Chiral Balance: Understanding Molecular Symmetry
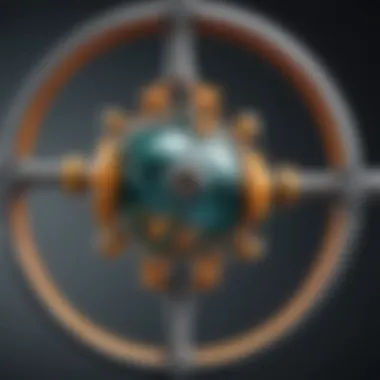
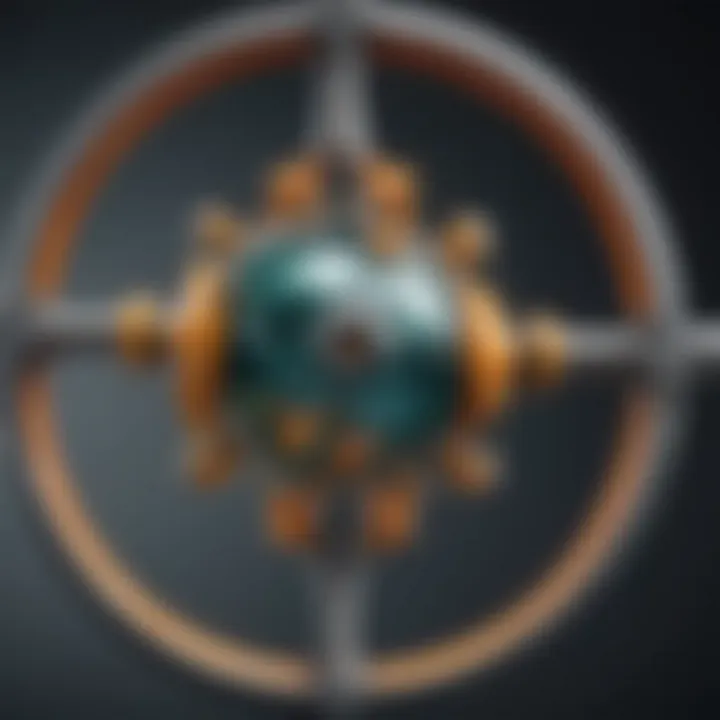
Intro
Chirality isn't just a fancy term tossed around in chemistry; it's a foundational concept that influences countless aspects of our lives, from the scents we embrace to the medications we take. This duality, where molecules can exist in mirror-image forms, leads to significant implications, especially when we consider the balance—or lack thereof—these forms relative to each other in the realm of molecular interactions. An imbalance in chirality can have pronounced effects, particularly in pharmaceuticals where one form can offer therapeutic benefits while its mirror image might cause adverse effects. As science marches forward, probing deeper into the nuances of chiral balance, we uncover not just the fundamental principles of chirality, but also how this intricate symmetry governs interactions on a molecular level.
Understanding chiral balance requires delving into its impact on life’s processes, including how organisms utilize chiral molecules for energy and survival. It also opens the door to discussing advancements in the synthesis and characterization of chiral compounds, which can lead to breakthroughs in drug design, environmental chemistry, and beyond. This piece will explore the fundamental principles underlying chirality, spotlighting its vital role across various scientific disciplines.
As we peel back the layers of chiral interactions, we aim to reach not just those engrossed in academia, but all audiences intrigued by the profound implications of this molecular phenomenon.
Understanding Chirality
Chirality is more than just a term tossed around in chemistry textbooks; it's a fundamental concept that shapes a myriad of disciplines, from biochemistry to pharmaceutical design. If you think of molecules as little sculptures, chirality explains why some sculptures can’t be superimposed on their mirror image. Picture a pair of gloves: the left and right ones are mirror images, yet they fit only their respective hands. This idea may seem quaint, but it’s a cornerstone in how we understand molecular interactions.
The importance of compehrending chirality lies in its impact on biological systems and drug development. Many biological molecules, such as amino acids and sugars, exhibit chirality, which means they interact with one another in very precise and unique ways. If we mess with that balance, we’re not in a good place. For example, one chiral form of a drug may work wonders, while its counterpart could be ineffective or even toxic. This interplay ignites a powerful need to grasp the subtleties of chirality and its implications in designing treatments and understanding biology.
