Understanding the RNA PCR Test: Methodology and Implications
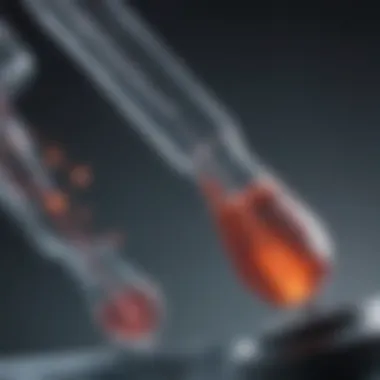
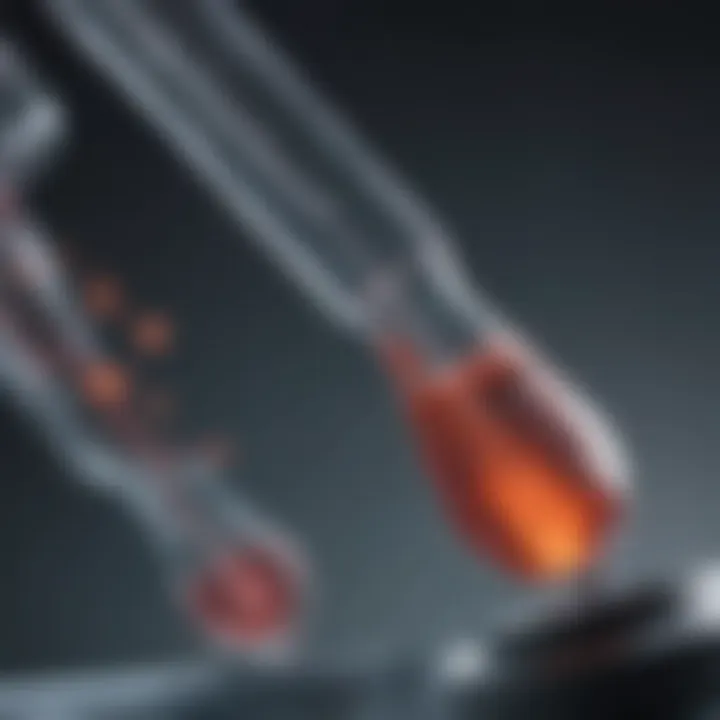
Intro
The world of molecular biology has evolved by leaps and bounds, fundamentally changing how we diagnose and treat diseases. One such monumental shift is represented by the RNA PCR test. Understanding this test isn’t just for the seasoned experts; it’s equally relevant for students and professionals who wish to stay ahead in the rapidly progressing field of medical science. This article aims to break down the intricacies of the RNA PCR test. By diving into its methodologies, challenges, and the future it holds in clinical and research settings, readers will find themselves better equipped with knowledge vital for making informed decisions in their respective areas.
Methodology
The realm of RNA PCR testing is complex and layered, but its methodology can be demystified through structured examination.
Study Design
At the heart of the RNA PCR test lies a meticulous study design that outlines its structure and approach. Typically, the studies employing RNA PCR involve controlled environments where biological samples, such as blood or tissue, are collected from subjects displaying symptoms of viral infections. This comparability in study design is crucial for ensuring reliable outcomes.
- Sample Size: The number of samples can vary widely, often depending on the infectious disease in focus. For statistical significance, studies usually aim for a larger sample size, which can range from dozens to thousands, depending on the research scale.
- Control Groups: Including a control group free from the infection ensures that results are valid. Comparing infected versus non-infected samples allows for clearer interpretations of the data collected.
Data Collection Techniques
Data collection in the RNA PCR test is rigorous and finely calibrated. Techniques employed span across various stages of the testing process.
- RNA Extraction: This is the initial phase, where the RNA is isolated from the sample using chemicals that effectively break down cell membranes.
- Reverse Transcription: The extracted RNA is then converted into complementary DNA (cDNA) by reverse transcriptase enzymes, preparing it for amplification.
- PCR Amplification: This step catapults the test into the spotlight. Using thermal cycles, targeted regions of the cDNA are exponentially copied, allowing for detection of even the smallest traces of viral genetic material.
- Results Interpreting: The final phase involves analyzing the amplified products through gel electrophoresis or real-time PCR instrumentation, depending on the methodology used.
