Exploring Flow Cytometry: Applications and Insights
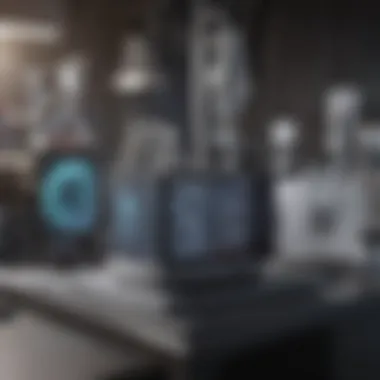
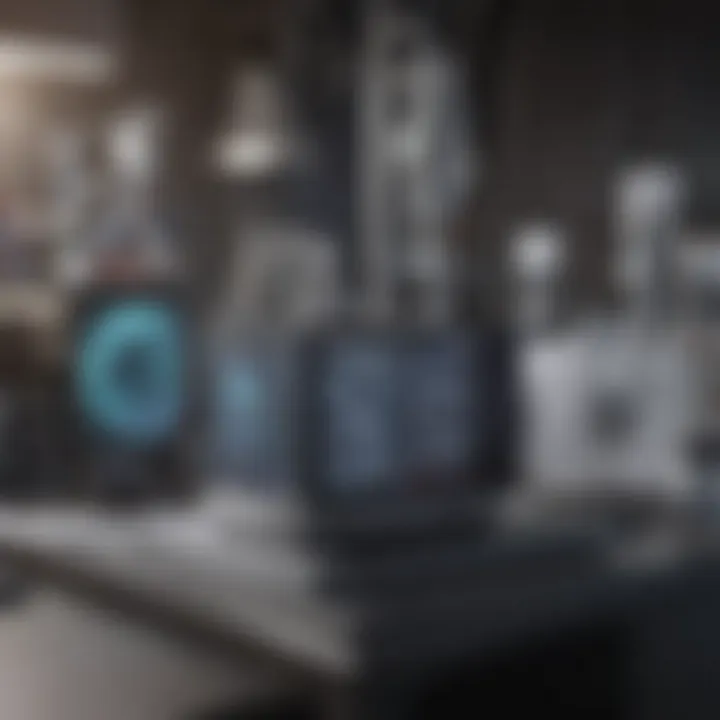
Intro
Flow cytometry has emerged as a pivotal technique within the realms of research and clinical diagnostics. Its capabilities allow for the precise analysis of cellular properties, making it invaluable in various scientific fields. By leveraging its advanced analytical features, researchers can gain insights into immune responses, tumor biology, and microbial dynamics. This understanding is crucial for advancing personalized medicine and improving patient care.
The significance of flow cytometry can be understood through a multi-faceted approach. This includes its components, methodology, and diverse applications in immunology, oncology, and microbiology. Each of these domains benefits from the quantitative and qualitative analysis afforded by flow cytometry.
As we embark on a detailed exploration of this subject, the following sections will outline the methodology employed in flow cytometry studies, discuss key findings, and highlight future research directions.
Methodology
Study Design
When conducting studies using flow cytometry, researchers typically follow a structured study design. This involves defining the objective clearly, as well as selecting appropriate controls and variables relevant to the hypothesis. The design also dictates the sample size, which should be sufficient to ensure statistical significance.
Data Collection Techniques
The data collection process in flow cytometry involves several critical steps:
- Sample Preparation: Blood or tissue samples are carefully processed to isolate the cells of interest.
- Staining: Cells are incubated with fluorescently labeled antibodies, enabling specific identification based on their surface markers.
- Flow Analysis: The prepared samples are passed through the flow cytometer, where lasers excite the fluorescent markers. Detectors then capture the emitted light, allowing for the measurement of cell characteristics, such as size and granularity.
This systematic approach ensures that data collected is both reliable and reproducible, forming a solid foundation for subsequent analysis and interpretation.
Discussion
Interpretation of Results
Interpreting results from flow cytometry studies requires a comprehensive understanding of the correlations between cell populations and their implications in health and disease. It often involves using statistical software to analyze the data generated, helping to visualize and summarize findings. For instance, identifying subpopulations of immune cells can provide insights into immune disorders or tumor microenvironments.
Limitations of the Study
Despite its many advantages, flow cytometry does have limitations. Some potential challenges include:
- Complexity: The techniques and data can be intricate, requiring skilled personnel.
