Understanding Weather Regions: A Scientific Perspective
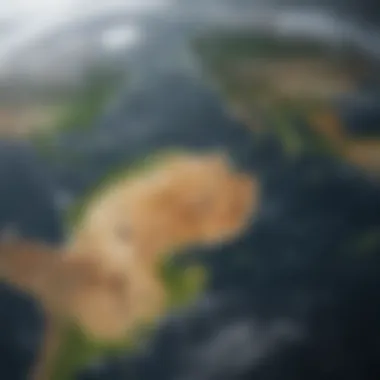
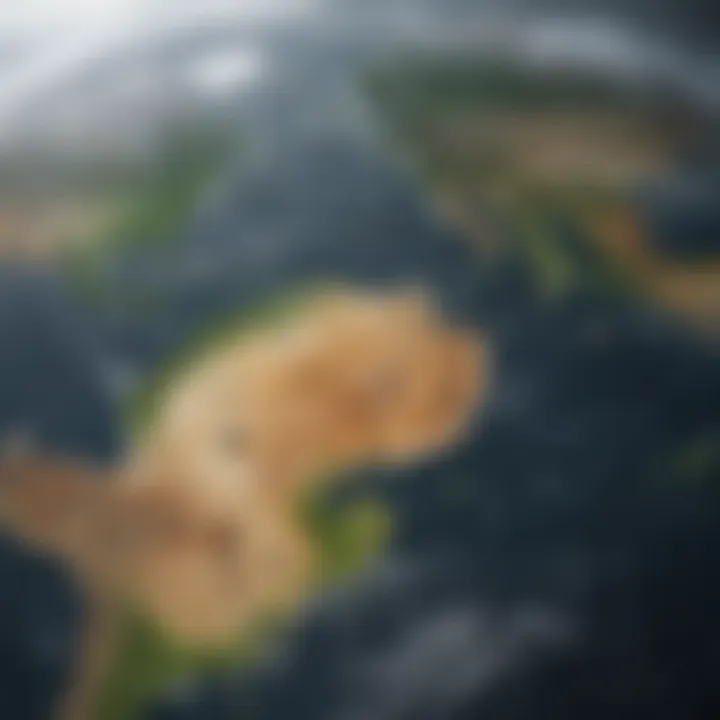
Intro
Grasping the complexities of weather regions requires not only an understanding of climatic characteristics but also an appreciation for the methodologies that define them. Each weather region on our planet exhibits unique patterns influenced by diverse factors, including geographical features, proximity to oceans, and atmospheric conditions. This article will offer insights into the classification of weather regions, exploring not just what they are, but why they matter.
We will identify how weather phenomena correlate with ecosystem dynamics and human activities. Today's challenges, from climate change to weather-related disasters, necessitate a thorough understanding of how different regions function under varying climatic conditions.
Methodology
Study Design
The study of weather regions involves a multi-faceted approach incorporating both qualitative and quantitative research methods. These methods include historical climate data analysis, field observations, and geographic information system (GIS) technologies. Combining these approaches allows for a more nuanced understanding of regional weather patterns and their implications.
Data Collection Techniques
Data collection is crucial in the assessment of weather regions. Various techniques are adopted:
- Satellite Imagery: Satellites provide comprehensive data on temperature, humidity, and cloud cover across large areas.
- Meteorological Stations: Ground-based stations collect real-time data on various meteorological variables, contributing to local weather forecasting and climate analysis.
- Ocean Buoys: These instruments measure sea surface temperatures and wave conditions, influencing coastal weather patterns.
- Historical Records: Accessing archives of historical weather data permits researchers to identify long-term trends and anomalies in climate behavior.
Understanding these methodologies is essential for accurately categorizing weather regions and their characteristics.
Discussion
Interpretation of Results
Through integrating diverse data sources, researchers can interpret how specific climatic factors define each weather region. For example, arid regions exhibit distinct temperature variations and precipitation patterns when compared to tropical climates. The analysis will shed light on how ecosystems adapt to these varying conditions.
Limitations of the Study
While this examination shines light on the dynamics of weather regions, limitations exist. Data gaps may arise due to the uneven distribution of weather stations or technological constraints with satellite data. Additionally, models predicting future conditions might not accurately capture the nuances of localized weather phenomena.
Future Research Directions
Understanding evolving weather patterns will be critical, especially in light of climate change. Future research should focus on:
- Enhanced satellite technology for improved data accuracy.