"The RNA PCR test's robust design and data collection techniques lay the foundation for its critical role in diagnostics, propelling scientific discovery forward."
Discussion
Navigating through the results derived from RNA PCR testing invites contemplation on various angles that merit discussion.
Interpretation of Results
The interpretation of results from RNA PCR tests is not merely about obtaining positive or negative outcomes. Nuances abound in differentiating between viral presence and the level of viral load. For instance, a high viral load might suggest an acute infection, while low levels could indicate a recent infection or recovery phase.
Limitations of the Study
Despite its promises, RNA PCR testing isn't without its flaws.
- Sensitivity and Specificity: False positives and negatives can occur, influenced by sample quality or timing of infections.
- Technical Complexity: The intricate processes of RNA extraction and amplification may introduce variability, making standardization across laboratories challenging.
Future Research Directions
As we look ahead, several avenues present themselves for future research.
- Automation: Enhancements in automation could streamline processes, increasing the reliability and efficiency of RNA PCR testing.
- Point-of-Care Testing: Research focusing on point-of-care tests could democratize access to this important diagnostic tool, enabling quicker decision-making in clinical settings.
Understanding the RNA PCR test isn't just an academic exercise; it's crucial for advancing both diagnostics and therapeutic strategies in medicine today. Every aspect, from its methodology to future implications, sheds light on its significant role in combating infectious diseases.
Intro to RNA PCR Testing
RNA PCR testing has emerged as a cornerstone in the realm of molecular diagnostics. Its significance, especially in the context of contemporary healthcare, cannot be overstated. By facilitating the rapid and precise detection of RNA viruses, this method not only aids in diagnosing infectious diseases but also enhances our understanding of complex biological processes at a molecular level. In an age where viruses—like SARS-CoV-2—can have vast implications for public health, grasping the nuances of RNA PCR testing is crucial.
Definition of RNA PCR Test
The RNA PCR test, or Polymerase Chain Reaction test for RNA, is a sophisticated technique that amplifies specific RNA sequences, making them detectable even in scant amounts found in biological samples. This process involves converting RNA into complementary DNA (cDNA) using an enzyme called reverse transcriptase, followed by amplification of that cDNA through standard PCR methods. This dual-step process allows for accurate quantification and enables the detection of low-abundance RNA in a range of samples, from clinical specimens to environmental sources.
History and Development
The journey of RNA PCR testing began in the late 1980s, following the advent of traditional PCR technology by Kary Mullis. Initially designed for DNA, it wasn't long before the potential for RNA detection became evident. In 1990, the development of reverse transcription PCR (RT-PCR) opened new avenues for diagnosing viral infections, paving the way for the explosive growth of molecular diagnostics. Since then, advancements in enzyme technology, thermal cycling apparatus, and analytic software have enhanced the sensitivity and specificity of RNA PCR tests.
Today, techniques like quantitative PCR (qPCR) further refine how scientists measure gene expression levels, allowing researchers and clinicians to interpret the data with unprecedented accuracy. These developments have made RNA PCR indispensable within both laboratory settings and real-world applications— from detecting pathogens to understanding genetic diseases.
"The emergence of RNA PCR testing has radically transformed our approach to disease diagnosis and monitoring."
As this method continues to evolve, its importance in personalized medicine and research applications grows accordingly. Engaging with RNA PCR testing not only equips professionals in the healthcare sector with invaluable tools but also offers a deeper insight into the fundamental mechanisms of life itself.
Basic Principles of PCR Techniques
Understanding the basic principles of Polymerase Chain Reaction (PCR) is essential for grasping how RNA PCR operates in molecular biology. This segment lays down the crucial concepts that underpin RNA PCR testing, underscoring its significance in various applications such as clinical diagnostics and research.
Polymerase Chain Reaction Explained
At its core, PCR is a technique that allows for the amplification of specific DNA or RNA sequences, turning a minute quantity of genetic material into millions of copies. Think of it as a multiplier for genetic information. The power of PCR lies in a few key processes.
The technique begins with the denaturation of the target nucleic acid, usually by heating the mixture to about 95°C. This step essentially "unzip" the double-stranded molecule, creating two single strands for copying.