- Costs: The equipment and reagents can be expensive, limiting accessibility for some labs.
Future Research Directions
As the field of flow cytometry evolves, there are numerous avenues for future research. Advancements in technology could lead to:
- Enhanced Resolution: Improving the ability to analyze smaller populations of cells.
- Integration with Other Techniques: Combining flow cytometry with genomic or proteomic data to gain deeper insights into cellular behavior and disease mechanisms.
Furthermore, as personalized medicine continues to mature, flow cytometry will play an even larger role in tailoring therapies to individual needs.
Prelude to Flow Cytometry
Flow cytometry represents a pivotal advancement in cellular analysis. It functions not only as a powerful quantitative tool but also as a qualitative one, elucidating the complex characteristics of cells within a sample. Understanding flow cytometry is crucial for various disciplines, especially in areas like immunology, oncology, and microbiology. It harnesses the ability to analyze multiple parameters simultaneously, providing insights that are often unattainable through traditional methods.
The benefits of this technique are multifold. First, flow cytometry offers rapid analysis of thousands of cells in a matter of seconds. This speed is essential in clinical diagnostics and research where time is of the essence. Secondly, it provides high-resolution data, enabling precise differentiation between cell types based on multiple attributes. Furthermore, the ability to analyze cells in a fluid stream enhances the study of dynamic cellular processes in real-time.
However, delving into the realm of flow cytometry also necessitates an understanding of its limitations and the careful considerations required for its effective application. Factors such as instrument calibration, sample preparation, and data interpretation demand meticulous attention to ensure reliable outcomes. This section introduces flow cytometry as a valuable analytical approach, setting the stage for a deeper exploration of its principles and components.
Definition and Basic Principles
Flow cytometry is defined as a laser-based technology used to measure the physical and chemical characteristics of cells or particles in a fluid as they pass through a laser beam. This technology counts and analyzes the size, complexity, and fluorescent characteristics of cells. Flow cytometers utilize a technique termed hydrodynamic focusing, which aligns particles in a single file to intersect with the laser beam, allowing for the detection of emitted signals.
The basic principles underlying flow cytometry involve light scattering and fluorescence. When a cell passes through the laser beam, it scatters light in different directions. This scattered light is categorized into forward scatter, which correlates to cell size, and side scatter, which provides information about cell complexity or internal granularity. Additionally, fluorescent dyes can be attached to specific cell components, enabling the analysis of specific markers on the cells' surface or within their interior.
Historical Context
The origins of flow cytometry date back to the 1960s. At that time, scientists were exploring techniques to analyze cells in a fluid medium efficiently. The groundbreaking work of Leonard Herzenberg and colleagues led to the creation of the first flow cytometer at Stanford University. This device was revolutionary as it allowed for the simultaneous measurement of multiple parameters in single cells, thereby laying the foundation for future advancements in the field.
As the technology evolved, the development of more sophisticated laser systems and detection mechanisms significantly improved sensitivity and data quality. The introduction of fluorescently labeled antibodies further enhanced the ability to distinguish specific cell populations based on surface markers. Today, flow cytometry is a standard technique in many laboratories, facilitating research in diverse areas including immunology and cancer biology. Its role continues to expand, making a lasting impact on both basic research and clinical applications.