In this article, we will delve into key elements that showcase aspects of chirality, including how it manifests in molecular structures, its properties, and the phenomenon of optical activity. By illuminating this topic, we hope to empower students, researchers, and professionals to appreciate the intricate balance of chiral molecules and their relevance in the wider fabric of science and industry.
The Concept of Chirality in Chemistry
Chirality in chemistry refers to the geometrical property of some molecules and ions. Simply put, certain molecules exist in two forms, known as enantiomers, that are non-overlapping mirror images of each other. Each enantiomer can behave quite differently when engaging with other chiral environments, such as enzymes or receptors.
Consider your common table salt versus the amino acid alanine: the former is achiral because it doesn’t have a distinct left or right-hand counterpart, whereas alanine is chiral. The carbon atom in alanine is bonded to four different groups, branding it as a chiral center. Without recognizing these subtle differences, scientists could end up pursuing pathways that carry little to no result.
Chiral Molecules and Their Properties
Molecules that showcase chirality carry distinct properties influenced by their spatial arrangement. One of the intriguing aspects of chiral molecules is their potential for differing reactivities and interactions based on which enantiomer you have. Some might have a sweet taste while the other is tasteless. A classic example is limonene, a compound found in citrus fruits. Its one enantiomer produces a citrus scent, while the other exudes a pine-like aroma.
Additionally, chirality affects how these molecules interact with light, resulting in optical activity that can be utilized for characterizations.
Optical Activity: Chirality in Action
Optical activity is a particularly fascinating manifestation of chirality. When polarized light passes through a solution containing chiral molecules, its rotation can indicate the presence and concentration of those molecules. This phenomenon is utilized in laboratories as a primary method for identifying and quantifying chiral substances.
It’s essential in processes like determining the purity of pharmaceutical drugs, where specific enantiomer proportions can drastically affect drug efficacy. To illustrate this, imagine a solution confidently spinning clockwise; it suggests a particular enantiomer is predominant. In contrast, counter-clockwise rotation may indicate another form. This simple, elegant act of light interacting with chiral molecules encapsulates the beauty of this complex subject.
Chirality plays a pivotal role in chemistry and biology, influencing molecular interactions that can determine life or death in biological systems.
Through understanding these concepts, we begin to see a world shaped by balance and symmetry, enabling us to harness the power of chiral compounds to advance the realms of science, health, and beyond. The immediacy of this knowledge isn’t just for chemists and biologists; it extends to industries, medical professionals, and even consumers in understanding the products they encounter daily.
Chiral Balance in Biological Systems
Chirality, in the context of biological systems, plays a pivotal role in the complex dance of molecular interactions that sustain life. The balance of chiral molecules is not just a trivial aspect of biochemistry; it underpins almost all biochemical processes. Whether it’s the way enzymes catalyze reactions or how drugs interact with biological targets, chirality is central. A deep dive into this area reveals not only the significance of chirality but also the fascinating interplay between structure and function.