- Greater integration of modeling techniques that incorporate human activity and its impacts on climatic changes.
- Longitudinal studies that monitor changes in ecosystems as climates evolve.
Overall, an interdisciplinary approach to meteorology will enrich our comprehension of weather regions and support the development of effective climate adaptation strategies.
Preface to Weather Regions
Understanding weather regions is essential in the field of meteorology. It allows us to categorize and analyze diverse climates across the globe. This classification influences various aspects of human life and natural ecosystems. By comprehending the different weather regions, researchers and students can deepen their appreciation of climatic variations and their implications.
Definition and Importance
Weather regions are defined by distinct climatic patterns that result from various factors, including latitude, geography, and ocean currents. These regions help scientists understand and predict weather phenomena, assess environmental risks, and plan for agricultural practices. Recognizing these categories enhances the ability to respond to climate-related issues.
The importance of classifying weather regions lies in its application. It assists in disaster management, ensuring that communities can prepare for extreme weather events, such as hurricanes or droughts. Furthermore, the study of weather regions provides insights into biodiversity, as certain climates foster specific ecosystems.
Historical Perspectives on Climate Classification
Historically, the classification of weather regions can be traced to early scientists and explorers who documented climatic variations as they traveled. Notable figures like Aristotle made early observations. However, modern classification systems emerged in the mid-20th century, often based on detailed studies and statistical analysis.
As science progressed, classifications have been refined. Today, the Köppen climate classification system is one of the most widely used. It categorizes climates based on temperature and precipitation, making it easier to understand the intricate relationships between weather and ecological systems. This evolution of classification reflects the growing sophistication in meteorological science and its applications to climate change.
Basic Principles of Weather Patterns
Understanding weather patterns is fundamental in meteorology. This section outlines the core elements that govern weather, laying a scientific foundation for the subsequent classifications of weather regions. Recognizing the principles of weather patterns aids not only in accurate forecasting but also informs numerous practical applications in agriculture, urban planning, and environmental conservation. With climate change shaping our world, comprehending these principles becomes ever more crucial.
Atmospheric Circulation
Atmospheric circulation refers to the large-scale movement of air in the Earth's atmosphere, driven primarily by the unequal heating of the Earth’s surface by the sun. This phenomenon is crucial in forming various weather regions. Differences in temperature create pressure gradients, leading to wind patterns. These winds can be classified into three main belts: the polar easterlies, prevailing westerlies, and trade winds.
In addition, the Coriolis effect, a result of Earth's rotation, influences the direction of wind flow, causing moving air to curve. This results in distinct weather systems, such as cyclones and anticyclones, which are instrumental in determining local climates. Understanding atmospheric circulation is vital for predicting weather patterns across various regions, highlighting its significance in climate studies.
Heat Distribution and Its Effects
Heat distribution across the planet is not uniform. Various factors, such as latitude, altitude, and proximity to bodies of water, affect how heat is absorbed and released. Areas closer to the equator receive more direct sunlight year-round, resulting in warmer climates. In contrast, polar regions experience minimal sunlight duration and intensity, leading to colder conditions.
The uneven heating of the Earth creates temperature differences, influencing atmospheric pressure and storm formation. For instance, warmer air holds more moisture, contributing to rainfall patterns, while colder air can lead to drier conditions. This dynamic interaction between heat distribution and weather phenomena is significant. It shapes ecosystems, agricultural practices, and even human settlements.
"A profound understanding of heat distribution is essential for predicting local and global climate changes."
In summary, the basic principles of weather patterns are essential for discerning the complexities of climate regions. They provide insight into the mechanisms that drive weather systems, which is vital for various fields including environmental science and urban planning.
Classification of Weather Regions
The classification of weather regions is a fundamental aspect of meteorology. Understanding these classifications helps in comprehending global climate variations and their implications. When we examine how different regions respond to atmospheric phenomena, we see a vast array of weather behaviors influenced by geographic, oceanic, and seasonal factors. This classification can aid in predicting weather patterns, assessing the risks of natural disasters, and studying the impacts of climate change on diverse ecosystems.