Components of a Flow Cytometer
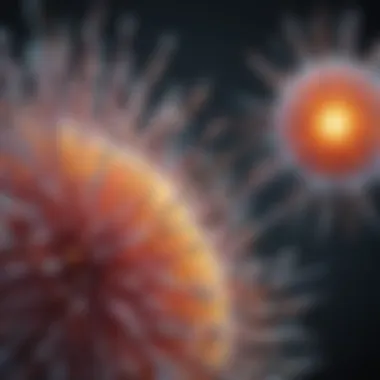
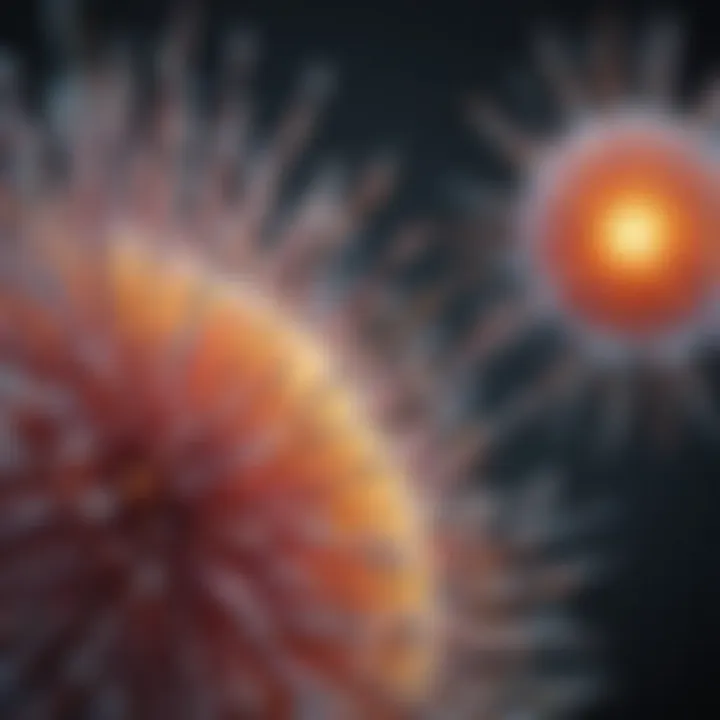
Flow cytometers are complex instruments that serve critical roles in cellular analysis. Understanding the components of a flow cytometer is essential for appreciating how this technology functions. Each component plays a vital part in the overall effectiveness and specificity of the analysis. In this section, we will examine the laser systems, optical filters, detectors, and fluidics system, detailing their importance and implications for research and clinical applications.
Laser Systems
Lasers are the primary excitation source in flow cytometry. Their ability to produce focused beams of light allows for precise measurement of cellular components. The selection of lasers usually depends on the unique fluorescent dyes used in an experiment. For instance, different lasers emit light at various wavelengths, optimizing the fluorescence of specific markers attached to cells. Common laser types include Argon, HeNe, and solid-state lasers.
The importance of laser systems cannot be overstated. They determine the resolution and sensitivity of the measurements. A well-calibrated laser can distinguish between closely related cell types, which is crucial for studies in immunology and oncology. Moreover, advances in laser technology continue to enhance sensitivity, enabling researchers to identify rare cell populations more effectively.
Optical Filters
In a flow cytometer, optical filters serve as intermediaries. After the laser light excites the fluorescing cells, optical filters isolate specific wavelengths of emitted light. This is crucial for accurately detecting the signals from different fluorescent tags, which are typically used to label various cellular components or molecules.
Two main types of filters are involved: bandpass and longpass filters. Bandpass filters allow only a specific range of wavelengths to pass through, while longpass filters focus on longer wavelengths, blocking shorter ones. The strategic placement and selection of these filters significantly impact the precision of data collection. Improper filtering can lead to data artifacts, potentially skewing results. Thus, careful consideration of optical filters is vital for obtaining reliable cytometric data.
Detectors
Detectors capture the light that passes through the optical filters. The most common detector types in flow cytometry are photomultiplier tubes (PMTs) and avalanche photodiodes (APDs). The choice of detector influences the dynamic range and sensitivity of the measurements.
Detectors convert light signals into electrical signals that can be quantified. High-quality detectors are sensitive enough to register even dim signals from rare events, such as apoptotic cells or stem cells. As technology progresses, newer types of detectors are being developed, promising not only better sensitivity but also faster data acquisition rates.
Fluidics System
The fluidics system is the backbone of the flow cytometer. This component is responsible for transporting cells in a hydrodynamically focused manner through the laser beam. The design of the fluidics system ensures that cells pass single file through the detection area, which is crucial for accurate measurements.
A well-functioning fluidics system minimizes issues like clogs or cell shear, which can impact measurement accuracy. Innovations in fluidics technology, such as microfluidics, have opened new avenues for more efficient cell sorting and faster processing times. Such advancements enhances the potential for high-throughput analysis, vital in busy labs and clinical settings.
Note: Each component’s reliability directly affects the overall performance of a flow cytometer. A breakdown or inefficiency in any area can compromise the quality of data generated.
In summary, the components of a flow cytometer—laser systems, optical filters, detectors, and fluidics systems—are interconnected and crucial for successful cellular analysis. Understanding how each part contributes to the overall capability of flow cytometry will help researchers and clinicians leverage this technology effectively.
