Exploring Graphene Quantum Dots: Properties and Applications
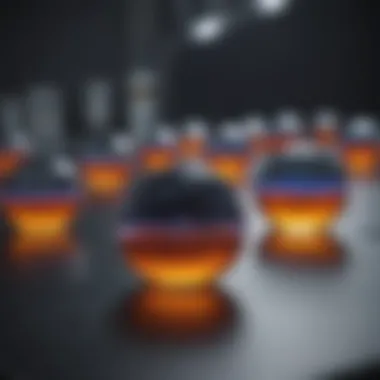
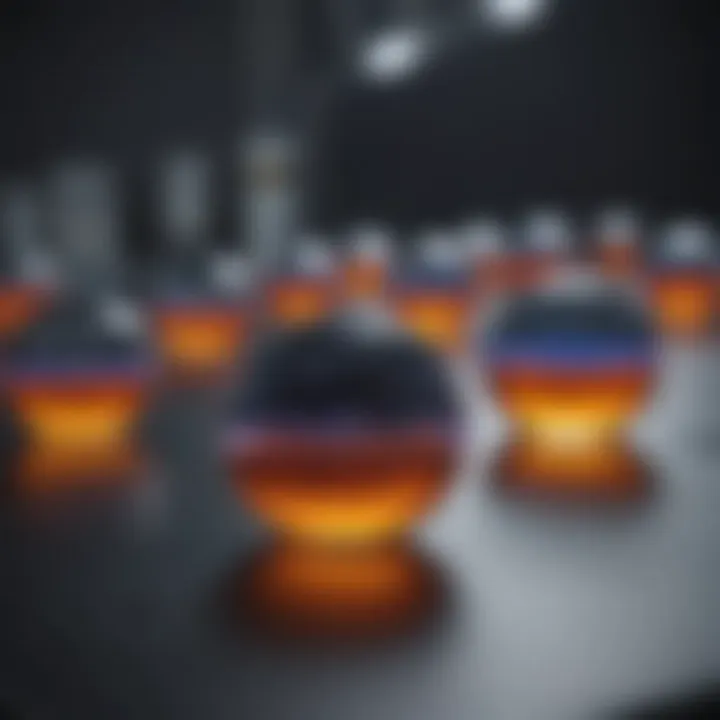
Intro
Graphene quantum dots (GQDs) are making waves across various scientific fields due to their unique size-dependent properties. These tiny particles, which are essentially small pieces of graphene, exhibit remarkable electrical, optical, and thermal characteristics. This has turned the spotlight on GQDs, not just for academic research, but also for their burgeoning applications in industries ranging from medicine to energy.
Researchers are keenly studying GQDs because their properties can be fine-tuned. When grappling with such materials, scientists must consider how size, shape, and surface chemistry impact their behavior. Understanding these elements is crucial for pushing the boundaries of their applications. The journey of GQDs invites an in-depth examination, providing insights into the methods used for synthesis, different avenues they're exploring for practical use, and the future paths that might open up.
As we delve deeper into the world of graphene quantum dots, the focus will extend to how these materials can transform existing technologies and spark innovations in yet-to-be-discovered fields. The following sections will cover the methodologies employed in researching GQDs, important discussions concerning their relevance, and the shiny future that awaits them.
Foreword to Graphene Quantum Dots
Graphene quantum dots have emerged as a cutting-edge topic within the fields of nanotechnology and materials science. Their remarkable properties and diverse applications make them an area of keen interest for researchers and engineers alike. As miniaturized versions of graphene, these dots boast unique electrical, optical, and mechanical features that cannot be overlooked. Understanding graphene quantum dots is essential, as they present a host of benefits in a variety of industries such as electronics, energy, and biomedicine.
The significance of exploring graphene quantum dots lies in their potential to enhance existing technologies and pave the way for new innovations. Given the growing need for efficient energy solutions, integrating graphene quantum dots into photovoltaic devices can lead to more effective solar cells. Likewise, their unique photoluminescent properties open avenues for advanced imaging techniques in biomedical applications.
Definition and Background
Graphene quantum dots (GQDs) are nanoscale fragments of graphene, typically measuring under ten nanometers. These quantum dots maintain certain properties of graphene while also exhibiting quantum confinement effects, which alter their electronic and optical characteristics. As they become increasingly smaller, the behavior of molecules shifts from bulk to quantum mechanical, giving rise to astonishing photon and electron interactions.
The sheer versatility of GQDs stems from their ability to exhibit various functional properties depending on their size and surface chemistry. In essence, GQDs combine the benefits of quantum dots with those of graphene, thus reinforcing their role as an exceptional material in current scientific research. With both hydrophilicity and hydrophobicity, they can also easily adapt to different environments, thereby enhancing their usability across multiple fields.