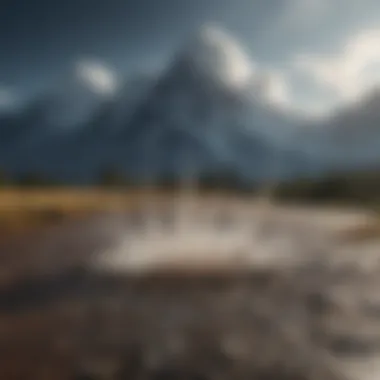
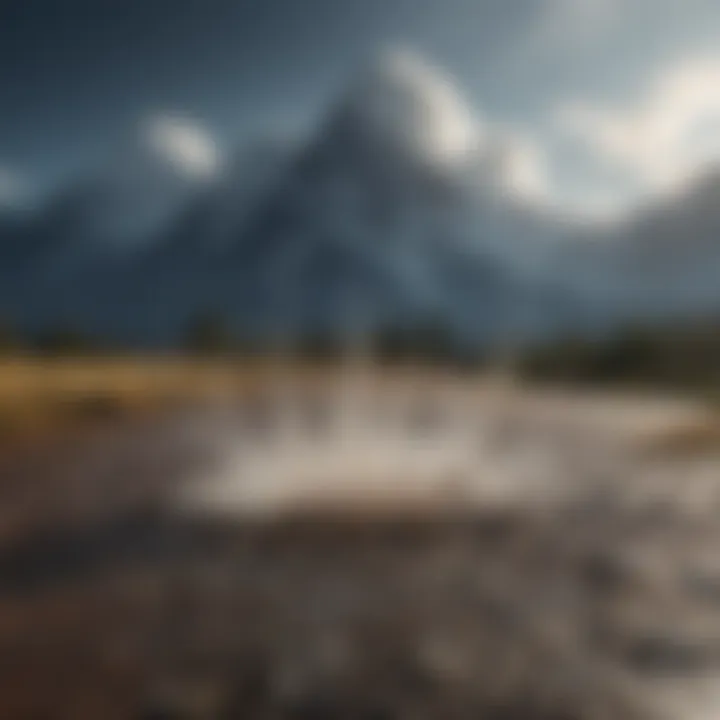
Weather regions are categorized based on distinct climatic characteristics including temperature, precipitation, and prevailing winds. Recognizing these divisions is vital for various sectors like agriculture, disaster management, and urban planning. By comprehensively understanding weather boundaries, sectors can adapt practices that align with regional climatic conditions, ultimately fostering sustainability and resilience.
Tropical Regions
Tropical regions, typically located between the Tropic of Cancer and the Tropic of Capricorn, are characterized by warm temperatures and significant rainfall throughout the year. These regions exhibit a phenomenon known as the Intertropical Convergence Zone (ITCZ), where trade winds from the Northern and Southern Hemispheres meet, creating conditions for regular precipitation. The amount of sunlight and warmth in tropical areas fosters lush vegetation and rich biodiversity.
Common climatic features include:
- High average temperatures: Often above 18°C throughout the year.
- Abundant rainfall: Annual averages can reach 2000 mm or higher.
Such conditions make tropical areas crucial for ecosystems and human livelihoods. However, they also render these regions vulnerable to extreme weather events like hurricanes and flooding, emphasizing the need for effective disaster response mechanisms.
Temperate Regions
Temperate regions are situated between the tropics and polar areas. These regions experience a mix of warm and cold temperatures, with distinct seasonal variations. The seasons can lead to significant shifts in weather patterns, impacting agriculture, natural habitats, and daily life.
Key features of temperate regions include:
- Four distinct seasons: Spring, summer, fall, and winter.
- Moderate rainfall: Average precipitation varies widely depending on the area, often between 500 mm to 1500 mm annually.
These seasonal changes influence agriculture significantly. For example, the growing season is limited to the warmer months, which impacts crop production and planning. Furthermore, the presence of extreme weather events like snowstorms or heatwaves can create challenges for communities and ecosystems alike.
Polar Regions
Polar regions, found near the poles of the Earth, are marked by extreme cold temperatures and ice-covered landscapes. The Arctic and Antarctic are prime examples of these regions, showcasing unique climatic conditions that substantially affect the global environment. Despite appearing harsh and inhospitable, polar regions hold significant geopolitical and ecological importance.
Characteristic features include:
- Permafrost: Ground that remains frozen year-round in many areas.
- Low precipitation levels: Often less than 250 mm annually, primarily in the form of snow.
The environmental challenges faced in polar regions are multifaceted. In addition to naturally occurring conditions, the impact of climate change is profound. Warming temperatures are leading to ice melting, altering habitats and threatening species that have adapted to these conditions over millennia. Understanding the polar climate is crucial for predicting global climate shifts and managing biodiversity.