Applications in Immunology
Flow cytometry plays a crucial role in immunology, offering precise analysis and characterization of immune cells. This technique provides insights into cell population dynamics, which is vital for understanding the immune response in various health conditions. By leveraging flow cytometry, researchers can gain a deeper understanding of both adaptive and innate immunity, enhancing our approach to disease prevention and treatment. Moreover, it enables better diagnostics and monitoring of immune-related disorders, thus underlining its significance in modern immunological studies.
Cell Population Analysis
Analysis of cell populations is one of the primary applications of flow cytometry in immunology. The technique allows scientists to distinguish between different types of cells based on specific surface markers. This is particularly important for identifying subsets of lymphocytes, such as CD4+ and CD8+ T cells, which play distinct roles in immune function.
Through the use of fluorescently labeled antibodies, flow cytometry generates data on the expression of various proteins on cell surfaces. This capability enables not only the identification of cell types but also provides quantitative information regarding their relative abundance in a sample. The ability to analyze multiple markers simultaneously enhances the complexity and depth of the analysis, allowing for a more nuanced understanding of immune cell interactions.
Cytokine Detection
Cytokines are essential signaling molecules in the immune system, influencing cell communication and immune response regulation. Flow cytometry facilitates the detection of cytokines at the single-cell level, offering insights into the functional status of immune cells. This application is particularly valuable in studying cytokine production during immune responses, such as those occurring during infections or autoimmune diseases.
By using intracellular staining techniques, flow cytometry allows researchers to measure cytokine levels within individual cells after activation. This provides a clearer picture of the immune landscape and can reveal important information about the pathophysiology of disease states. Moreover, understanding cytokine profiles may help in the development of targeted therapies aimed at modulating immune responses to achieve better clinical outcomes.
Applications in Oncology
Flow cytometry has emerged as a pivotal technological advancement in oncology, bolstering the understanding of cancer biology and facilitating enhanced patient management. Its ability to provide quantitative data on tumor cells allows for a detailed analysis of cellular phenotypes and functional status, thus aiding in the development of targeted therapies. Through flow cytometry, oncologists can analyze not only the tumor microenvironment but also the individual characteristics of cancer cells, leading to more informed diagnostic and therapeutic decisions.
Tumor Cell Analysis
The analysis of tumor cells using flow cytometry primarily focuses on characterizing the diverse populations of cells within a tumor. This characteristic is crucial for understanding tumor heterogeneity, which can influence treatment effectiveness. Tumor cells exhibit various markers on their surfaces, which can be detected and quantified through this method.
In practice, flow cytometry allows for the analysis of multiple parameters in a single cell simultaneously. This includes different surface antigens, internal proteins, and even cell cycle status. The following are some significant benefits of tumor cell analysis using flow cytometry:
- Identification of Cancer Stem Cells: Cancer stem cells are often resistant to standard treatments. Flow cytometry helps in identifying these cells based on their unique markers.
- Drug Sensitivity Testing: By examining how different tumor populations respond to chemotherapy, personalized treatment plans can be developed.
- Tumor Microenvironment Assessment: Analyzing immune cell interactions within the tumor can provide insights into immune evasion mechanisms.
"Flow cytometry is essential in the comprehensive characterization of tumor cells, unveiling insights that drive personalized cancer therapies."
Monitoring Treatment Response
Monitoring treatment response is another crucial application of flow cytometry in oncology. As therapies become increasingly targeted, understanding how patients respond to treatment is of utmost importance. Flow cytometry provides a rapid and accurate means to assess changes in tumor cell populations over time.