Historical Context of Graphene Research
The road to the discovery of graphene quantum dots is paved with extensive research on graphene itself, which began gaining traction in the early 2000s. Graphene, a single layer of carbon atoms arranged in a hexagonal lattice, caught the scientific community's attention due to its extraordinary properties such as high electrical conductivity and exceptional mechanical strength.
In 2004, Andre Geim and Konstantin Novoselov successfully isolated graphene, which later skyrocketed research efforts worldwide. It wasn't until a few years later that GQDs were identified and characterized. The re-emergence of interest in quantum dot technologies in the 2010s, along with the quest for smaller, more efficient materials, propelled GQDs into the spotlight.
As studies on GQDs gained momentum, examples of innovative uses began to flourish. From their role in enhancing the efficiency of LEDs to acting as fluorescent markers in bioimaging, graphene quantum dots quickly established a reputation as a game-changer in various applications.
"Graphene quantum dots not only reflect the advancements in nanotechnology but also signify a broader shift toward innovative materials in science and engineering."
Today, research continues to delve deeper into the functionalization and practical applications of GQDs, hinting at a promising future for this remarkable class of materials.
Structural Properties
Understanding the structural properties of graphene quantum dots (GQDs) is crucial in many aspects, spanning from their physical characteristics to their prospective applications. The intricacies tied to the atomic makeup and arrangements within these nanostructures lay the groundwork for their unique behavior and versatility in various fields. With different applications relying heavily on these properties, a detailed exploration of GQDs can unravel fundamental insights that push the boundaries of current technologies.
Atomic Structure of Graphene Quantum Dots
Graphene quantum dots possess a distinct atomic structure that primarily features a few layers of graphene, generally measuring fewer than 100 nanometers in diameter. Unlike bulk graphite or larger graphene forms, GQDs are characterized by their reduced dimensions, which significantly influence their electronic properties. The binding of carbon atoms in these dots occurs in a honeycomb lattice formation, imparting exceptional strength and conductivity.
Additionally, defects within the atomic structure also play a role in determining the properties of GQDs. Such imperfections can enhance photoluminescence, making these tiny dots valuable in applications like imaging. As researchers delve deeper, they uncover how manipulating the atomic arrangement can lead to remarkable outcomes in sensing and electronic devices.
Quantum Confinement Effects
The phenomenon of quantum confinement is pivotal in understanding GQDs' performance in diverse applications. When the size of a material approaches nanometer dimensions, classical physics begins to falter, giving way to quantum mechanics.
This effect can alter the electronic and optical characteristics of GQDs, triggering notable changes in their energy levels. For instance, as the size of graphene quantum dots decreases, the energy gap widens, leading to increased electron energy. This implies that smaller GQDs might exhibit different absorption and emission properties compared to larger counterparts.
Such alterations present significant opportunities; for example, tailored emission wavelengths can be engineered for applications in LEDs or display technologies. Thus, the quantum confinement effects stand as a cornerstone for innovating materials that push the envelope in optoelectronic devices.
Size-Dependent Properties
A key aspect of graphene quantum dots lies in how their physical and chemical properties evolve with their size. The smaller the quantum dot, the more pronounced these effects become. This size-dependent variability often governs optical features such as photoluminescence and absorption spectra.
For instance, larger GQDs might display broader absorption peaks, while smaller ones can result in sharp transitions. This behavior can be likened to how tuning an instrument alters its sound; slight changes in size can manifest substantial differences in emitted light color or absorption capabilities. By understanding these principles, researchers and engineers can design and fabricate GQDs precisely for specific applications like solar cells, pharmaceuticals, or sensor technologies.
"The future of materials science heavily relies on our mastery of nanostructures like GQDs, a journey paved by understanding their fundamental properties."
Keywords
- Graphene quantum dots
- Quantum confinement
- Atomic structure
- Size-dependent properties
- Photoluminescence
Synthesis Techniques
Synthesis techniques are a cornerstone of graphene quantum dots (GQDs) development. These methods not only influence the quality and properties of the dots but also shape their potential applications across various fields. Understanding these techniques is essential for researchers and developers aiming to leverage the unique characteristics of GQDs for technological innovations.
Top-Down Approaches
In the world of material synthesis, top-down approaches refer to breaking down bulk materials into nanoscale components. This method is vital in creating GQDs while ensuring specific structural integrity and functional properties.
