Understanding Stem Cell Engraftment: Mechanisms and Implications

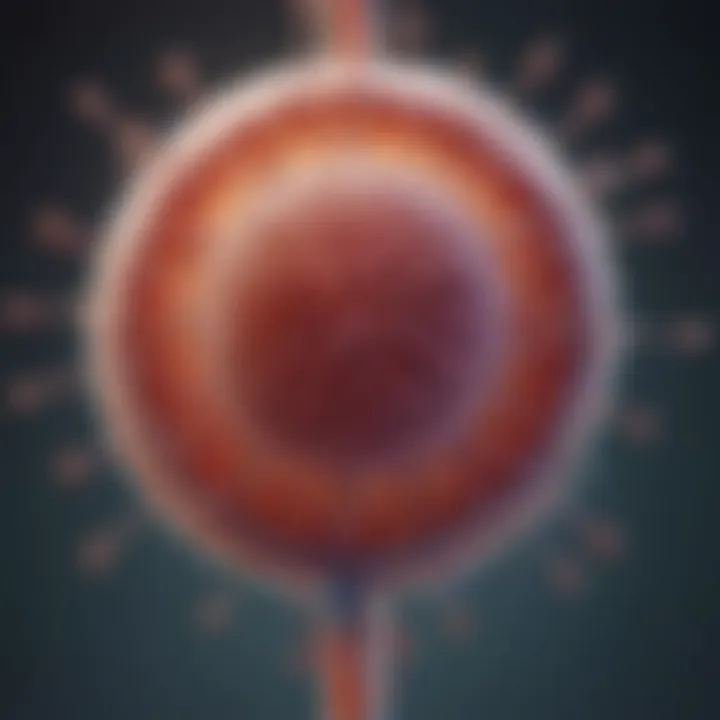
Intro
Stem cell engraftment is more than just a biological phenomenon; it is a critical component of regenerative medicine and a pivotal element in modern therapeutic strategies. The mechanisms that govern this intricate process are multifaceted and dependent on a variety of internal and external factors. Understanding how stem cells integrate into host tissue is essential for advancing stem cell therapies and enhancing patient outcomes.
In this exploration, we delve into the core mechanisms that facilitate successful engraftment, identify the pivotal factors influencing the process, and discuss the far-reaching implications that stem cell engraftment holds for the future of medicine. By breaking down the biological and cellular dynamics involved, we aim to provide a comprehensive framework that seeks to unravel the complexities that scientists and clinicians encounter in their pursuit of effective therapies.
As we navigate through this discussion, we shall also face the critical challenges associated with engraftment. Highlighting the importance of the microenvironment, we will emphasize how external influences can either bolster or impede the successful integration of stem cells. Ultimately, we intend for this examination to not only bridge foundational knowledge with advanced therapeutic applications but also inspire future research directions that promise to refine clinical practices.
Preface to Stem Cell Engraftment
Stem cell engraftment is a vital process in regenerative medicine, where stem cells avidly integrate into host tissues to restore functionality and repair damage. Grasping the intricacies of engraftment is essential not just for laboratories and research institutions but extends its reach to clinical settings where innovative therapies emerge. This section serves as a launch pad into understanding what engraftment entails, its significance, and how it intermingles with both biological principles and therapeutic applications.
Through examining stem cell engraftment, one can appreciate the symbiotic relationship between biological science and practical applications. Indeed, successful engraftment can be the difference between successful recovery or a setback in treatment regimens. Each cell, like a newcomer in an alien town, must establish itself amidst surrounding residents to thrive. This ongoing saga is what practitioners and researchers aim to decode.
Definition and Importance
Engraftment refers to the process during which transplanted stem cells effectively implant into the host tissue and proliferate. This term takes root in the very essence of stem cell research, spotlighting its potential in treating dire conditions like leukemia or chronic degenerative diseases. The importance of understanding this mechanism cannot be overstated; it plays a direct role in optimizing therapies that hinge on stem cells.
Without successful engraftment, even the most promising stem cell therapies can falter. To shed light on this:
- Cell Survival: For a stem cell to engage successfully, it must withstand the host's environmental conditions. These conditions include oxidative stress, nutrient availability, and inflammatory responses.
- Functional Integration: It is not enough for cells to just 'stay put'; they must integrate functionally into the tissue and assume the roles needed—be it producing blood cells or regenerating muscle fibers.
- Therapeutic Implications: As therapies evolve, the aim is often to maximize engraftment efficiency. The outcomes hinge on understanding the localized dynamics, laying bare the relevance of both intrinsic and extrinsic factors.
Recognizing these facets prepares researchers and healthcare practitioners for what lies ahead in stem cell therapy and sets the tone for exploring further.
Historical Context
Tracing the roots of stem cell engraftment provides a fascinating window into medical progress. Stem cells were first identified in the late twentieth century, with significant milestones marking their journey in the medical realm.
- 1970s: The initial flurry stemmed from discoveries regarding bone marrow transplants. This breakthrough opened avenues for treating blood-related disorders.