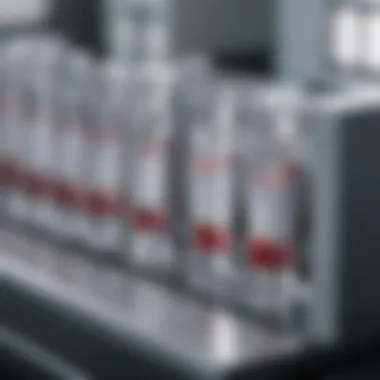
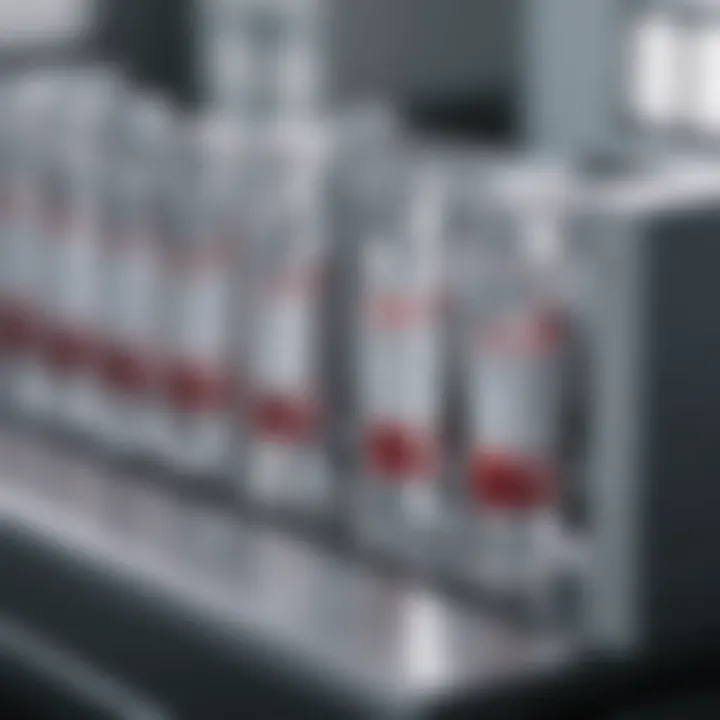
Next comes annealing, where the temperature is dropped to around 50-65°C to allow short sequences called primers to bind to their complementary sequences on the single-stranded templates. Without these primers, the PCR process would stall, as DNA polymerase would have no spot to start.
Finally, the extension phase kicks in, where the temperature is raised again, allowing the DNA polymerase to synthesize new DNA strands by adding nucleotides to the primers. The entire cycle is repeated multiple times, usually 20-40 cycles, exponentially increasing the amount of target nucleic acid.
Role of Primers and Enzymes
Primers and enzymes are the unsung heroes in any PCR process. Primers, specifically designed sequences that bind to target RNA or DNA, are crucial because they dictate the specificity of the amplification. A well-chosen primer can mean the difference between a successful PCR and one that fails completely.
Enzymes also pivotally influence the process. Taq polymerase, the most widely used enzyme in PCR, is heat-stable, which makes it perfect for withstanding the high temperatures of the denaturation phase. Other enzymes like Reverse Transcriptase, which is used in RNA PCR, convert RNA into complementary DNA (cDNA), setting the stage for the traditional PCR cycle. Together, these components work in harmony to ensure that only the desired target is amplified, minimizing contamination and error in results.
Thermal Cycling Steps
The thermal cycling steps in PCR serve as the backbone of the entire process. Each step plays a specific role in ensuring successful amplification. The cycling typically includes three main steps:
- Denaturation: As previously mentioned, this step involves heating the mixture to separate the strands of DNA or RNA.
- Annealing: When the temperature drops, primers find their perfect match on the separated strands and bind.
- Extension: Here, the DNA polymerase connects nucleotides, effectively constructing new strands, thus amplifying the initial target.
Moreover, more sophisticated protocols may introduce additional steps or vary temperatures and durations, particularly in experiments requiring higher specificity or sensitivity.
"PCR is not just about amplification; it’s about precision, specificity, and speed, hinging on careful control of temperature and time."
In summary, the basic principles underpinning PCR techniques are foundational to the effectiveness and accuracy of RNA PCR testing. An in-depth understanding of these mechanics not only aids in the technical execution of tests but also enhances interpretation and application in both clinical and research settings.
By resolving the minutiae of this powerful technique, professionals and students alike can appreciate its role in advancing medicine and biotechnology.