Mechanical Exfoliation
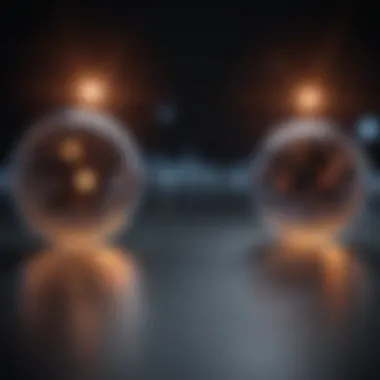
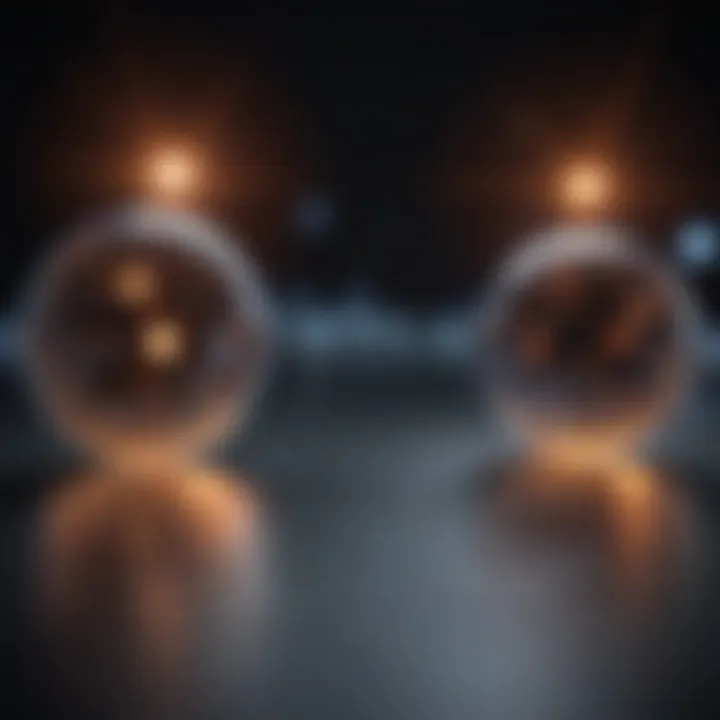
Mechanical exfoliation is akin to peeling an onion; it involves removing layers from a bulk material to achieve the desired nanostructure. This technique is celebrated for its simplicity and effectiveness. With minimal extra steps, researchers can produce high-quality graphene sheets, which can subsequently be processed into quantum dots. One key characteristic of mechanical exfoliation is that it retains the electronic properties of graphene, making it a favored choice in the initial stages of GQD research.
The unique feature of this method lies in its ability to produce GQDs with minimal defects. However, while mechanical exfoliation offers high-quality products, its scalability is often a sticking point since it may not produce large quantities of dots, which can limit its industrial application.
Etching Methods
Etching methods carve out nanostructures from larger sheets of graphene, utilizing a variety of chemicals or physical processes. This technique underscores precision in producing GQDs with a specific shape and size. The striking feature of etching methods is the ability to control the surface characteristics and functionality of the resulting quantum dots effectively.
Despite its advantages, etching can lead to unwanted defects, impacting the electronic properties of the GQDs. It's also worth noting that these techniques can be resource-intensive, adding to the costs involved in mass production.
Bottom-Up Approaches
Bottom-up approaches synthesize GQDs from molecular precursors, allowing for better control over the size, surface properties, and overall quality of the dots. This technique has gained traction for its ability to produce uniform and defect-free graphene quantum dots.
Chemical Vapor Deposition
Chemical vapor deposition (CVD) represents a sophisticated way to grow graphene layers—think of it as planting a garden where conditions are meticulously controlled for optimal growth. CVD stands out for its ability to produce high-purity GQDs with large surface areas. The process allows for precise tuning of the dimensions and properties of the resulting quantum dots.
The unique feature of CVD is its scalability; this technique is amenable for industrial applications, making it a popular choice among researchers. However, it often requires costly equipment and a controlled environment, which might deter some newcomers to the field.
Solvothermal Synthesis
Solvothermal synthesis involves a chemical reaction in a solvent under high temperature and pressure. This process enables the formation of GQDs with high purity while allowing for the introduction of various functional groups. The beauty of solvothermal synthesis lies in its potential to produce dots with desirable surface properties, which is crucial for many applications.
However, the required conditions for this synthesis can present challenges. It may lead to variable outcomes depending on the reaction parameters, and careful tuning is essential to achieve reproducible results consistently.
Novel Synthesis Routes
The emergence of new synthesis techniques highlights the dynamic nature of GQD research. These innovative methods focus on sustainability and efficiency, presenting alternatives to traditional techniques.