The Role of Chirality in Biochemistry
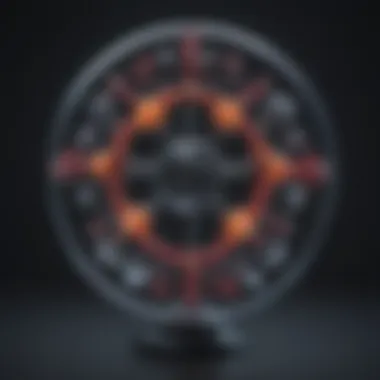
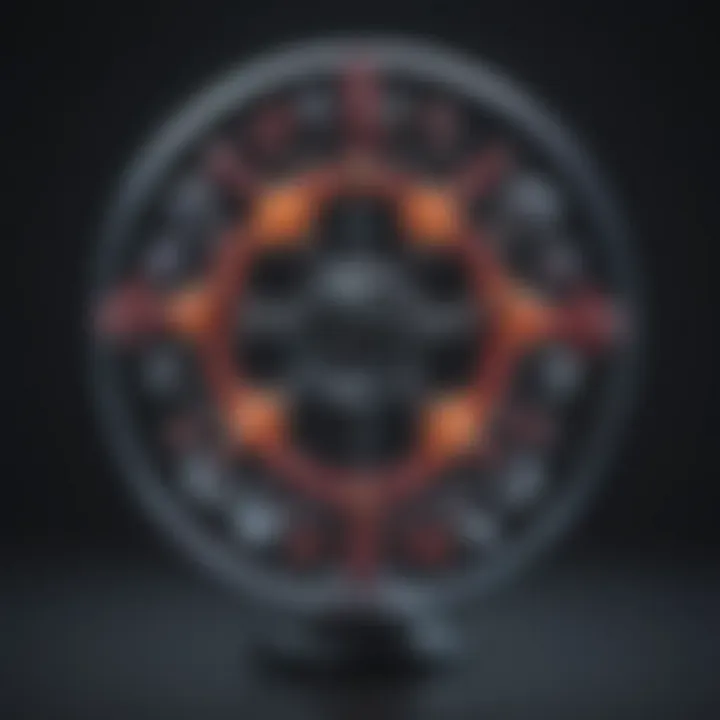
Chirality is a hallmark of many biological molecules, particularly amino acids and sugars. In nature, only certain enantiomers play active roles. For example, amino acids, the building blocks of proteins, are predominantly found in their L-forms, while D-forms are rarely utilized. This selectivity is crucial; proteins composed solely of one type are functionally distinct from those that mix forms.
Chirality's impact extends to metabolic pathways. Consider glucose, a simple sugar. Its D-form is what our bodies use to produce energy, while the L-form is virtually non-existent in biological systems. This specificity is not just an interesting fact; it has real-world implications. If the wrong enantiomer accumulates, it can disrupt normal cellular function, leading to metabolic issues. In essence, the molecular mirror image often determines survival.
Chiral Drugs and Their Efficacy
In the realm of pharmaceuticals, chirality takes center stage, and the implications are profound. Many drugs are chiral, and even a slight variation in molecular configuration can lead to vastly different effects in the body. One famous example is thalidomide. One enantiomer alleviated morning sickness, while its mirror image caused serious birth defects. The tragedy emphasized the need for rigorous chiral analysis in drug development.
Understanding this concept can significantly influence drug design and provide insights into personalized medicine. When drugs traditionally tailored to active enantiomers are administered, side effects often arise from the non-active counterpart. Therefore, developing single-enantiomer drugs could lead to better efficacy and safety profiles.
Chiral Imbalance and Biodiversity
The significance of chiral balance extends beyond human health and touches ecological dynamics. An imbalance in chiral substances can manifest in various ways, impacting biodiversity and ecosystem stability. For example, certain species may rely on specific chiral compounds for food or habitat. If those compounds are altered, the species that depend on them could face survival challenges.
Moreover, as the environment becomes increasingly polluted with chiral contaminants, the consequences skyrocket. Chiral pollutants can affect organisms differently, leading to various degrees of toxicity, which unravels the intricate tapestry of ecological relationships. Preserving chiral balance therefore becomes a matter of ecological importance.
"The existence of chirality in biological systems reveals a deep-seated preference not only within molecules but also across the spectrum of life itself."
In summary, the presence and proper balance of chirality in biological systems are not merely academic considerations. From fundamental biochemical processes to the practical implications in drug design and environmental health, understanding chirality can illuminate many facets of life. This intricate web of interactions underscores the necessity for a closer examination of chiral compounds as we venture toward a coherent understanding of nature's blueprint.
Synthesis and Characterization of Chiral Compounds
The world of chemistry often revolves around the seemingly simple yet profound concept of chirality. Synthesis and characterization of chiral compounds play a pivotal role in understanding this concept. These processes form the backbone of creating compounds that have the desired chirality, an essential aspect for their function in biological systems and pharmaceutical applications. With chirality influencing the activity of molecules, grasping the methods of synthesis and how to characterize these compounds is crucial.
A few key elements one might consider include cost-effectiveness, efficiency, and the intended application of the synthesized compounds. Some methods are bound to yield a particular enantiomer, while others might produce a mix that needs further separation. This takes us directly into the intricate tango of constructing perfect chiral compounds, wherein every leap in synthesis can either lead to breakthroughs or missteps.