Applications of RNA PCR Testing
The applications of RNA PCR testing extend far beyond the confines of a laboratory, permeating various fields to bring about significant advances in healthcare, research, and environmental science. The essence of this test lies in its ability to detect and quantify RNA from different sources, providing insights that have far-reaching implications. Let’s delve into the specific realms where RNA PCR testing has proven indispensable.
Clinical Diagnostics
In the realm of clinical diagnostics, RNA PCR testing serves as a cornerstone for accurately identifying pathogens, particularly viruses. For instance, during the COVID-19 pandemic, the ability to swiftly detect SARS-CoV-2 RNA became crucial for both individual patient care and public health strategies. This methodology allows healthcare professionals to conduct diagnostic testing with notable sensitivity and specificity, often resulting in faster treatment decisions and improved patient outcomes.
Additionally, RNA PCR can assist in the diagnosis of a variety of other conditions, such as respiratory infections, HIV, and other viral, bacterial, and fungal diseases.
- Rapid Turnaround: The speed at which results are delivered is a game changer in clinical settings.
- Target Specificity: The test's ability to amplify specific RNA sequences ensures that the diagnosis is not only accurate but also reliable.
"In a world where time is of the essence, RNA PCR testing trims the fat from the typical diagnostic process, revealing the exact culprits without much ado."
Research Applications
Researchers are increasingly leveraging RNA PCR testing for a plethora of studies. In fundamental research, understanding gene expression through RNA quantification can unveil the mysteries of cellular mechanisms and disease processes. This approach can aid in developing targeted therapies and emerging treatments by illuminating relationships between RNA levels and specific health conditions.
Moreover, RNA PCR testing facilitates experimentation in genetic studies, including RNA interference and gene editing ventures. Its precise nature can highlight even the slightest fluctuations in RNA quantities, enabling a better understanding of how various external factors — such as environmental stressors or pharmaceuticals — impact gene expression.
- Gene Expression Profiling: Essential for identifying diseases at the molecular level.
- Epidemiological Studies: Understanding the spread of infectious diseases becomes easier when specific RNA sequences are targeted.
Environmental Monitoring
The significance of RNA PCR testing in environmental monitoring cannot be understated. Its capability to detect and quantify RNA from microorganisms helps in assessing ecosystem health and identifying potentially hazardous organisms in various environments, such as soil, water, and air. Environmental scientists utilize this testing method for several purposes, such as tracking pollution, monitoring biodiversity, and managing natural resources.
For example, detecting the RNA of dangerous pathogens in water systems aids public health officials in prompt responses to contamination events, safeguarding communities from waterborne diseases. With a focus on sustainability, RNA PCR can also contribute to monitoring and assessing biodiversity by characterizing microbial communities in different habitats.
- Biodiversity Assessment: Gives a clearer picture of ecosystem health.
- Disease Outbreaks: Early detection of harmful algal blooms or pathogens ensures timely interventions.
In essence, the applications of RNA PCR testing are diverse and impactful, cutting across various spheres of human endeavor. This technology does not just belong in a research facility; it’s an integral part of modern clinical diagnostics, research initiatives, and environmental stewardship.
Technical Aspects of RNA PCR
The technical facets of RNA PCR are pivotal, forming the backbone of its reliability and efficiency. Mastering these elements not only enhances the accuracy of the results but also underlines the significance of standardized practices in research and clinical settings. As the molecular diagnostic field evolves, understanding these technical aspects becomes crucial for anyone involved in RNA PCR, from students to seasoned researchers.
Sample Collection and Preparation
Sample collection is the first step in the RNA PCR process and is critical to mitigating contamination and ensuring quality. Proper techniques here can significantly affect the test's outcome. Collecting samples requires sterile equipment and appropriate handling procedures. For instance, if you're working with nasopharyngeal swabs for a virus like SARS-CoV-2, the swabs must be made of materials that minimizes the risk of RNA degradation.
Furthermore, the timing of collection also matters. Samples should ideally be taken early in the infection—when viral RNA levels are typically the highest—to improve detection chances. After the sample is gathered, it must be prepared for processing. This may include:
- Isolation of RNA: Extracting RNA from cells requires delicate and appropriate reagents to maintain integrity.
- Elimination of contaminants: Contaminants can throw off the results, leading to false readings.