Biological Synthesis
Biological synthesis taps into nature’s complexity—using organic materials or biological processes to create GQDs. This method is intriguing because it minimizes environmental impact while producing functional materials. The key characteristic of biological synthesis is its ability to utilize waste materials, contributing to a more circular economy.
Despite its benefits, biological synthesis is still evolving, and reproducibility can be a concern. There's a degree of unpredictability when relying on biological systems, which may vary from batch to batch.
Green Chemistry Approaches
Green chemistry approaches prioritize sustainability in the synthesis of GQDs. By employing non-toxic solvents and using eco-friendly procedures, these methods not only reduce harm to the environment but also lessen health risks associated with toxic materials. The unique feature of green chemistry is its focus on reducing waste and energy consumption.
Though green techniques offer considerable promise, they can sometimes lead to lower quality products, necessitating further refinement. Researchers are actively exploring ways to enhance these methods to ensure they can compete with more conventional techniques on quality and scale.
The ongoing development of novel synthesis routes reflects a growing commitment to environmental sustainability within materials science.
Through a careful selection of synthesis technique—be it top-down or bottom-up—researchers aim to produce graphene quantum dots that can meet the rigorous demands of modern technology.
Optoelectronic Properties
Understanding the optoelectronic properties of graphene quantum dots (GQDs) is pivotal, as these characteristics play a vital role in various technological applications. These properties involve the interaction of GQDs with light and electricity, making them ideal candidates for innovations in electronic devices, light-harvesting applications, and even in medical imaging. The unique structure of graphene contributes to these properties, transforming potential technological capabilities.
Photoluminescence Characteristics
The photoluminescence (PL) of graphene quantum dots reveals much about their electronic and optical behavior. When light is absorbed by GQDs, they re-emit that light at different wavelengths, creating the phenomenon known as photoluminescence. This property can vary vastly depending on the size of the quantum dots and their surface functionalization.
- Size Matters: The size of GQDs significantly influences their emission spectrum. Smaller dots tend to emit light at shorter wavelengths, while larger GQDs emit at longer wavelengths. This size-dependent emission is essential for tailoring GQDs for specific applications, especially in optoelectronic devices.
- Surface Modifications: Functionalizing the surface of GQDs with different chemical groups alters their photoluminescence. This could lead to enhanced emission intensities, facilitating their application in bio-imaging and sensing technologies.
One significant aspect to note is that the photoluminescence efficiency of GQDs can also be tuned through temperature and concentration, providing versatility in practical applications. Research continues to explore ways to maximize the emissions and refine control over these parameters.
Electrical Conductivity
Another fundamental optoelectronic property of GQDs is their electrical conductivity, which plays a crucial role in electronic applications. While graphene exhibits exceptional conductivity overall, GQDs exhibit varying degrees of conductivity depending on their size and surface modification.
- Intrinsic Conductivity: GQDs generally showcase intrinsic conductivity facilitated by their unique electronic structure. The delocalized π-electrons in GQDs enable effective charge transport, essential for applications in transistors and sensors.
- Modulations: Changing environmental factors, such as temperature and electric field, can also affect conductivity. For instance, by doping GQDs with metallic nanoparticles, researchers can significantly enhance their electrical properties. This modulation process is vital for designing more effective electronic components.
The balance between photoluminescence and electrical conductivity makes GQDs remarkably interesting for developing optoelectronic devices that unite both functions, such as light-emitting diodes and solar cells.
Photonic Applications
Graphene quantum dots hold immense promise for a variety of photonic applications due to their unique optoelectronic properties.
- Light-Emitting Devices: GQDs can be utilized in organic light-emitting diodes (OLEDs), providing brighter and more efficient display technologies. Their tunable emission allows for potential advancements in full-colour displays.
- Solar Cells: Researchers are keen on leveraging GQDs to enhance the efficiency of solar cells. The combination of GQDs with traditional silicon cells has paved the way for photovoltaic systems that harness a broader spectrum of light.
- Sensing: The sensitivity of GQDs in emitting light makes them remarkable for sensing applications. For example, by integrating GQDs into biosensors, they can detect low concentrations of substances by measuring changes in PL intensity or wavelength.