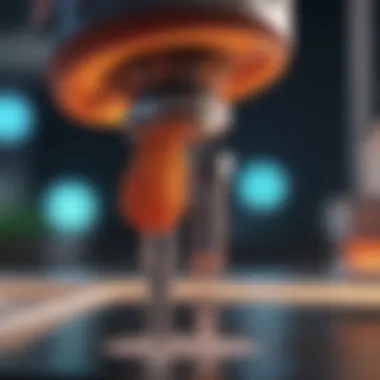
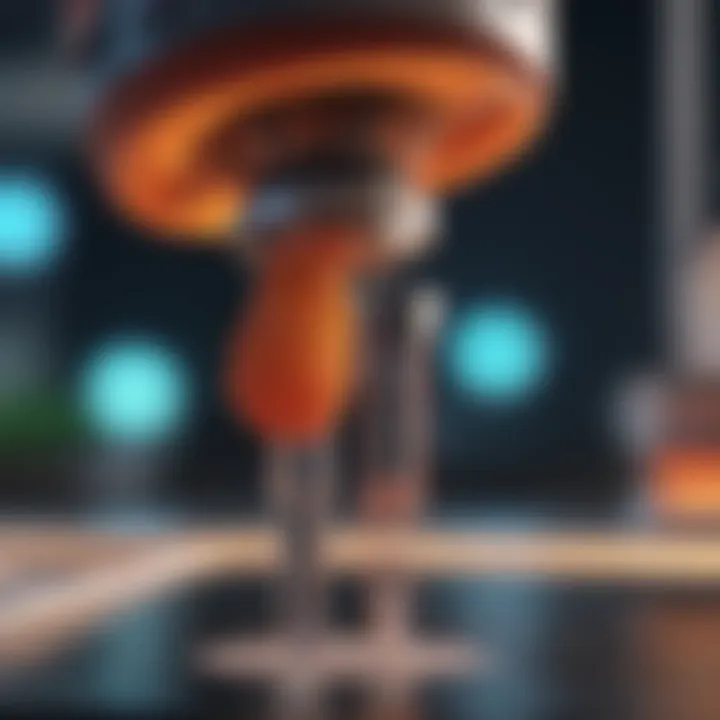
This aspect involves the longitudinal tracking of specific markers associated with treatment effects. For instance, reaching a quantitative decrease in specific tumor markers indicates treatment efficacy. The ability to monitor efficacy in real time allows for timely adjustments to treatment regimens, sparing patients from ineffective therapies that may cause unnecessary side effects. Some important considerations include:
- Quantitative Analysis: Real-time data can measure changes in cell populations, offering a clear picture of treatment progression or resistance.
- Early Detection of Resistance: Monitoring may reveal early signs of treatment resistance, prompting a shift in the therapy approach.
- Influence on Clinical Trials: Flow cytometry results can provide critical data points in clinical trials, particularly when assessing novel therapies or combination treatments.
Applications in Microbiology
Flow cytometry has become an invaluable tool in the field of microbiology. This technique allows researchers to analyze and quantify bacteria at a cellular level. The precision and efficiency it offers are indispensable in understanding microbial dynamics, especially in the context of human health, disease, and environmental factors.
Bacterial Identification
The ability to identify bacteria rapidly and accurately is crucial in microbiology. Flow cytometry facilitates this process by allowing for multiparametric analysis. Researchers can utilize flow cytometers to differentiate bacterial species based on their size, granularity, and fluorescence characteristics. This means that specific labeling techniques can be employed, using fluorescent tags to bind to unique bacterial markers. In doing so, it isolates bacteria from mixed cultures, helping identify pathogens quickly in clinical settings.
Furthermore, flow cytometry can assess viability. By using specific dyes that penetrate only dead cells, researchers can distinguish live bacteria from dead ones. This is especially useful in infection studies where live bacteria need to be monitored.
"Flow cytometry has revolutionized microbial analysis. It opens pathways to understanding complex ecological interactions among bacterial species."
Antimicrobial Resistance Studies
Antimicrobial resistance is a growing concern in the medical community. With the rise of resistant strains, the need for effective detection methods is urgent. Flow cytometry aids in these studies by enabling researchers to analyze the response of bacterial populations to various antimicrobial agents.
Through this method, scientists can quickly determine the minimum inhibitory concentration (MIC) of antibiotics. This is achieved by measuring changes in bacterial fluorescence over time after exposure to antibiotics. Such insights can inform treatment strategies for infections caused by resistant bacteria.
In summary, flow cytometry plays a vital role in enhancing our understanding of microbiological challenges. Its applications in bacterial identification and antimicrobial resistance studies are significant for both research advancements and clinical diagnostics.
Role in Personalized Medicine
Personalized medicine is an emerging approach that aims to tailor medical treatment to individual characteristics of each patient. Flow cytometry plays a crucial role in this context, enhancing the precision of therapies and improving patient outcomes. This section will delve into how flow cytometry influences personalized medicine by providing insights into immune profiling and cellular dynamics.