Methods of Chiral Synthesis
Chiral synthesis is a somewhat refined art; it's not merely about creating molecules, but crafting them with precision. Several techniques stand out:
- Asymmetric Synthesis: This method utilizes catalysts that can preferentially promote the formation of one enantiomer over another. A classic example would be the use of chiral ligands in transition metal catalysis.
- Kinetic Resolution: It leverages the difference in reactivity between enantiomers, allowing one to be selectively reacted while the other remains untouched. While effective, this method often leads to incomplete conversion.
- Dynamic Kinetic Resolution: A more advanced form that combines racemization and asymmetric transformation, effectively allowing for maximum yield of a desired enantiomer.
Choosing the right method often depends on the nature of the substrate and the desired outcome. Each approach presents its own unique sets of challenges and opportunities, making it a field ripe for innovation.
Chiral Catalysis: An Overview
Chiral catalysis stands at the forefront, marrying the principles of catalysis with chirality to propel the synthesis of specific enantiomers. By employing chiral catalysts, chemists can influence the pathway of reactions, steering them toward creating one enantiomer over the other. This has substantial implications, especially in drug design, where the efficacy of a compound can hinge on its chirality.
Some remarkable advancements include:
- Organocatalysis: Utilizing small organic molecules as catalysts, this approach has transformed the way we think about synthetic routes. It’s greener and often simpler than metal-based catalysts.
- Enzyme Catalysis: Nature’s own catalysts, enzymes, provide a robust model for underpinning chiral synthesis and immensely aid in creating more complex molecules that would be difficult to assemble through traditional organic chemistry.
Tools for Characterizing Chirality
Once compounds are synthesized, understanding their chirality is next on the agenda. Several tools and methods exist to characterize these compounds:
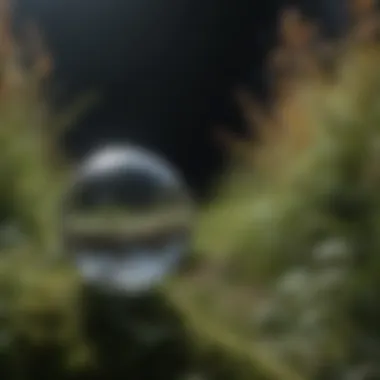
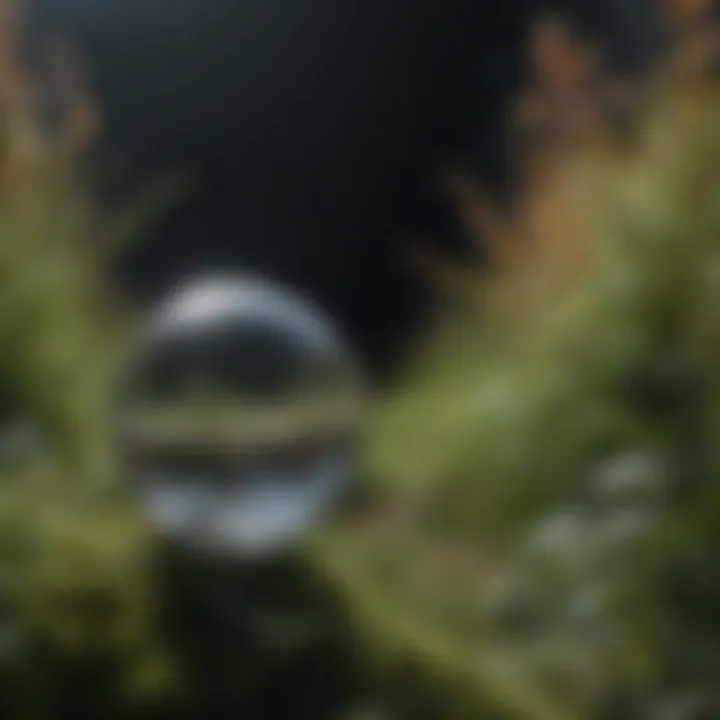
- Polarimetry: A direct method to measure optical activity, polarimetry assesses how chiral molecules rotate polarized light, thus indicating their enantiomeric composition.
- Nuclear Magnetic Resonance (NMR) Spectroscopy: This powerful technique can distinguish between enantiomers, especially when enhanced by chiral solvents or derivatization processes.