- 1990s: With advancements in understanding how stem cells functioned, researchers began to grasp the necessity of engraftment for successful outcomes.
- 2000s and Beyond: Fast forward to the 21st century, and the landscape transformed with the advent of induced pluripotent stem cells, showcasing the profound capabilities of stem cells in regenerative medicine.
This ascension through various phases highlights the evolving conversation around stem cell engraftment. Now, with research rooted in diligence and authenticity, strides in this field offer unprecedented hope for understanding, developing, and implementing effective treatment strategies going forward.
"Understanding the past is not merely a retrospective glance; it's a crucial compass guiding the quest to navigate future horizons in science."
Biology of Stem Cells
The study of stem cells is crucial in the broader framework of stem cell engraftment. Understanding their biology provides insights into how these cells can be utilized for therapeutic purposes, particularly in regenerative medicine. Stem cells have unique abilities that set them apart from other cell types—they can self-renew and differentiate into various cell lineages. This not only establishes their foundational role in development and tissue repair but also marks them as prime candidates for medical interventions aimed at regeneration.
Types of Stem Cells
Stem cells are primarily classified into three categories, each with distinct characteristics that dictate their application in therapy and research.
Embryonic Stem Cells
Embryonic stem cells (ESCs) exhibit a remarkable versatility; they are derived from early-stage embryos, granting them pluripotent capabilities. This means they can give rise to any cell type in the body, making them a hot commodity in the field of regenerative medicine. A key characteristic of ESCs is their ability to proliferate indefinitely, which means researchers can generate vast amounts of these cells for study and application.
However, working with ESCs comes with ethical quandaries, particularly around the source of these cells, as they require the destruction of embryos. This presents a significant obstacle in some circles, making researchers tread carefully. Their broad differentiation potential is advantageous, yet the ethical implications can hinder their practical use and acceptance in clinical settings.
Adult Stem Cells
Adult stem cells, in contrast, are found in various tissues throughout the body. These cells are multipotent, which means they have a more limited scope of differentiation compared to ESCs. However, they play an essential role in tissue maintenance and repair. For instance, hematopoietic stem cells, which are found in the bone marrow, are vital for producing blood cells.
A key characteristic of adult stem cells is their relative ease of retrieval from the patient’s own tissues, significantly reducing the risks of immune rejection after transplantation. However, their limited differentiation potential can restrict their application in certain regenerative strategies. While they are practical and ethically sound, their multipotency may not always meet the needs of diverse clinical scenarios.
Induced Pluripotent Stem Cells
Induced pluripotent stem cells (iPSCs) represent a breakthrough in stem cell biology. These cells are generated by reprogramming adult somatic cells to revert them back to a pluripotent state. The ability to create iPSCs from a patient’s own cells opens exciting avenues for personalized medicine. This feature makes iPSCs an appealing option for research and potential therapies since they combine the ethical advantages of adult stem cells with the versatility of embryonic stem cells.
Despite their promise, iPSCs face challenges, particularly around their stability and potential tumorigenicity. The processes used to reprogram these cells can introduce genetic abnormalities, raising safety concerns in therapeutic applications. Nevertheless, their unique feature lies in their ability to mimic the ESCs’ characteristics while avoiding some of the ethical dilemmas tied to embryonic stem cells.
Stem Cell Niches
Stem cell niches are specialized microenvironments that support and regulate stem cells through various biochemical signals and physical interactions. These niches are essential for maintaining the functionality of stem cells, ensuring their proliferation and differentiation when necessary. Factors such as oxygen levels, extracellular matrix components, and neighboring cell types play significant roles in nurturing the stem cells within these niches. Understanding these niches is pivotal for developing therapeutic strategies to enhance engraftment and tissue regeneration, as it gives insights into how to simulate these environments in vitro and facilitate better outcomes post-engraftment.
The Engraftment Process
The process of engraftment is a pivotal element in the realm of stem cell therapies, acting as a bridge that connects the initial therapeutic intervention with the desired clinical outcomes. It is during this phase that stem cells migrate from their injection site to their target tissues, eventually integrating into those environments to regenerate or repair damaged cellular structures. Understanding the intricacies of engraftment can significantly enhance therapeutic efficacy and improve patient outcomes.
As we delve into this fascinating topic, it’s essential to recognize the three fundamental stages of engraftment: homing, migration, and integration. Each stage plays a critical role by ensuring that the stem cells successfully navigate their journey within the body, establishing a foothold in areas where they can exert their regenerative effects. By dissecting these stages, we not only illuminate the mechanics of engraftment but also identify opportunities for improvement in stem cell therapies.
