challenges like clean drinking water access and pollution control.
- Current research trends focus on integrating nanotechnology to enhance membrane performance, signaling significant advancements in membrane design and functionality.
Final Thoughts on Ion Semi-Permeability
As we cast a gaze toward the future, the study of ion semi-permeable membranes bursts with promise and innovation. The integration of nanotechnology and advancements in synthetic membrane design could portend a new era in biomedical applications, particularly in drug delivery systems and implantable devices.
Ultimately, understanding these membranes profoundly impacts our approach to tackling pressing environmental issues and improving human health. Each layer of this topic reveals not just the operational science behind membranes, but their wider implications for both our daily lives and the scientific community. The pursuit of knowledge in this field is not merely academic—it serves as a cornerstone for advances that will shape the world to come.
"In science, there are no shortcuts to truth. Each discovery builds on previous knowledge, leading us to new possibilities."