Tailoring Treatments
Flow cytometry allows for detailed analysis of cell populations in a patient's sample. By evaluating specific markers on the surface of cells, healthcare professionals can identify patient-specific characteristics. For example, in oncology, understanding the unique profile of tumor cells can lead to more effective treatments. Physicians can ascertain whether a patient’s tumor expresses certain antigens, allowing for targeted therapies such as monoclonal antibodies or immune checkpoint inhibitors.
Moreover, flow cytometry facilitates the identification of biomarkers that predict treatment response. This is especially significant in the field of cancer immunotherapy, where knowing if a patient is likely to respond to a specific treatment can save time and resources, ultimately leading to a more effective treatment plan tailored to that individual.
Flow cytometry enhances the potential of treatments by ensuring they align accurately with patient-specific characteristics. This can lead to significant improvements in clinical efficacy.
Prognostic Indicators
Another important aspect of flow cytometry in personalized medicine is its ability to provide prognostic indicators. By analyzing the types and status of immune cells present in a patient's blood or tissue, healthcare providers can gain insights into disease progression and patient survival. For instance, in chronic lymphocytic leukemia (CLL), flow cytometry can evaluate levels of specific surface markers predictive of patient longevity.
Understanding these indicators helps clinicians make better-informed decisions concerning treatment strategies. Patients identified with high-risk markers may require more aggressive therapy, while others may opt for less intensive treatment based on their flow cytometry profiles.
Integrating Flow Cytometry with Other Techniques
Integrating flow cytometry with other techniques enhances our capacity for cellular analysis. This multifaceted approach allows researchers to gain more precise insights into cellular behavior and interactions. By combining flow cytometry with other analytical methods, one can validate findings and explore complex biological questions that single methods may not address alone. The result is more robust data that supports informed conclusions in research and clinical applications.
Genomic Analysis
Genomic analysis allows for a deeper understanding of genetic content within cells. When combined with flow cytometry, this analysis can provide significant insights into the relationship between specific gene expression and cellular characteristics. For instance, a researcher can use flow cytometry to sort cell populations based on particular surface markers and then analyze gene expression patterns within those sorted cells. This approach is invaluable in studying diseases, such as cancer, where genetic mutations may influence cell behavior.
The benefits of integrating genomic analysis with flow cytometry include:
- Improved identification of potential biomarkers for diseases.
- Enhanced understanding of cellular responses to therapies at the genetic level.
- Facilitated discovery of new therapeutic targets by linking cellular phenotypes with genotypes.
Aligning flow cytometry with genomic analysis strengthens research findings. This combination underscores the intricate relationship between genetic and phenotypic expressions, contributing to nuanced understanding.
Proteomics
Proteomics focuses on the study of proteins, their structures, and functions within biological systems. Integrating flow cytometry with proteomics provides a comprehensive view of cellular activities. Flow cytometry can analyze proteins on the cell surface or within the cells through specific labeling techniques. This is important for quantifying protein expressions and understanding cellular signaling pathways.
The integration offers several advantages:
- Quantitative assessment of protein levels across heterogenous cell populations.
- Insights into post-translational modifications that might affect protein functions.
- Enhanced ability to correlate protein data with specific cell populations, leading to better understanding of cellular mechanisms.
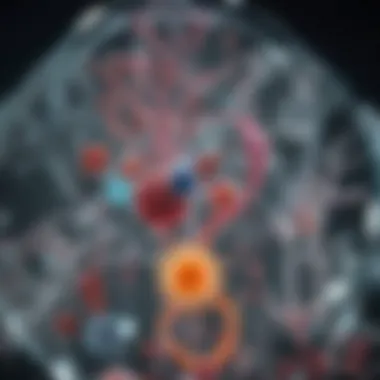
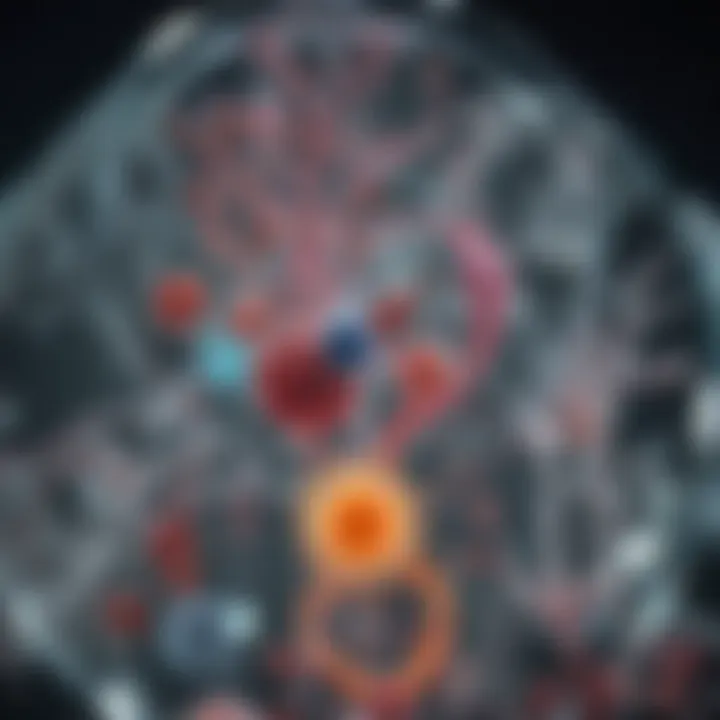
In summary, combining flow cytometry with proteomics yields a powerful toolkit for researchers. This integration leads to a more complete picture of cellular dynamics, facilitating advancements in biology and medicine.