Understanding weather classifications is essential for addressing climate challenges and facilitating informed decision-making in policy and environmental management.
Tropical Weather Regions
Tropical weather regions are crucial sections of our planet’s climatic landscape. Often characterized by warm temperatures and abundant rainfall, these areas serve as vital ecosystems and hold significant importance for global weather patterns. Understanding tropical weather is essential for comprehending broader climatic processes.
Characteristics and Climatic Features
Tropical regions are distinguished by their high temperatures, usually maintaining an average above 18°C (64°F) year-round. The key characteristic is the lack of seasons as experienced in temperate regions. Instead, there are wet and dry periods, influenced largely by monsoonal winds and ocean currents. Areas such as the Amazon Basin and the Congo River Basin exemplify this climate, with lush vegetation and rich biodiversity.
Humidity in tropical weather regions is generally high, due to proximity to the equator. This humidity fosters the growth of dense forests and unique wildlife, further extending the climatic richness of these areas.
Rainfall Patterns
Rainfall in tropical regions is typically abundant, with some areas receiving over 2000 mm of precipitation annually. The rain is not uniformly distributed; rather, it exhibits distinct wet and dry seasons. The wet season often correlates with stronger solar heating, which induces convectional rainfall.
Several factors impact rainfall patterns in tropical zones, including:
- Geographical location: Proximity to oceans and large bodies of water can enhance rain, as moisture-laden air is drawn inland.
- Topography: Areas with mountains can create orographic rainfall where moist air rises, cools, and condenses.
- Seasonal winds: Monsoons, prevalent in regions such as South Asia, bring sustained rainfall during certain months.
Impact on Biodiversity
Tropical weather regions are home to approximately 50% of the world's plant and animal species. This remarkable biodiversity is linked to the climate's capacity to support various habitats. The warm temperatures and plentiful rain foster the growth of tropical rainforests and coral reefs, both of which are critical for ecosystem balance.
The interplay between climate and biodiversity is evident in several ways:
- Niche Specialization: Species adapt to specific niches, taking advantage of the abundant resources in tropical areas.
- Ecosystem Services: Tropical ecosystems offer significant services, including carbon storage, which helps mitigate climate change impacts.
- Threats: Climate change poses risks to these regions, potentially altering weather patterns and disrupting the delicate balance of biodiversity.
"Tropical regions, with their ecological wealth, are essential for the health of our planet and provide crucial resources for human survival."
Temperate Weather Regions
Temperate weather regions represent key areas of our planet where moderate climates prevail. These regions showcase distinctive seasonal changes, which contribute to the diversity of life and human activities. Understanding temperate weather regions is essential not just from a climatic standpoint but also for its implications on ecosystems, agriculture, and regional economies.
The variability of seasons within temperate zones provides unique opportunities and challenges. The cycles of spring, summer, autumn, and winter strongly influence how both wildlife and human populations interact with their environment.
Seasons and Their Variability
The temperate regions experience a distinct set of seasons. Each season brings specific conditions, affecting temperature and precipitation. For instance, in summer, temperatures typically rise, leading to increases in evaporation and changes in vegetation growth patterns. In contrast, winter brings colder temperatures and often snow, influencing animal behavior such as migration and hibernation.
Variability in seasons ensures that no two years are identical. Climate anomalies, such as El Niño or La Niña, can lead to unexpected changes in temperature and precipitation; hence, the relevance of monitoring these patterns is crucial for research and practical applications.
"The impact of seasonal changes can be profound, influencing everything from agriculture to animal breeding cycles."