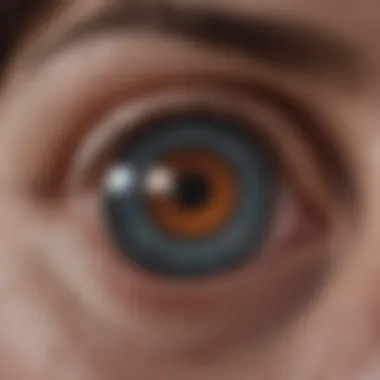
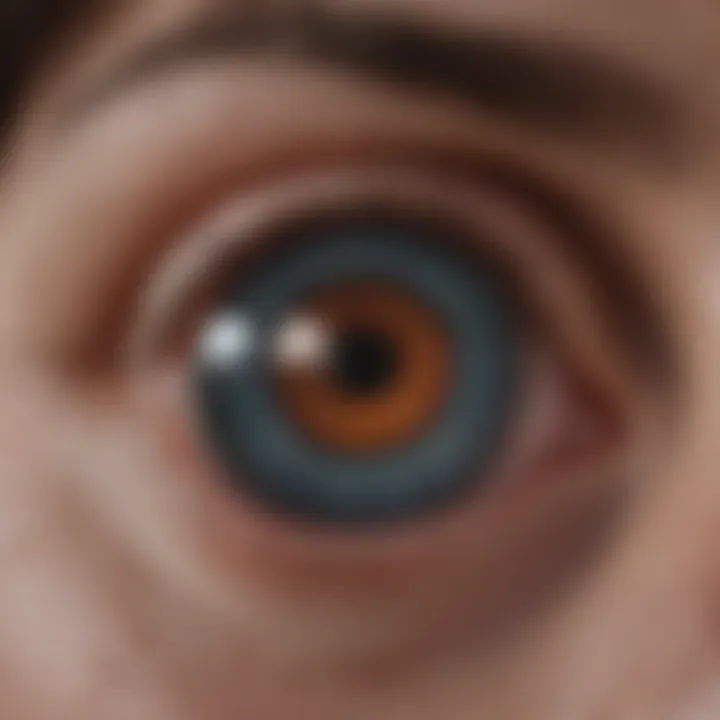
Stages of Engraftment
Homing
Homing represents the initial phase where stem cells find their way from the bloodstream to the target tissue. This process is crucial; it ensures that the stem cells arrive at the appropriate destination. The primary characteristic of homing is guided by cellular signaling. Stem cells utilize specific receptors to respond to chemokines and cytokines present in the tissues. This directional movement is often likened to a homing pigeon returning to its nest, providing a clear analogy for the journey stem cells undertake.
One unique feature of homing lies in its reliance on the microenvironment. For instance, when stem cells are introduced into the body, they actively detect chemical signals in the host tissues. Their success relies heavily on these cues. However, external factors such as inflammation or the presence of competing cell populations can complicate homing, leading to variable outcomes. This phase is not without its challenges, but understanding it can lead to strategies that enhance the precision of where stem cells are deposited within the target tissues.
Migration
Once stem cells have successfully homed to their destination, the next step is migration. This step is characterized by the movement of these cells through the extracellular matrix—essentially the scaffolding of tissue. It involves complex interactions between the stem cells and the surrounding matrix components.
A key point about migration is that it requires significant energy and cellular activity, akin to climbing a steep hill. The stem cells need to retract and extend their cellular processes, navigating through various obstacles along the way. The complexity of this journey underscores the importance of understanding matrix composition and its influence on cellular movement. Rough or rigid areas can hinder migration, making it vital to prepare a conducive environment for the stem cells.
However, while migration is critical, it comes with its own set of complications. For example, cells that do not effectively migrate can become stagnant or even apoptotic if they fail to reach their target site. Therefore, comprehending the mechanisms that drive migration is essential not only for ensuring efficiency but also for maximizing the survival prospects of transplanted stem cells.
Integration
Finally, integration signifies the moment when stem cells embed themselves within the target tissue, effectively becoming part of the existing cellular architecture. This stage cement the stem cells' new role in tissue restoration or regeneration.
Integration hinges on the cells' ability to establish functional connections with host cells. A notable characteristic of integration is its variability, influenced by both the origin of the stem cells and the tissue type involved. For instance, hematopoietic stem cells, when integrated into bone marrow, undergo specific adaptations not seen in other forms of stem cell engraftment. This aspect highlights the necessity for precision in selecting appropriate stem cell types for various medical applications.
With these features comes a duality of advantages and disadvantages. On one side, successful integration can restore function to damaged tissues, while on the other, unsuccessful integration may lead to poor outcomes or undesirable responses such as fibrosis or aberrant growth.
Understanding each of these stages offers insights into improving engraftment efficiency. By developing techniques aimed at facilitating homing, migration, and integration, researchers aim to advance the therapeutic potential of stem cells in regenerative medicine.
Cell-Cell Interactions
Cell-cell interactions play an equally significant role during the engraftment process, as they influence stem cells' performance in vivo. These interactions not only impact engraftment success but also dictate how integrated stem cells will function within the host tissues. Researchers are increasingly recognizing that fostering positive relationships between stem cells and neighboring cells can enhance therapeutic outcomes, making it an essential area of focus in ongoing studies.