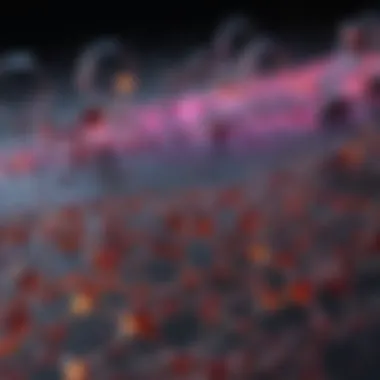
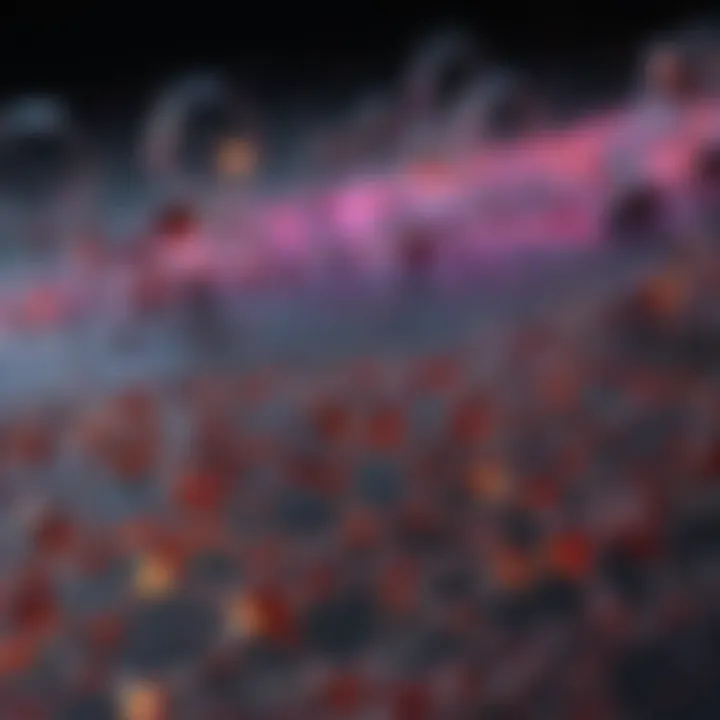
"The multifaceted optoelectronic properties of graphene quantum dots position them at the forefront of next-generation photonic devices, reflecting their potential to redefine the landscape of numerous technological domains."
As GQDs continue to be researched and developed, the horizon for their practical application becomes broader, indicating a transformative shift in how we utilize materials in electronics and beyond.
Applications in Technology
The applications of graphene quantum dots are multifaceted, having garnered attention from various fields including electronics, medicine, and renewable energy. Their unique properties, such as tunable photoluminescence and high electrical conductivity, make them significant assets across different technological platforms. As researchers dig deeper into the capabilities of these nano-sized structures, it's clear that understanding their role in technology will be paramount to future innovations.
Role in Photovoltaic Devices
Graphene quantum dots are shaking up the solar cell market. Traditional photovoltaic devices often struggle with efficiency, but integrating graphene quantum dots can enhance their light-absorbing capabilities. By tuning the size of these dots, researchers can optimize the light absorption spectrum. This means they can potentially capture more sunlight and convert it into energy more efficiently.
For instance, a recent study demonstrated that solar cells incorporating graphene quantum dots showed a 20% increase in efficiency compared to their silicon counterparts. This improvement comes partly from the dots' strong photoluminescence, which can funnel light more effectively into the active layers of the solar cell.
Moreover, the flexibility and lightweight nature of graphene quantum dots could pave the way for the development of a new class of solar applications, such as wearable solar technology. The potential is vast, not just for enhancing existing solar technologies but also for making solar cells more accessible and adaptable.
Utilization in Sensing Applications
When it comes to sensing technologies, graphene quantum dots offer remarkable possibilities. Their unique optical and electronic properties allow for high sensitivity in detecting various biological and chemical substances. This has particular implications in medical diagnostics, environmental monitoring, and food safety.
For instance, researchers have developed biosensors using graphene quantum dots that can detect glucose levels in blood drops with extreme accuracy. Such sensors are invaluable in diabetes management, providing real-time health monitoring without the need for complex machinery.
In another scenario, the environmental sector is utilizing these dots for detecting heavy metals in water sources. The surface modifications of graphene quantum dots can be tailored to bind specifically to contaminants, making them a powerful tool for quick and accurate assessments of water quality.
Integration with Semiconductor Technology
Graphene quantum dots are increasingly seen as vital to the semiconductor industry due to their unique electronic characteristics. They can be easily integrated into existing semiconductor processes, offering an avenue for the development of next-generation electronic components.
The miniaturization of electronic devices requires materials that can sustain performance at nano scales. Graphene quantum dots fit the bill perfectly due to their enhanced conductivity and tunable bandgap properties, which can lead to transistors with lower power consumption.
As the semiconductor industry grapples with the limits of silicon technology, the introduction of graphene quantum dots could potentially break down these barriers, ushering in a new era of faster and more efficient electronic devices.