Weather Extremes
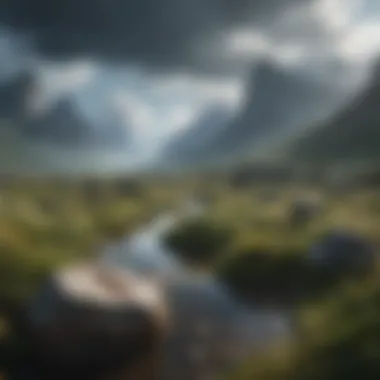
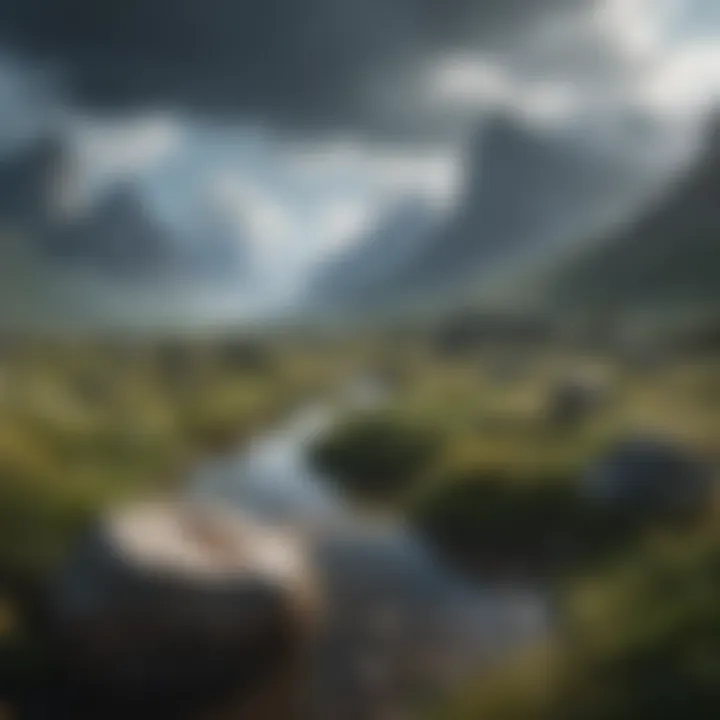
Within temperate regions, weather extremes can manifest in various forms. These may include intense storms, heat waves, and prolonged cold snaps. Such events arise from the interaction of different air masses, which frequently occurs in these climates.
For example, during late summer, heat waves may occur as warm air masses dominate, while transitioning to autumn, the likelihood of storms can increase due to changing pressure patterns. These extremes often affect infrastructure and public safety, requiring adaptive planning from urban and local governments to mitigate their impacts.
Agricultural Implications
Agriculture thrives in temperate regions due to the favorable climate and varied seasons. Crops can be diversified, allowing farmers to engage in practices that maximize yield throughout the year. Essentially, such regions facilitate both summer crops like maize and winter crops such as wheat.
However, the agricultural sector must also contend with the weather extremes previously mentioned. Farmers face risks from unexpected frost in spring or summer droughts that can diminish their crop outputs. Smart farming strategies, including season extension techniques and moisture retention practices, can help mitigate these risks.
In summary, temperate weather regions are of great significance. They affect biodiversity, influence agricultural practices, and determine the lives of many people. A deeper understanding of these regions and their dynamics is vital for research, policy-making, and sustainable development.
Polar Weather Regions
The study of polar weather regions holds significant importance within the broader context of climate science. These areas, located near the Earth's poles, showcase extreme climatic conditions that can profoundly influence global weather patterns. Understanding the unique characteristics of polar regions is vital, as they act as critical components in the Earth's climate system.
Climate Conditions and Features
Polar climates are distinct, characterized by long, harsh winters and short, cool summers. The average annual temperatures in these areas are often below freezing, particularly in the winter months. Areas like Antarctica and the Arctic have nearly permanent ice coverage, impacting local ecosystems profoundly.
- Temperature: Winter temperatures can plunge as low as -50 degrees Celsius, while summer temperatures might reach just above freezing.
- Precipitation: Precipitation is generally low, with most falling as snow. The sparse moisture leads to dry conditions, making the polar climate feel even colder.
- Daylight Variation: Polar regions experience significant variation in sunlight, with long periods of darkness in winter and nearly constant daylight in summer.
These climate conditions create a unique environment that supports specially adapted flora and fauna but also presents challenges for survival.
Environmental Challenges
The extreme conditions of polar weather regions pose various environmental challenges. Ecosystems are particularly sensitive to changes in climate. Some of the key challenges include:
- Melting Ice Caps: Global warming leads to ice cap melting, significantly disrupting marine life and habitats.
- Habitat Loss: As sea ice diminishes, species such as polar bears and seals face threats to their habitat and hunting grounds.
- Ecosystem Imbalance: Changes in temperature and the length of seasons can lead to mismatches in food availability and migration patterns.
Furthermore, human activities such as oil drilling and shipping routes also contribute to environmental stress in these delicate regions.
Impact of Climate Change
Polar regions are among the most affected by climate change. Their feedback mechanisms can amplify global warming effects, making them critical to study:
- Temperature Rise: The Arctic has warmed at more than double the global rate, which increases the risk of extreme weather events.