Factors Influencing Engraftment Success
The success of stem cell engraftment is not just a simple toss of the dice; it's influenced by a blend of factors that can either pave the way for successful integration or throw a wrench in the works. Understanding these factors is crucial as it digs into the essence of how and why certain engraftments succeed while others lag behind. This section tackles the intricate relationship between intrinsic and extrinsic elements that sway engraftment outcomes. By examining these dynamics, researchers and practitioners can glean insights that could lead to enhanced therapies in the realm of regenerative medicine.
Intrinsic Factors
Intrinsically, stem cells come with their own set of characteristics that significantly affect their engraftment performance. Diving deeper, we look at two major aspects that shape their intrinsic value, namely Stem Cell Origin and Cell Cycle Dynamics.
Stem Cell Origin
Stem cell origin plays a pivotal role in engraftment success. Different types of stem cells exhibit various traits based on their origin, leading to diverse engraftment potentials. For instance, stem cells derived from embryos typically have a broader range of differentiation capabilities compared to adult stem cells, which may possess a more limited plasticity. This characteristic of embryonic stem cells stems from their pluripotent nature, allowing them to transform into multiple cell types.
On the flip side, adult stem cells, while more specialized, come with their own set of advantages. They often have a lower risk of teratoma formation, making them a safer option for certain applications. Employed in treatments such as hematopoietic stem cell transplantation, their well-documented abilities lend credence to their use in clinical settings.
In short, the unique features of stem cell origin can either enhance or hinder engraftment, leading to significant differences in therapy outcomes. Careful consideration of these attributes is necessary for optimal engraftment strategies.
Cell Cycle Dynamics
Another intrinsic factor of great importance is cell cycle dynamics. The timing of a cell’s entry into the active cycle influences how well it engages in the engraftment process. Stem cells that are in a more proliferative state tend to possess a better capacity for migration and integration into host tissues. For example, cells in the S phase of the cell cycle may exhibit heightened activity, thereby influencing their survival and functional integration.
While this aspect can certainly boost engraftment potential, it adds layers of complexity. Cells in a more quiescent state may exhibit slower integration but provide stability and longevity in the niche they settle into. Consequently, understanding the nuances of cell cycle dynamics helps tailor strategies that either accelerate engraftment or improve long-term survival. Balancing these dynamics is the key to customs that would suit specific therapeutic goals.
Extrinsic Factors
Beyond intrinsic characteristics, various extrinsic factors also hold sway over the success of engraftment processes. Microenvironment conditions and biochemical signaling all contribute to the nuances of how well stem cells thrive after being introduced into a target area.
Microenvironment Conditions
Microenvironment conditions act as a guiding compass for stem cell behavior after engraftment. This includes factors like oxygen levels, pH, and the presence of various extracellular matrix components. For instance, a hypoxic environment can trigger specific cellular pathways that enhance stem cell survival and integration, which is a vital consideration for engraftment success.
What makes microenvironmental conditions especially fascinating is their ability to vary significantly between different tissues. This heterogeneity means that one size certainly does not fit all—strategies that work for engraftment in one type of tissue may not translate well into another.
In summary, attention to the specific microenvironment conditions can create a nurturing setting for engraftment, thereby bolstering cellular functions and acceptance.
Biochemical Signaling
Biochemical signaling serves as the final piece of the puzzle in understanding extrinsic factors. Different signals orchestrated by the host tissue can either enhance or inhibit stem cell engraftment. These signals include various cytokines, growth factors, and other molecules that can stimulate or suppress stem cell activity.
Let’s consider the example of growth factors like vascular endothelial growth factor (VEGF), which plays a critical role in promoting angiogenesis. This signaling can accelerate the formation of new blood vessels, crucial for providing stem cells with nutrients and oxygen following their engraftment.
The challenge lies in the ability of transplanted stem cells to respond accurately to these biochemical signals. If they can’t interpret the cues of the surrounding environment appropriately, it can lead to failure of engraftment. Hence, it’s crucial to study the complex interplay of biochemical signaling to optimize engraftment strategies effectively.