- Chiral Chromatography: Techniques such as high-performance liquid chromatography (HPLC) tailored for chiral separations allow chemists to efficiently separate enantiomers, yielding insights into their purity and composition.
In sum, the synthesis and characterization of chiral compounds are not just routine practices; they are crucial for unlocking the biological potential that chirality embodies. Future advances in these fields promise to keep pushing boundaries, leading to novel compounds, new applications, and a deeper understanding of chiral balance in both chemistry and biology.
Chiral Balance and Pharmaceutical Applications
Chirality plays a monumental role in the realm of pharmaceuticals, influencing not just how drugs interact with their biological targets, but also their efficacy and safety. In drug design, an understanding of chiral balance is paramount as even minor changes in molecular structure can yield vastly different biological outcomes. Given that the human body is a chiral environment, the configuration of drug molecules can lead to significant variations in therapeutic effects. Ignoring chirality can result in the development of ineffective drugs or, worse, harmful side effects.
Impact of Chirality on Drug Design
The impact of chirality on drug design cannot be overstated. Each enantiomer of a chiral molecule often exhibits distinct behavior in biological systems, making it essential to isolate and optimize one form over another. Consider the case of Thalidomide, a drug that was prescribed in the late 1950s and early 60s. One enantiomer was effective as a sedative, while its mirror image led to severe birth defects. This tragic example underscores the need for thoughtful consideration of chirality in drug development. Modern methodologies now emphasize selective synthesis of specific enantiomers, significantly reducing risks and enhancing therapeutic efficacy.
"The same molecular structure can lead to two entirely different therapeutic pathways; it’s a dance of molecules where one moves gracefully while the other stumbles."
The advancements include techniques like chiral chromatography and asymmetric synthesis, allowing chemists to refine drug formulations with a keen eye on chirality. Thus, successful drug design is not merely a matter of what works; it’s about what works best, precisely.
Case Studies: Success and Failure
Examining successful and failed pharmaceutical endeavors offers rich insights into the importance of chirality.
Successful Cases:
- Ibuprofen: A classic example where the S-enantiomer is the active form responsible for its anti-inflammatory effects. Studies show that isolating this form brings about potent relief from pain without the adverse effects often associated with non-steroidal anti-inflammatory drugs (NSAIDs).
- L-DOPA: Used to treat Parkinson's disease, this enantiomer demonstrates how selecting the right chiral form can significantly improve a patient's quality of life, aiding in the restoration of dopamine levels in the brain efficiently.
Failures:
- Thalidomide serves as the archetype of a pharmaceutical failure, where the oversight of chirality led to dire consequences.
- Cetirizine: While one enantiomer of this antihistamine is effective, the other isn’t. A blend may have led to unnecessary side effects, but separating the enantiomers has made treatments much safer.
These cases illustrate how the balance of chiral compounds can mark the difference between a groundbreaking success and a catastrophic failure.
Regulatory Perspectives on Chiral Drugs
Regulatory bodies now place an increased emphasis on chirality in drug approval processes. Organizations like the FDA and EMA require extensive documentation on the stereochemistry of drug candidates. This scrutiny helps ensure that only the most efficacious and safest enantiomer is brought to market.
- Guidelines include detailed requirements for stereochemical characterization and evaluation of biopharmaceutical behavior.
- Approval Processes advocate for the prioritization of chiral drugs that demonstrate optimized therapeutic profiles.
As research progresses, the regulatory landscape adapts, becoming more robust in addressing the nuances of chiral balance in pharmaceuticals. This evolution reflects a broader understanding of molecular interactions, emphasizing the need for precision in drug development that benefits both the industry and public health.
Environmental Implications of Chiral Chemistry
Chirality extends its reach beyond the confines of laboratories and pharmaceuticals, weaving into the fabric of our environment. The implications of chiral chemistry can be as significant as they are complex. As researchers and professionals, it's vital to understand this relationship, particularly in terms of environmental sustainability and biodiversity.