Challenges in Flow Cytometry
Flow cytometry represents a cutting-edge tool in cellular analysis, yet it faces notable challenges that impact its effectiveness and reliability. Understanding these challenges is crucial for researchers and clinicians alike, as they can significantly influence experimental outcomes and the interpretation of results. The main elements under consideration in this section include technical limitations and data interpretation issues. Addressing these factors is imperative for maximizing the potential of flow cytometry in both research and clinical applications.
Technical Limitations
Technical limitations in flow cytometry encompass a range of aspects which can hinder the accuracy and precision of results. Here are some key elements to consider:
- Instrument Sensitivity: Each flow cytometer has a specific range of detectable events. Certain low-abundance populations may not be easily identifiable due to instrument sensitivity issues. This can be particularly critical in studies involving rare cell types, such as circulating tumor cells.
- Fluorochrome Selection: The choice of fluorochromes is essential for effective multi-parameter analysis. Some colors may overlap in emission spectra, leading to spectral compensation complications. If not adequately addressed, this overlap can skew analytical results and interpretations.
- Sample Preparation: Inadequate sample preparation can introduce artifacts and affect cell viability. Factors such as the time between sample collection and analysis, as well as storage conditions, play a significant role in maintaining sample integrity.
"Understanding the instruments and ensuring proper sample handling are vital for obtaining valid flow cytometry data."
- Throughput Limitations: While flow cytometry enables rapid analysis, very large sample sizes can lead to bottlenecks. The capacity of flow cytometers to process samples can be a limiting factor when dealing with extensive patient cohorts in clinical settings.
By acknowledging these technical limitations, researchers can devise strategies to mitigate potential issues, thus improving the robustness of flow cytometry analyses.
Data Interpretation Issues
Data interpretation is crucial in flow cytometry, yet it presents several challenges that can obscure findings:
- Complex Data Sets: Flow cytometry generates vast amounts of data, often involving multiple parameters simultaneously. The sheer volume and complexity can create difficulties in data analysis and visualization. Users must be proficient in using software tools to correctly analyze and represent this data.
- Biological Variability: Cellular responses can vary significantly between samples. Factors such as patient health status, sample processing, and experimental conditions can introduce variability. This can complicate comparisons and conclusions drawn from experimental data.
- Statistical Considerations: Many users may lack an adequate background in statistics, which is vital for interpreting flow cytometry data. Decisions regarding gating strategies and statistical tests impact the robustness of conclusions. Incorrect analytical approaches may lead to misleading interpretations of the biological significance of results.
In summary, a clear understanding of these data interpretation issues is essential. Researchers must be cautious in their analyses, ensuring that appropriate methods are applied to obtain trustworthy conclusions.
Addressing these challenges enhances the utility of flow cytometry, leading to more accurate and meaningful results in both basic and clinical research.
Future Directions of Flow Cytometry
The increasing complexity of biological and clinical questions demands continual innovation in flow cytometry. This section explores not only the technological advancements shaping the field but also the expanding applications that demonstrate flow cytometry's evolving relevance.