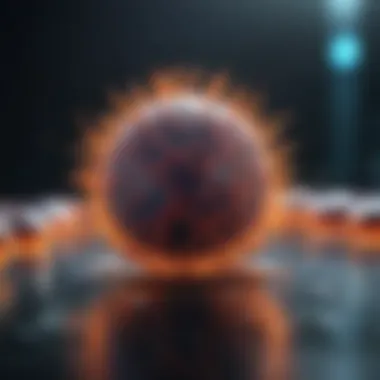
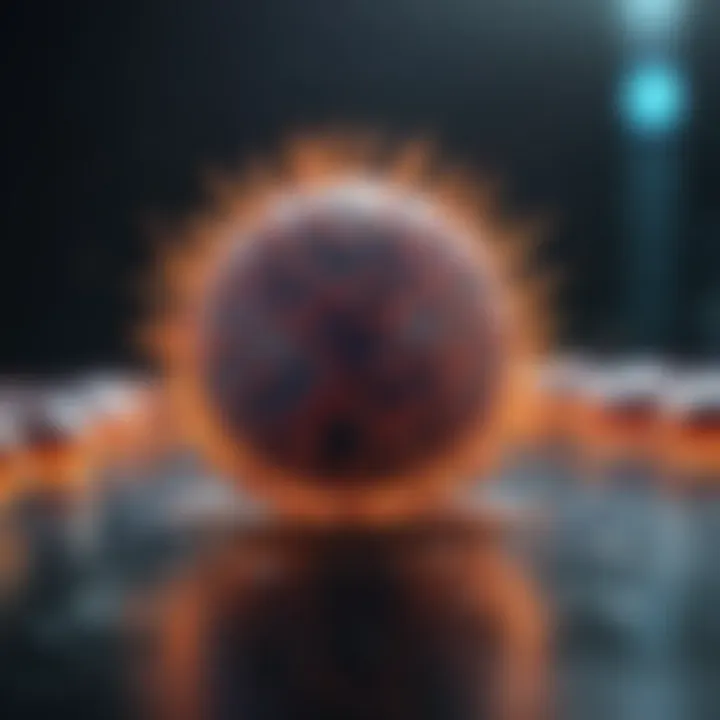
Understanding the layers of intrinsic and extrinsic factors behind engraftment can be the difference between trial and triumph in regenerative therapies.
In summary, the intertwining of these intrinsic and extrinsic factors greatly influences the success of stem cell engraftment. Recognizing their roles can assist in carving out strategies that advance our understanding and capability within regenerative medicine.
Challenges in Stem Cell Engraftment
Understanding the challenges associated with stem cell engraftment is crucial for improving the efficacy and safety of stem cell therapies. Successful engraftment is the cornerstone of regenerative medicine, influencing the overall outcome of treatments. Various hurdles, ranging from biological incompatibilities to the unpredictable nature of stem cell behavior, can significantly dampen the success rates of such interventions.
The two most prominent challenges that researchers face are immune rejection and limited cell survival. Addressing these issues not only enhances the performance of stem cell therapies but also contributes to a greater understanding of the intricate interplay between stem cells and their environment. Here’s a closer look at these pressing challenges.
Immune Rejection
One of the foremost obstacles in engraftment is immune rejection. This phenomenon occurs when the recipient's immune system identifies transplanted stem cells as foreign entities and mounts an attack against them. The body’s natural defense mechanism can view even autologous stem cells—those derived from the same individual—as threats, particularly if they have been manipulated or cultured outside of their original environment for an extended period.
- Factors at Play: Various elements influence immune rejection, including the genetic compatibility between the donor and recipient. Human Leukocyte Antigen (HLA) matching plays a pivotal role here. Mismatched HLA molecules can lead to acute graft-versus-host disease (GVHD), which not only threatens the engrafted cells but can also cause severe complications for the patient.
- Strategies for Mitigation: Several strategies are being explored to mitigate immune rejection. These include:
- Immunosuppressive Therapy: This approach involves administering drugs designed to dampen the body's immune response, making it easier for transplanted cells to survive. However, the downside is the increased risk of infections and other complications.
- Stem Cell Engineering: Genetic modification of stem cells to enhance their survival rate and reduce their recognition as foreign cells is another area of interest. Techniques like gene editing have shown promise in this regard.
Immune rejection remains a critical barrier that limits the success of engraftment in clinical settings, and understanding its mechanisms will pave the way for more refined treatment strategies.
Limited Cell Survival
The second major challenge is the limited cell survival following engraftment. Upon transplantation, stem cells must navigate a hostile environment that can hinder their ability to thrive and integrate into the host tissue. Various factors contribute to cell death, including:
- Microenvironmental Conditions: The niche into which the stem cells are transplanted significantly influences their survival odds. Harsh conditions such as nutrient deprivation, low oxygen levels, or acidic pH can inhibit stem cell function and lead to premature cell death.
- Intrinsic Cell Factors: The characteristics of the stem cells themselves, such as their age, genetic stability, and metabolic state, also play crucial roles. For example, aged stem cells may lose their reparative capabilities, rendering them less effective upon transplantation.
To improve cell survival, researchers are employing various techniques:
- Pre-conditioning Treatments: These involve preparing the stem cells or the recipient tissue to create a more hospitable environment prior to transplantation. For example, inducing mild hypoxia before engraftment can enhance cell resilience against the harsher conditions they may encounter.
- Supportive Therapies: Utilizing factors that promote cell survival, such as specific growth factors or cytokines, can aid the engraftment process by offering a supportive environment for the cells to flourish.
These challenges intersect and complicate the engraftment process, marking them as significant considerations in the development of more efficient and reliable stem cell therapies.
"Understanding and overcoming these challenges is not just about improving transplant success; it is about providing hope and better outcomes for patients facing chronic conditions."