Chiral compounds, considered as a distinct class of molecules, exhibit activities that depend on their spatial arrangements. This uniqueness grants them differential effects on biological systems. As such, the presence of chiral pollutants can result in unexpected ecological outcomes. For instance, one enantiomer may be harmless to certain organisms, while its mirror image could be toxic. Thus, the balance of chirality is not just a matter of scientific curiosity; it's a fundamental element in maintaining ecological equilibrium.
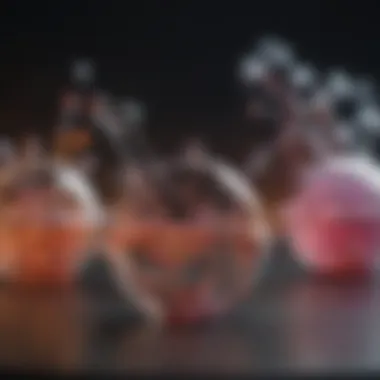
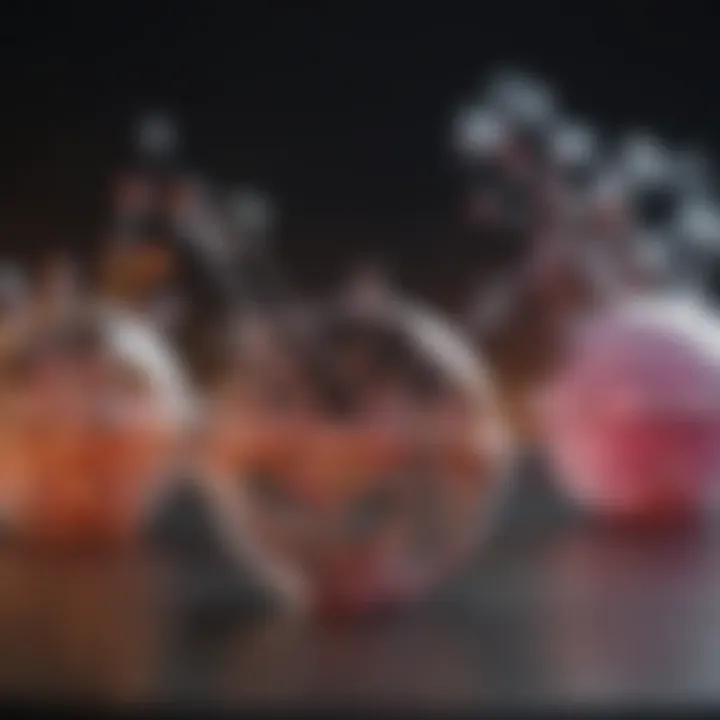
Chiral Pollutants and Their Effects
Pollutants in our environment often exist as chiral molecules. Their effects can vary drastically based on their chiral forms. To illustrate, consider the pesticide chiral cypermethrin. This widely used insecticide has two enantiomers, one of which is significantly more toxic to certain beneficial insects than the other. Such instances raise crucial questions about agricultural practices and the long-term health of ecosystems.
- Toxicity Variation: Different enantiomers can interact with biological systems in contrasting ways, influencing not only the targeted species but also non-target organisms, including beneficial species that are pivotal in maintaining ecological balance.
- Bioaccumulation: Over time, chiral pollutants can accumulate in the food chain, magnifying their effects in higher trophic levels. This process can lead to reduced populations of sensitive species and potentially disrupt entire ecosystems.
- Remediation Challenges: Traditional remediation approaches might not distinguish between enantiomers, complicating the cleanup efforts of contaminated sites. Techniques that specifically target harmful enantiomers need to be developed for more effective solutions.
Chirality in Ecotoxicology
Ecotoxicology, the study of toxic substances in the environment, often takes chirality into account. As we probe deeper into local ecosystems, the implications of chiral compounds become clearer. For instance, the assessment of chiral toxicity is becoming integral in understanding how different enantiomers affect aquatic organisms, flora, and fauna.
- Testing Methods: Researchers utilize various in vitro and in vivo methods to assess the relative toxicities of chiral molecules. This can help identify which enantiomer poses the greatest threat to aquatic environments. Moreover, biotechnological advancements have enabled the development of more precise assays to test for chiral toxicity.
- Regulatory Frameworks: Regulatory bodies are increasingly emphasizing the need to consider chirality in environmental studies. Guidance documents are being updated to reflect these insights, so that ecotoxicological assessments consider the full spectrum of biological impacts stemming from chiral pollutants.