"Graphene quantum dots hold the key to overcoming the limitations of current semiconductor technologies, enabling innovations that could redefine electronics as we know them."
In summary, the integration of applications in technology provided by graphene quantum dots is promising. Its role in photovoltaic devices enhances energy efficiency, while application in sensing devices offers critical advancements in health monitoring and safety. Furthermore, their compatibility with semiconductor technology indicates a leap forward in electronic development, ultimately pushing the boundaries of what's technologically possible.
Biomedical Applications
Biomedical applications of graphene quantum dots (GQDs) sit at the zenith of contemporary scientific exploration. These tiny yet powerful materials are not merely a passing fad; they hold transformative potential for several critical domains in medicine. Their unique properties, such as high surface area, tunable fluorescence, and biocompatibility, make them suitable candidates for a vast array of bio-related purposes. In this discussion, we focus on three main applications: drug delivery systems, imaging techniques, and therapeutic applications.
Drug Delivery Systems
One of the most exciting aspects of GQDs is their role in drug delivery systems. The ability to encapsulate drugs within these nano-scale structures offers significant advantages in targeting specific cells or tissues while minimizing side effects. GQDs can be functionalized to enhance their interactions with particular biological markers on target cells, allowing for more efficient drug delivery.
- Key Benefit: Improved targeting reduces the collateral damage to healthy cells, making it a safer treatment option.
- Consideration: However, one must remain cautious about the potential toxicity of the quantum dots themselves, necessitating thorough safety evaluations before clinical applications.
Imaging Techniques
In the realm of biomedical imaging, GQDs serve as brilliant probes. Their photoluminescence properties provide an excellent contrast that can enhance imaging techniques like fluorescence microscopy. This quality makes them invaluable in tracking cellular processes in real-time and diagnosing diseases.
- Unique Feature: GQDs can be engineered to emit different colors of light based on their size, leading to multi-color imaging.
- Advantage: This multi-color capability can enable deeper insights into complex biological systems by providing simultaneous information from multiple sources.
Therapeutic Applications
Photothermal Therapy
Photothermal therapy, an emerging treatment modality, uses GQDs for cancer treatment. The fundamental aspect of this technique is that GQDs can absorb light energy and convert it into heat, selectively destroying cancer cells while sparing surrounding healthy tissues. This synergistic effect can lead to significant reductions in tumor size.
- Key Characteristic: The capability of GQDs to generate localized heat is a major reason they are considered a frontrunner in non-invasive treatment options.
- Disadvantage: Nonetheless, careful consideration is needed regarding heat distribution and potential damage to adjacent healthy cells.
Targeted Therapy
Targeted therapy is another vital area benefiting from the application of GQDs. Through functionalization, these quantum dots can carry therapeutic agents directly to tumor sites, significantly improving treatment efficacy. Targeted therapy minimizes side effects that usually come with traditional therapies, offering a more personalized approach to cancer treatment.
- Unique Feature: The ability to couple GQDs with antibodies or ligands enables them to hone in on specific cancer types, facilitating tailored treatment plans.
- Advantage: This precision enhances the potential effectiveness of drugs by ensuring they reach their intended targets.
"Graphene quantum dots represent a marriage between nanotechnology and medicine, with the promise of enhancing treatment efficacy while reducing side effects."
In summation, the biomedical applications of graphene quantum dots are extensive and varied. Even as researchers confront the challenges associated with their use, the potential benefits—ranging from safer drug delivery systems to advanced imaging techniques and cutting-edge therapies—herald a new dawn in medical science. As knowledge and technology evolve, so too will the possibilities for harnessing GQDs in improving human health.