The continued research into these obstacles is not merely an academic endeavor. It has profound implications for future clinical applications of stem cell therapy. As we dissect these barriers, the insights gained will drive innovation, ultimately leading to refined techniques and improved patient outcomes.
Techniques to Enhance Engraftment
The success of stem cell engraftment is a decisive factor in the efficacy of regenerative therapies. Techniques that enhance engraftment are pivotal not just in increasing the survival rate of transplanted cells but also in their functionality within the host. Thiss section will explore two prevalent strategies: preconditioning and genetic modification, shedding light on their significance and potential applications.
Preconditioning Strategies
Preconditioning strategies represent a crucial component in preparing the patient or tissue environment for the introduction of stem cells. This step essentially creates a hospitable setting, which is necessary because the microenvironment can significantly influence the behavior and fate of incoming cells.
Preconditioning can involve various approaches:
- Chemotherapeutic agents: Administering certain drugs can help create space within the bone marrow or affected tissues. For instance, agents like cyclophosphamide can deplete existing, unwanted cells, thereby giving incoming stem cells a better chance to thrive.
- Irradiation: This method reduces the immunogenicity of the target tissue, making it less hostile to the transplanted cells. However, the risks involved with irradiation need careful consideration, as they can lead to unwanted damage to surrounding tissues.
- Cytokine treatment: Using specific growth factors can boost the body's own stem cell population or improve the regenerative environment. The infusion of granulocyte-colony stimulating factor (G-CSF) is one example that helps in mobilizing stem cells from their niches into peripheral circulation, where they can be harvested more effectively.
Preconditioning is like setting the stage for a grand performance; the better the stage is prepared, the more likely the show will go on without a hitch.
These strategies often require a fine balance. While being effective in enhancing engraftment, they also carry risks, and improper application might result in complications or even adverse effects in patients. Therefore, a deep understanding of these strategies, along with monitoring advancements in research, is essential for optimizing outcomes.
Genetic Modification
Genetic modification is another intriguing and rapidly evolving area that has the potential to significantly improve the efficiency of stem cell engraftment. Through various techniques, stem cells can be altered at the genetic level to enhance their properties, making them more suitable for therapies.
There are some common approaches to genetic modifications:
- CRISPR-Cas9 technology: This revolutionary method allows for precise edits to DNA, enabling the insertion or deletion of specific genes. For example, researchers might edit stem cells to knock out genes that are known to trigger rejection or to enhance their migration ability toward damaged tissues.
- Viral vectors: These are often used to deliver beneficial genes into the stem cells. For example, introducing growth factors that promote survival and integration can increase the chances of successful engraftment. However, the challenge with this method lies in ensuring that the viral vector does not cause unwanted side effects or mutations.
- Transgenic approaches: These involve integrating new genes into the stem cell's genome to give them traits like resistance to apoptosis or enhanced proliferation capabilities.
Genetic modification enhances not only the engraftment potential but also the functional capabilities of the stem cells post-engraftment. However, ethical considerations surrounding the use of such techniques should not be overlooked, as they raise questions about the long-term consequences and implications for patients.
Clinical Applications of Stem Cell Engraftment
The sphere of stem cell engraftment bears significance that reaches beyond the confines of laboratory research; its applications in clinical settings are monumental. The ability to harness stem cells for therapeutic purposes has the potential to alter the landscape of modern medicine. This section delves into the pivotal role of stem cell engraftment in clinical practice, illustrating its broad implications on patient care and treatment efficacy.
Hematopoietic Stem Cell Transplantation
Hematopoietic stem cell transplantation (HSCT) stands as a cornerstone in treating various hematological malignancies like leukemia and lymphoma. The essence of HSCT lies in its capacity to repopulate the bone marrow with healthy blood cells after the destructive effects of chemotherapy or radiation.