- Rising Sea Levels: The melting of glaciers and ice sheets contributes to rising sea levels, threatening coastal regions globally.
- Disruption of Weather Patterns: Changes in polar temperatures can shift atmospheric circulation, impacting weather patterns far from the poles.
As we see changes in polar weather regions, we witness how interconnected our planet is. The impact of these regions stretches far beyond their geographical boundaries, affecting climates and ecosystems worldwide.
The detailed examination of polar weather regions highlights their significance in understanding global climatic shifts and the urgent need for conservation efforts. Studying these regions not only provides insights into the Earth's climate but also emphasizes the interconnectedness of all ecological systems.
The Role of Ocean Currents
Ocean currents are pivotal in shaping global weather patterns. They act as massive conveyor belts, distributing heat and influencing climate conditions across various regions. Through this section, we will explore how ocean currents function and their far-reaching impacts on weather systems and atmospheric interaction.
Influence on Weather Systems
Ocean currents have a direct influence on weather systems. They help in regulating temperatures, which affects surrounding air masses. For instance, the Gulf Stream, a warm-current in the North Atlantic Ocean, raises the temperature of the air above it. This contributes to the milder climate seen in western Europe compared to eastern Canada, which is affected by colder currents.
- Warm currents generally lead to increased humidity and precipitation, while cold currents may suppress rain, creating drier conditions.
- The interaction of different currents can lead to complex weather phenomena, such as hurricanes forming over warm waters, which intensify as they move over them.
In addition, changes in ocean current patterns can signal shifts in weather conditions. For example, an alteration in the El Niño or La Niña phenomenon can disrupt normal weather patterns, leading to unusual rainfall or droughts in various parts of the world.
Interaction with Atmospheric Patterns
The connection between ocean currents and atmospheric patterns is significant. The air above the ocean is influenced by the water temperature, which in turn affects wind patterns.
- When warm air rises over warm ocean currents, it leads to low-pressure areas, fostering cloud formation and precipitation.
- Conversely, cold currents can result in high-pressure systems, pushing air downwards and leading to clearer skies.
This interaction is crucial for understanding the global atmospheric circulation. The trade winds, for instance, are deeply affected by oceanic conditions. They help in maintaining the circulation of air which is essential for weather systems worldwide.
In summary, the understanding of ocean currents is vital for developing accurate weather predictions and comprehending climatic shifts. By grasping how these currents influence atmospheric patterns, scientists can better anticipate weather-related events, preparing communities for potential impacts.
Ocean currents serve as a fundamental component in the puzzle of global climate regulation, underscoring the interconnectivity of Earth’s systems.
Microclimates: A Deeper Look
Microclimates represent the localized variations in climate across a small area. These variations are essential in understanding the dynamics of weather regions. They illustrate how factors like topography, vegetation, and human activity can lead to significant differences in climate and weather, even over short distances. Recognizing the importance of microclimates helps us understand broader climatic systems and their interplay with ecosystems and human practices. In urban settings, for example, the heat generated by buildings and vehicles can create much warmer conditions compared to nearby rural areas. Such insights have implications for agriculture, urban planning, and conservation efforts.
Definition and Formation
Microclimates are characterized by the specific weather conditions that differ from the surrounding area. They can form due to various reasons. For instance, hills can create shade and block wind, resulting in cooler temperatures in their shadowed areas. Similarly, bodies of water can moderate temperatures, leading to milder conditions nearby. Vegetation plays a crucial role too; forests can retain moisture and provide cooler environments compared to bare land.
In terms of formation, microclimates may develop from:
- Elevation Changes: Higher altitudes tend to have cooler climates.
- Proximity to Water: Lakes and oceans can influence local air temperature.
- Urban Development: Cities often experience the urban heat island effect.
- Soil Type and Vegetation: Different soil compositions and plant types can alter temperature and humidity levels.