Technological Advancements
Over the past few years, advancements in flow cytometry technology have markedly improved its capabilities. Multicolor flow cytometry has become a powerful tool, enabling the simultaneous analysis of numerous parameters within a single cell. This allows for a more nuanced understanding of cellular heterogeneity, essential in cancer research and immunological studies.
Recent developments include the integration of microfluidics, which enhances the efficiency of sample processing. Microfluidic devices allow for precise manipulation of small volumes of fluids, reducing reagent waste and improving analysis speed. Additionally, the advent of imaging flow cytometry combines the principles of flow cytometry with high-resolution microscopy, offering exquisite detail on cellular morphology. These technologies collectively broaden the scope of information obtainable from flow cytometric analyses.
Moreover, the introduction of AI and machine learning tools plays a crucial role in data analysis. These tools can handle large datasets efficiently, allowing for advanced pattern recognition and predictive modeling. By automating complex data interpretation tasks, researchers can now focus on generating biologically relevant insights rather than being bogged down by data management.
Expanding Applications
Flow cytometry's applications continue to expand, firmly establishing it as a versatile tool across diverse research areas. Its role in clinical diagnostics is particularly noteworthy, helping in the identification of hematological malignancies through precise analysis of blood and bone marrow samples. Furthermore, flow cytometry is pivotal in vaccine development, where it assists in evaluating immune responses to various antigens.
In the field of stem cell research, flow cytometry aids in the characterization and isolation of stem cells. The ability to identify specific cell surface markers allows researchers to sort stem cells efficiently, advancing understanding of cellular development and therapies. Additionally, flow cytometry is gaining momentum in microbiome studies, enabling researchers to analyze the composition and functionality of microbial communities in health and disease.
Flow cytometry continues to evolve, driven by technological innovations and an expanding landscape of applications, presenting new opportunities for deeper biological understanding and clinical breakthroughs.
The future of flow cytometry lies in its integration with complementary techniques, such as genomic and transcriptomic analyses. This interdisciplinary approach will enhance comprehensive cellular profiling, ultimately transforming biomedical research and personalized medicine. As we look ahead, embracing these advancements and applications is crucial for fostering new scientific discoveries.
The End
The conclusion section is essential in summarizing the insights gleaned from the extensive discussions about flow cytometry applications. This article has traced the journey of flow cytometry from its foundational principles to its far-reaching implications across various fields. The understanding of flow cytometry is not just an academic exercise but a critical element that drives innovation, research, and clinical practices.
In examining the applications in immunology, oncology, and microbiology, we highlight how flow cytometry serves as a vital analytical tool. It allows researchers to assess complex cellular populations and mechanisms efficiently. The benefits of leveraging flow cytometry are multiple:
- Enhanced Precision: Flow cytometry provides a high level of accuracy in measuring cellular characteristics, essential for both research and clinical applications.
- Versatility: The technique’s adaptability across different disciplines enables its use in various complex research scenarios.
- Impact on Personalized Medicine: By tailoring treatments to individual cellular profiles, flow cytometry significantly improves patient outcomes.
Considering these advantages, one must also recognize the considerations surrounding the conclusions drawn from flow cytometry data.
Summary of Key Points
- Flow cytometry is a powerful technique that enables precise analysis of cellular characteristics.
- It has diverse applications in immunology, oncology, and microbiology, providing critical insights for each field.
- Personalized medicine benefits greatly from the detailed cellular data that flow cytometry can provide.
- Understanding the technical limitations and data interpretation of flow cytometry is crucial for its effective application.
- Future enhancements in technology are likely to bridge existing gaps and expand its applications further.
Implications for Future Research
Future research in flow cytometry has promising potential. As technologies evolve, one implication is the integration of advanced imaging techniques with conventional flow cytometry. This could enhance the resolution of analyses, allowing scientists to visualize cellular interactions in real-time. Other avenues for exploration include:
- Automation: The move towards automated systems could streamline processes, increase throughput, and reduce human error in data collection and analysis.
- Data Analytics: Advances in machine learning and data analysis techniques can enable deeper insights from flow cytometry data, potentially uncovering patterns that were previously undetectable.
- Cross-disciplinary Uses: Continued collaboration among disciplines can lead to innovative applications beyond the current known fields.
In summary, the conclusion encapsulates the vital role of flow cytometry in scientific research and clinical diagnostics. Its relevance continues to grow, ushering in new possibilities for understanding complex biological systems.