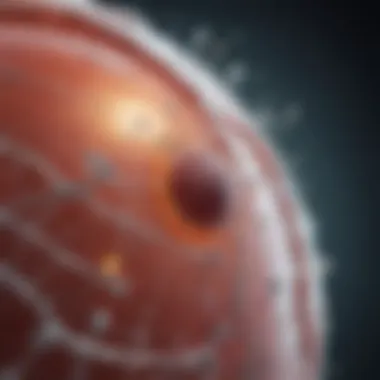
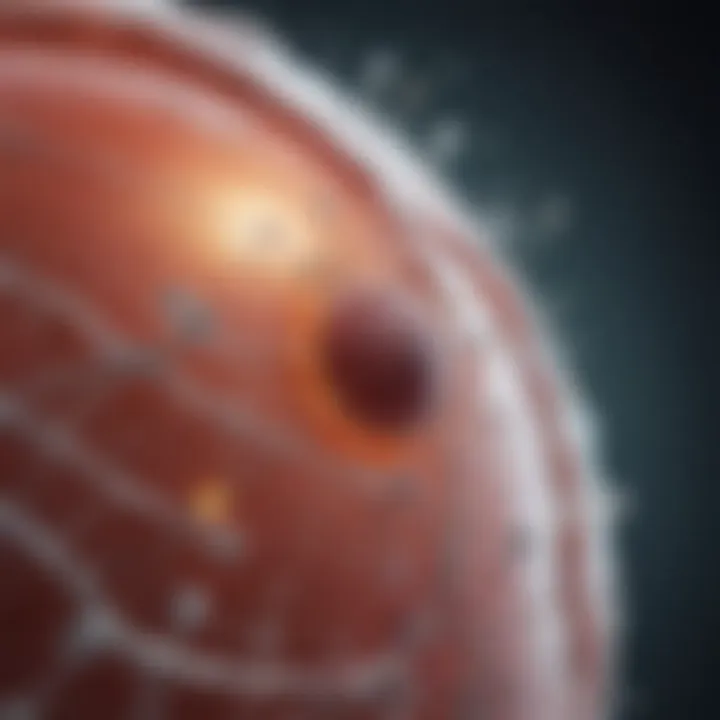
- Process Overview: The procedure begins with the collection of stem cells from either the patient or a matched donor. Following this harvest, the patient undergoes conditioning therapy, aiming to eradicate diseased cells and make space for the new cells to thrive. Finally, the harvested stem cells are infused into the patient, embarking on their journey to engraft and restore normal blood production.
- Advantages: The benefits of HSCT are manifold. Beyond simply replenishing blood cells, this technique enhances immune system function, offering patients a renewed vigor to fight infections. Many patients experience prolonged remissions thereafter, underscoring the importance of successful engraftment.
However, HSCT also faces considerable hurdles. Immune reactions post-transplant can lead to complications such as graft-versus-host disease (GVHD). Thus, careful matching of donor and recipient is crucial.
"Hematopoietic stem cell transplantation not only aims for survival but attempts to yield a quality of life that many patients thought lost."
Regenerative Medicine Approaches
Beyond hematological disorders, stem cell engraftment shows promise in regenerative medicine. This approach prioritizes the repair or replacement of damaged tissues and organs through stem cell intervention. One of the most compelling applications involves treating neurodegenerative diseases like Parkinson’s or Alzheimer’s by implanting neural stem cells that may restore lost functions.
- Examples of Application:
- Considerations: While the promise is exciting, the effectiveness and safety of these therapies require more extensive investigation. Challenges abound, including tracking the long-term engraftment of cells, potential tumorigenesis, and ensuring proper differentiation of stem cells into the desired tissue types.
- Cardiac Regeneration: Stem cells can potentially mend damaged heart tissue, improving heart function following a myocardial infarction.
- Bone and Cartilage Repair: Mesenchymal stem cells are being researched for their ability to aid in the healing of bone fractures and cartilage injuries, thus promoting overall joint health.
In essence, the applications of stem cell engraftment in clinical practice encapsulate a blend of hope and complexity. Each application, whether it is HSCT or regenerative methodology, underscores the need for meticulous research and thoughtful innovation to unlock the full potential of stem cell therapies in improving patient outcomes.
Ethical Considerations
The field of stem cell engraftment is not only nuanced from a biological perspective but also deeply intertwined with ethical considerations. As innovations push the boundaries of what we can achieve with stem cells, it’s crucial to reflect on the moral implications of these advancements.
Source of Stem Cells
The origin of stem cells is one of the most debated ethical issues in this domain. Stem cells can be harvested from various sources, each carrying its own set of ethical dilemmas.
- Embryonic Stem Cells: These are derived from early-stage embryos, which leads to considerable controversy. Many individuals and religious groups believe that life begins at conception, adding a layer of moral complexity. The debate often centers on whether it's justifiable to use embryos for research when potential life is at stake.
- Adult Stem Cells: Obtained from tissues such as bone marrow or fat, adult stem cells are viewed as less contentious. However, they may offer limited differentiation potential compared to embryonic stem cells, leading researchers to argue about their efficacy in certain therapies.
- Induced Pluripotent Stem Cells (iPSCs): These cells are reprogrammed from adult cells to an embryonic-like state. While they avoid the ethical concerns of using embryos directly, questions still arise about consent and the manipulation of genetic materials.
Ultimately, the need for ethical oversight is paramount. Researchers must navigate these waters carefully to ensure that they respect both human life and the pursuit of scientific knowledge. Decisions made about the source of stem cells can influence public perception and funding in stem cell research.
"The essence of scientific progress often relies on the delicate balance between innovation and ethics."
Regulatory Framework
The regulatory landscape surrounding stem cell research is complex and varies significantly worldwide. Different countries have developed different standards, shining a light on the need for coherent and comprehensive regulations.
- Government Regulations: In many jurisdictions, government bodies set stringent rules about the use of stem cells, especially embryonic types. Agencies like the Food and Drug Administration (FDA) in the United States require rigorous testing and clinical trial phases before any stem cell based treatment can be approved.