Assessing Chiral Balance in Ecosystems
Understanding chiral balance is essential for sustaining healthy ecosystems. Imbalances can lead to detrimental effects that cascade through food webs, affecting species diversity and ecosystem services.
- Biodiversity Monitoring: Continuous monitoring is crucial. Utilizing tools that can analyze chiral compounds in various species helps scientists assess molecular diversity and its ecological implications.
- Ecosystem Resilience: Ecosystems exhibit varying levels of resilience depending on their component species. By observing how different species respond to chiral pollutants, researchers can evaluate the resilience of ecosystems to external stressors.
- Future Directions: Emerging studies are looking into the role of chirality in environmental resilience and recovery. Understanding the chiral nature of compounds can aid in forecasting how ecosystems adapt to changing conditions.
"The spatial arrangement of atoms is not merely a chemical curiosity; it is a potential determinant in the health of entire ecosystems."
Reflecting on these aspects, we see that the interplay of chirality and environmental science is a burgeoning field with substantial implications. Chiral chemistry is not just a niche interest; it is pertinent in addressing ecological challenges we face today.
Future Prospects of Chiral Research
The discussion surrounding the future of chiral research signals a critical juncture, underpinning both scientific inquiry and practical application. As we look ahead, the importance of this area comes into sharper focus, influenced by the increasing complexity of biomolecular interactions and the need for specificity in drug design. Realizing the full potential of chirality not only holds promise for enhancing pharmaceutical efficacy but also in addressing pressing environmental challenges.
Innovations in Chiral Synthesis
Chiral synthesis is at the forefront of future research, continuously adapting to the dynamically changing landscape of chemical production. Innovative methods are being developed that promise higher selectivity and lesser waste. An example to note is the use of biocatalysts for asymmetric synthesis. Unlike traditional methods, this approach utilizes enzymes which offer regioselective reactions under mild conditions.
- Enzyme engineering is evolving, leading to tailored enzymes capable of catalyzing specific reactions more efficiently.
- Flow chemistry brings another layer of efficiency, allowing for continuous reactions that reduce reaction times and improve yields.
- Solid-supported catalysts present an opportunity to streamline processes while increasing chiral purity.
These innovations are not merely academic; they can radically impact manufacturing processes in pharmaceuticals, making them greener and more affordable. Through concerted efforts in these technologies, researchers are narrowing the gap between theory and practical application.
Interdisciplinary Approaches to Chirality
The next phase of chiral research will likely demand a collaborative approach, bringing together diverse fields such as chemistry, biology, environmental science, and even artificial intelligence. Integrating these disciplines holds great promise for fostering breakthroughs in how we perceive and manipulate chirality. For example, leveraging machine learning algorithms to predict chiral center formation could revolutionize molecular design processes. Collaborative research frameworks can lead to:
- Better insights into molecular interactions at biological levels, aiding in the development of targeted drugs.
- Environmental assessments that consider chirality's role in ecosystem health.
- New pathways for materials science, pushing the envelope on creating chiral materials that can have practical applications in electronics or energy storage.
By bridging gaps between various spheres of study, there is enormous potential to cultivate innovative solutions tailored for today’s complex challenges.
The Path Ahead: Challenges and Opportunities
Despite the optimism that accompanies the future of chiral research, challenges remain that must be addressed to advance our understanding and application of chirality. One prominent hurdle lies in the standardization of chiral analysis techniques, which differ significantly across fields and applications. Developing universally accepted methods for chirality assessment will not only streamline research but also enhance regulatory compliance, which is paramount in pharmaceuticals.
Moreover, there are financial constraints tied to research funding, often causing projects to stall. Finding avenues for public-private partnerships could alleviate some of the financial burdens and foster innovation through resource sharing.
The synergy between scientists and industry can catalyze advancements that will prove beneficial for both parties.
The future of chiral research is laden with potential, shaped by both challenges and opportunities. As breakthroughs and innovations unfold, the research landscape is set to transform, significantly influencing various fields and paving the way for new applications. The call to action is clear: embracing interdisciplinary collaboration and addressing critical challenges will foster the growth of this ever-evolving area of science.