Challenges and Limitations
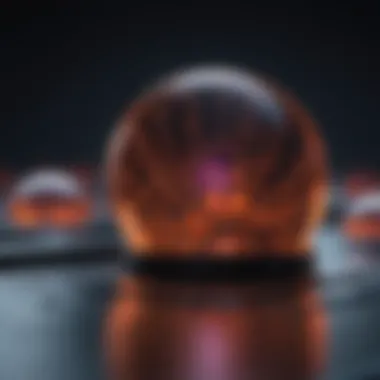
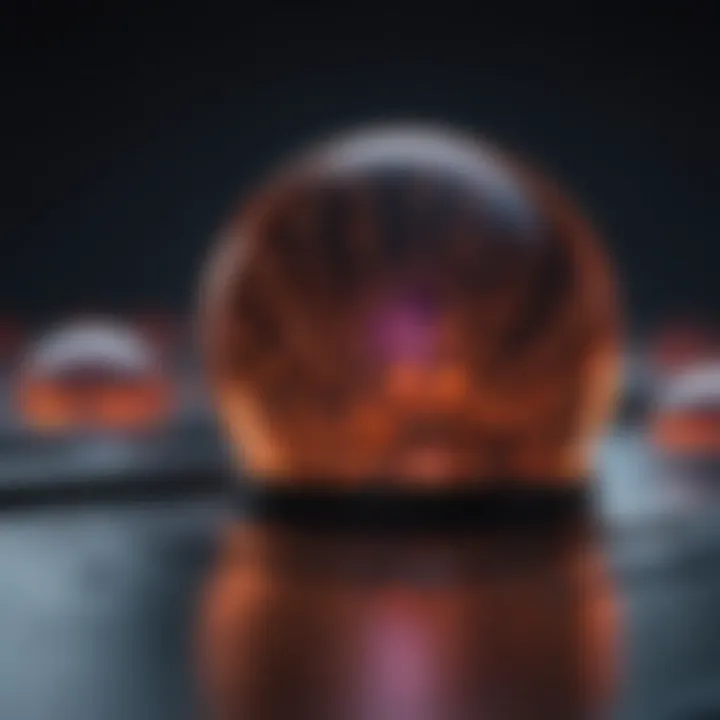
Understanding the challenges and limitations associated with graphene quantum dots is vital for comprehending their potential impact on various fields. While these materials hold great promise, addressing inherent issues is key to advancing their practical applications. Researchers and developers are constantly striving to maximize the benefits of graphene quantum dots while navigating obstacles that could hinder progress. This section delves into specific considerations regarding their stability and toxicity, the scalability of production methods, and the regulatory hurdles faced in biomedical applications.
Stability and Toxicity Concerns
One significant concern regarding graphene quantum dots is their stability under varying environmental conditions. Factors such as humidity, temperature, and exposure to light can affect their properties, potentially resulting in degradation or altered behavior. Moreover, their toxicity is under scrutiny, especially for applications in healthcare. Studies indicate that certain graphene quantum dots can elicit adverse biological responses, raising questions about their safety for drug delivery and imaging techniques.
To navigate these issues, researchers are investigating strategies to enhance the stability of these materials. For example, functionalization methods can be applied to improve biocompatibility and reduce toxicity. By modifying the surface characteristics of graphene quantum dots, it may be possible to better control their interactions within biological systems. However, this quest for safety does not come without complexities, as each modification may alter their effectiveness in applications.
Scalability of Synthesis Methods
Despite the advancements in synthesis techniques, scalability remains a pressing challenge. Current methods, especially top-down approaches like chemical etching, often yield limited quantities of graphene quantum dots. This limitation poses a barrier for industrial applications where large-scale production is essential. Notably, bottom-up syntheis approaches, such as chemical vapor deposition, show promise but can be cost-prohibitive and complex.
Innovations in synthesis techniques are critical to overcoming these scalability issues. Researchers are exploring alternative pathways, including green chemistry methods, which not only aim to optimize yields but also focus on environmental sustainability. Nonetheless, crafting scalable solutions that do not compromise the quality or properties of graphene quantum dots remains a complex undertaking, demanding continued research and collaboration across multiple scientific disciplines.
Regulatory Hurdles in Biomedical Applications
When it comes to applying graphene quantum dots in the biomedical field, navigating the regulatory landscape is a daunting challenge. These materials must undergo rigorous testing to ensure their safety and efficacy before being approved for clinical use. Different countries have distinct regulatory frameworks, which can complicate international research and collaboration.
A key challenge is the lack of standardized protocols for assessing the toxicity and biocompatibility of graphene quantum dots. As these materials are relatively novel, existing regulations may fall short in addressing their unique characteristics. This gap necessitates the development of new regulatory guidelines that encompass the peculiarities of nanomaterials.
"The road to effectively integrating graphene quantum dots into medical applications will be paved by overcoming regulatory challenges, ensuring that innovation does not outpace safety considerations."
In summary, the challenges associated with graphene quantum dots—be it their stability, production scalability, or navigating regulatory landscapes—are significant but not insurmountable. As the field progresses, continued dialogue and research will be essential to unlock the full potential of these fascinating materials.
Future Directions in Research
The exploration of graphene quantum dots (GQDs) is still in the nascent stage, but the implications for future research are profound. These structures promise to reshape various scientific fields by leveraging their distinct properties and functionalities. Understanding these future directions is not merely an academic exercise; it’s a gateway to innovation that can impact technology, medicine, and environmental solutions. As we venture into this realm, it's crucial to look into specific avenues like synthesis advancements, new applications, and functionalization strategies.