- Ethics Committees: Institutions often establish internal review boards to assess research proposals involving stem cells. These committees look into the ethical implications of research objectives, ensuring that protocols are established to protect the rights of participants.
- International Standards: Collaborative efforts, such as the guidelines from the International Society for Stem Cell Research (ISSCR), aim to provide a global framework. These guidelines include principles like respect for persons, beneficence, and justice, which guide researchers and promote ethical practices across borders.
The evolving nature of stem cell research necessitates ongoing dialogue about these ethical considerations and regulatory measures. As the realms of science and medicine intertwine ever more closely, establishing thorough, thought-out guidelines is crucial to uphold ethical integrity — ensuring that stem cell advancements yield beneficial outcomes without compromising moral values.
Future Directions in Stem Cell Engraftment Research
In recent years, stem cell engraftment has emerged as a pivotal aspect of regenerative medicine. The future is bright as ongoing investigations pave the way for innovative strategies aimed at enhancing engraftment success. Understanding where this field is headed is crucial, not just to keep pace with advancements, but to realize the full potential of stem cell therapies in clinical settings. With science constantly advancing, researchers are looking at various angles—technology, biology, and patient-centric approaches—all aiming to improve outcomes.
Innovations in Cell Therapies
The development of novel cell therapies stands to reshape the landscape of stem cell engraftment. Recent technological leaps have brought forth exciting innovations. One notable example includes the advent of bioengineered scaffolds, which effectively mimic the native extracellular matrix. These supports guide stem cells during the engraftment process, improving their integration and survival in the host tissue.
Moreover, researchers are exploring the realm of 3D bioprinting. This innovative method allows for the precise placement of stem cells in specific patterns, optimizing their ability to engraft and function within the targeted tissue. This synergistic combination of technology and biology enhances the overall practicality and scope of stem cell therapies.
As techniques evolve, genetic editing tools like CRISPR stand out. They enable scientists to refine stem cell characteristics, making them more resilient to challenging microenvironments or even resistant to immune rejection. This capacity to tailor stem cells to individual patient needs represents a significant leap forward in patient outcomes and precision medicine.
"The impact of these innovations extends beyond mere survival rates; we are now looking at the possibility of creating fully functional tissues tailored specifically for individual patients."
Potential for Personalized Medicine
Personalized medicine is set to play a game-changing role in stem cell engraftment. As the field progresses, there's a growing understanding of how genetic profiles and environmental factors uniquely influence each patient's response to therapies. This knowledge fosters the development of highly individualized treatment plans.
With advancements in genomics and biotechnology, clinicians can now select the most suitable types and sources of stem cells, taking into account a patient’s unique genetic makeup, background, and even their specific disease state. For instance, rather than using a one-size-fits-all approach, medical professionals might employ a patient’s own stem cells or those derived from closely matched donors to reduce the risk of rejection and improve engraftment efficiency.
Additionally, integrating advanced diagnostics allows for real-time monitoring of engraftment status. This capability enables adjustments to be made on-the-fly, ensuring therapies remain aligned with the patient’s evolving biological landscape. As such, the potential for personalized medicine not only enhances the efficacy of treatments but also tailors the entire healing experience.
In summary, the future directions in stem cell engraftment research promise significant strides through innovations in cell therapies and personalized medicine. These developments open doors for enhanced effectiveness and patient-centric approaches that may ultimately redefine the field, making treatment safer and more effective.
Closure
The conclusion serves as the culmination of insights gathered throughout this article, wrapping up the intricate tapestry of stem cell engraftment. Understanding engraftment is not merely an academic exercise; it's a pivotal facet of regenerative medicine that can significantly enhance patient outcomes. This concluding section underscores the importance of integrating various elements discussed, from biological mechanisms to ethical considerations.
Summary of Findings
The article highlights several key findings regarding stem cell engraftment, including:
- Mechanisms of Engraftment: Successful engraftment hinges on a series of coordinated biological processes. The homing of stem cells to target tissues, their migration through specialized niches, and subsequent integration into existing cellular frameworks are crucial steps.
- Influencing Factors: Both intrinsic and extrinsic factors play critical roles in the success of engraftment. For instance, stem cell origin and the surrounding microenvironment can dictate the overall efficacy of engraftment procedures.
- Challenges: Immune rejection and limited cell survival present significant hurdles. These challenges necessitate innovative strategies to enhance engraftment success rates.
- Future Directions: Innovations, such as personalized medicine approaches, hold promise for more effective stem cell therapies. Understanding the microenvironment's role could further refine these strategies.
These findings reflect the complexity of stem cell engraftment, showcasing it as an area ripe for exploration and development.
Final Thoughts on Engraftment
In summary, the significance of stem cell engraftment cannot be overstated. The implications for regenerative medicine are profound, potentially altering the landscape of treatment for diseases that, until now, were deemed challenging or insurmountable. The adoption of refined techniques and an emphasis on the microenvironmental dynamics of engraftment offer pathways toward more successful outcomes.