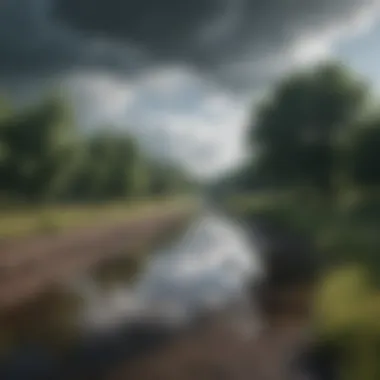
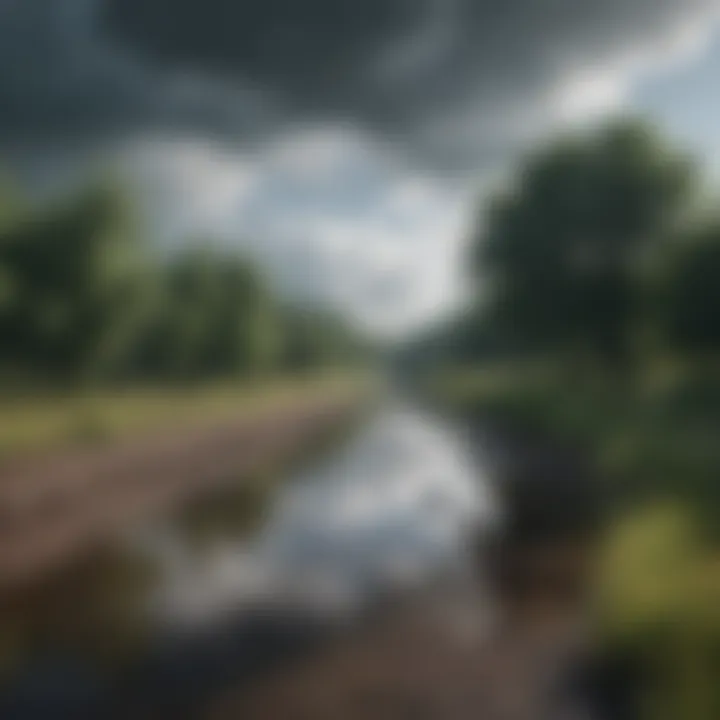
Factors Influencing Microclimates
Several factors influence the formation and characteristics of microclimates. These include:
- Topography: The layout of the land is crucial. Valleys may trap cold air, while hilltops can collect heat.
- Land Cover: Areas with dense vegetation are generally cooler and more humid compared to open fields.
- Wind Patterns: Wind direction and strength can impact temperature and moisture levels.
- Human Activities: Urbanization changes natural environments, often leading to increased temperatures and altered precipitation.
Microclimates are not static; they can evolve due to climate change. Changes in precipitation and temperature trends influence the distribution and types of vegetation, which in turn affects local climates. Understanding these changes can provide insights necessary for adapting urban planning, conservation strategies, and agricultural practices to suit evolving environmental conditions.
Effects of Climate Change on Weather Regions
Climate change is a pressing concern that affects weather regions across the globe. Understanding its effects helps in predicting future climate scenarios and their impacts on ecosystems and human activities. This section will analyze two critical aspects: changes in temperature and precipitation patterns, and shifts in weather regions. These topics are significant as they have profound implications for agriculture, biodiversity, and urban planning.
Changes in Temperature and Precipitation Patterns
The rising levels of greenhouse gases in the atmosphere have altered the global temperature balance. This increase in temperature is not uniform; some regions experience greater warming than others. For example, polar regions are warming at a rate much faster than the global average. The effect of this warming can lead to drastic changes in precipitation patterns.
- Increased Rainfall: Some areas may see more intense and frequent rainfall, leading to flooding and soil erosion.
- Drought Conditions: Conversely, other regions may suffer from prolonged droughts, which can decimate crops and affect water supply.
According to numerous climate models, regions that are traditionally considered wet or dry are beginning to exhibit significant changes. The shift in precipitation can affect local agriculture and water management. Consequently, understanding these shifts is crucial for planning effective responses to ensure food security.
"Climate change is not just about temperature rise; it's about the entire precipitation regime, impacting rivers, lakes, and water supplies."
As temperature and precipitation patterns change, they will disrupt local climates, creating challenges for regions that must adapt to new normals.
Shifts in Weather Regions
Climate change is not only affecting the weather patterns. It is fundamentally altering weather regions. Many scientists believe that if current trends continue, entire regions may transition into different climatic zones.
- Expansion of Dry Zones: Areas like southern Europe and the Mediterranean are predicted to become more arid. This change can affect biodiversity and water availability.
- Tropical Regions: The tropics may expand, leading to shifts in plant and animal distributions. Some species may not be able to adapt quickly enough, resulting in ecological imbalances.