Innovations in Synthesis Techniques
One significant area to focus on is the innovation in synthesis methods. As GQDs gain traction, the need for efficient, cost-effective, and environmentally friendly synthesis routes becomes essential. Several promising techniques are emerging:
- Hydrothermal methods: Utilizing high-pressure steam to facilitate the formation of GQDs shows potential for scalability and uniformity in production.
- Laser ablation: This technique offers high precision and allows for the production of GQDs with tailored sizes and functionalities.
- Electrochemical exfoliation: By applying voltage, it can transform bulk graphite into nano-scaled forms effectively.
Each of these methods has its own pros and cons, but the ongoing research aims to refine these processes to make them more viable in real-world applications. The interplay between accessibility and efficacy in synthesis will largely determine how widely adopted GQDs will become.
Emerging Applications in Energy Storage
Energy storage is another promising frontier for GQDs. With the ongoing struggle to improve battery technologies, GQDs display remarkable potential as components in supercapacitors and lithium-ion batteries due to their high surface area and conductivity. Some key points include:
- Enhancing charge storage capacity: GQDs can significantly increase the energy density of traditional batteries, making them more efficient.
- Facilitating quicker charge times: Due to their excellent conductivity, GQDs enable fast electron transport, reducing the time required for charging.
- Environmental impact: By integrating GQDs, energy storage systems could lead to greener technologies, lessening reliance on toxic materials in current battery designs.
Companies and research institutions are exploring these dynamics rigorously, which might usher in a new era of sustainable energy storage solutions.
Advancements in Functionalization
Functionalization of GQDs represents yet another critical area for future research. By tweaking their surface chemistry, the properties of GQDs can be tailored to meet specific needs, enabling a plethora of applications. Consider the following advancements:
- Targeted drug delivery: Functionalized GQDs can help deliver medications directly to diseased cells, improving the efficiency of treatments while minimizing side effects.
- Sensor development: By attaching functional groups, GQDs can be engineered to selectively interact with various biomolecules, resulting in sensitive biosensors for medical diagnostics.
- Photonic devices: Their optical properties can be fine-tuned, allowing for innovative applications in telecommunications and display technologies.
"The journey of graphene quantum dots is just beginning, yet the possibilities are endless; as researchers push the boundaries of their capabilities, the transformative power of these materials could lead to groundbreaking advancements across multiple domains."
As the landscape of GQD research continues to evolve, the commitment to innovation in synthesis, application, and functionalization stands as a beacon for future exploration. Each stride taken opens up new avenues, inviting researchers and professionals to partake in this exciting journey.
Culmination
The exploration of graphene quantum dots stands as a landmark entry in the narrative of modern material science. This article encapsulates the intricate properties, diverse applications, and compelling future directions associated with this remarkable material. The significance of graphene quantum dots extends far beyond their fundamental characteristics; they embody a bridge between theoretical nanoscience and practical applications that promise to revolutionize several industries.
Summary of Key Insights
Graphene quantum dots exhibit several unique attributes due to their atomic structure and size. The confinement of electrons gives rise to size-tunable optical properties, useful in various technological sectors such as electronics and optoelectronics. Additionally, the synthesis methods discussed highlight the growing capability to produce high-quality dots at scale, augmenting their practicality for real-world applications. One can't overlook their role in biomedical fields—ranging from drug delivery systems to imaging techniques—which underscores their versatility and effectiveness.
The benefits derived from these insights include:
- A nuanced understanding of how structural properties impact performance in applications.
- Recognition of the synthesis advancements paving the way for large-scale implementations.
- Insight into the multifaceted applications, especially in biomedicine, illustrating their potential to enhance existing technologies.
Implications for Future Research
The future of graphene quantum dots is not merely promising; it is ripe with potential for groundbreaking developments. Continued research focused on innovative synthesis techniques can lead to more efficient production methods, ultimately breaking down cost barriers. Moreover, exploration in emerging fields such as energy storage, particularly in battery technology and supercapacitors, may yield profound effects on sustainability efforts.
Furthermore, as environmental concerns loom larger, advancements in functionalization methods could catalyze the creation of truly sustainable materials. To foster a holistic approach, interdisciplinary collaboration is essential, encouraging researchers from various domains to share insights and strategies.
In summary, further exploration of graphene quantum dots is bound to unveil new applications and functionalities, shaping not only technology but also addressing significant global challenges. As researchers and professionals dive deeper, the synergy between scientific inquiry and practical application will hopefully yield innovations that impact society at large.
"In the world of nanotechnology, the possibilities are as vast as the universe itself."
Understanding these dimensions underlines the critical role of graphene quantum dots and lays the groundwork for future advancements in science and technology.