The movement of weather regions is a serious concern. Regions that were once stable may face unexpected climates. Thus, it is vital for policymakers and scientists to monitor these changes. They must also prepare for the socio-economic consequences that may arise as people adjust to new climatic realities.
Understanding the effects of climate change on weather regions is essential for creating adaptive strategies that safeguard both natural ecosystems and human livelihoods.
Human Activities and Climate Impact
The intersection of human activities and climate impact is increasingly significant in understanding weather regions. As populations grow and industrial activities expand, the delicate balance of climatic factors is disrupted. This section examines the various ways that human actions contribute to climate variability, affecting weather patterns and regional characteristics. Recognizing this relationship is essential for developing sustainable strategies and mitigating adverse effects on the environment.
Urbanization and Its Effects
Urbanization plays a critical role in transforming weather regions. As cities expand, they create heat islands—areas where temperatures are consistently higher than surrounding rural areas due to human activity. The development of buildings, roads, and other infrastructure replaces natural vegetation, thereby reducing the land's ability to absorb heat and moisture.
Moreover, urbanization alters local wind patterns and precipitation. These changes can lead to increased storm intensity and altered rainfall distributions. For instance, cities like Los Angeles experience modified weather due to the presence of structures that redirect wind flow.
Key points on the effects of urbanization include:
- Increased air pollution, which can intensify greenhouse gases, leading to climate change.
- Changes in drainage systems which have implications for flooding; urban areas often face sudden and severe floods due to poor drainage planning.
- Alteration of natural habitats which affects local ecosystems and reduces biodiversity.
Understanding these effects is crucial in planning and designing urban areas that are resilient to climate impacts.
Agricultural Practices
Agricultural practices greatly influence weather regions and their climate. Farming techniques can lead to land degradation and soil erosion, which disrupts local ecosystems. For instance, monoculture—growing a single crop over a large area—can deplete soil nutrients, making the land less productive over time.
Additionally, agricultural practices can push the boundaries of weather regions by altering the local microclimate. The use of irrigation, for example, can change humidity levels and local temperatures. In regions where extensive irrigation is practiced, weather patterns can be significantly affected.
Important considerations in agricultural impact include:
- Use of fertilizers and pesticides which contribute to runoff, affecting water quality and aquatic systems.
- Deforestation for agricultural expansion, which impacts local climates and associated biodiversity.
- Need for sustainable agricultural practices that minimize environmental harm and enhance resilience against climate effects.
In summary, human activities, through urbanization and agricultural practices, have profound implications for weather regions. Recognizing these impacts can foster a more responsible approach toward environmental stewardship and climate action.
Closure
In summarizing the key elements of weather regions, we underline their critical role in understanding not only the planet's diverse climates but also the intricate interplay between weather phenomena and ecological dynamics. Weather regions are defined by distinct characteristics such as temperature variability, precipitation patterns, and seasonal changes. These elements influence ecosystems and everyday human activities, making it imperative to grasp their significance.
The importance of recognizing weather regions extends beyond academic interest. It informs agricultural practices, disaster preparedness, and urban planning. Assessing how climate change impacts these regions allows for adaptation strategies that can mitigate adverse effects. Ultimately, an enhanced understanding of weather regions fosters a more informed public, capable of responding to climatic shifts appropriately.
"Awareness of weather patterns can lead to better preparedness and resource management in the face of climate change."
Summary of Key Findings
Through our exploration, several key findings emerged:
- Diversity of Weather Regions: The globe hosts a variety of regions, each with unique climatic traits, from tropical to polar.
- Impact of Climate Factors: Atmospheric circulation and ocean currents significantly influence weather patterns in all regions.
- Microclimates: Localized climate variations can have profound effects on biodiversity and agriculture, highlighting the complexity of climate interactions.
- Human Influence: Urbanization and specific agricultural practices alter local weather patterns, necessitating thoughtful environmental management.
These findings not only highlight the complexity of weather science but also the necessity of understanding these topics in a rapidly changing environment.
Future Research Directions
Further research into weather regions should consider the following areas for deeper investigation:
- Technological Advances: The use of satellite data and artificial intelligence could enhance predictions and analyses of climate trends.
- Regional Climate Models: More localized models can help in understanding microclimates and their specific implications for ecosystems.
- Climate Change Impacts: Monitoring shifting weather patterns due to climate change across various regions will yield crucial insights for adaptation strategies.
- Public Health Implications: Understanding how weather regions affect the spread of diseases and environmental health can lead to better public health policies.