In short, you want your sample to be as clean and direct as possible—akin to a painter using the right brush to avoid muddied colors.
Types of RNA Targets
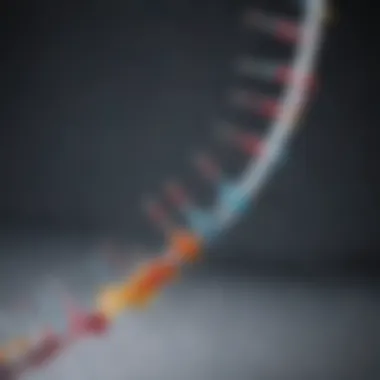
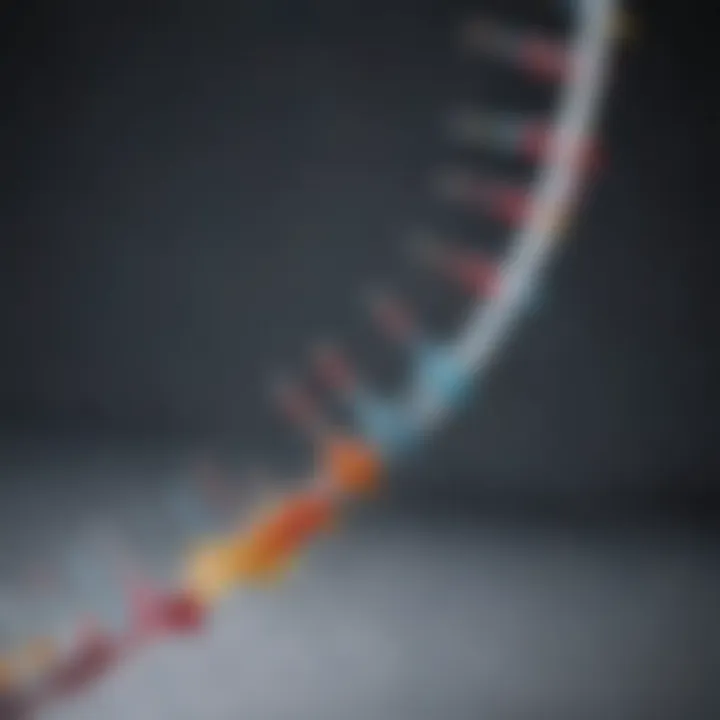
The RNA targets in a PCR test can vary widely, influencing the results and their interpretations. Understanding these targets helps to select the appropriate methods for detection. There are primarily two types of RNA you might come across:
- Messenger RNA (mRNA): This type carries genetic information from DNA to the ribosome, guiding protein synthesis. It's typically present in abundance when a specific gene is being expressed.
- Non-coding RNA (ncRNA): Though it doesn't code for proteins, this RNA plays various roles in regulating gene expression, protein synthesis, and overall cellular functions, making it equally significant in certain contexts.
The choice of RNA target can be dictated by the specific goals of the testing, be it for diagnosing a viral pathogen or studying gene expression patterns in cancer.
Quantitative vs Qualitative PCR
When it comes to PCR tests, the distinction between quantitative and qualitative methodologies is essential for accurately interpreting data.
- Qualitative PCR: This method simply determines whether a target RNA is present or absent in a sample. It’s straightforward—like flipping a switch. You know if there’s a signal, but you can’t gauge how much signal is there.
- Quantitative PCR (qPCR): In contrast, qPCR goes a step further by not only answering the presence question but also providing information on how much target RNA exists. This kind of data can be vital in research areas like personalized medicine, where knowing the RNA levels can influence treatment decisions.
Both types have their place in the lab. Qualitative tests may suffice for a simple diagnosis, but if one needs to track the progression of a disease or treatment response, quantitative measurements are indispensable.
Understanding these technical aspects is not just academic—they directly influence clinical outcomes and the integrity of research findings.
The foundations of RNA PCR are firmly built upon these technical aspects. By focusing on sample integrity, identifying the right RNA targets, and choosing the appropriate PCR methodology, researchers and clinicians can fortify their testing processes. Such diligence guarantees that the results yielded are not just numbers, but vital insights into health and disease.
Interpretation of RNA PCR Results
Interpreting the results of an RNA PCR test is a sophisticated activity that carries substantial weight in both diagnostic and research contexts. The complexity of these results isn't just numbers and figures; they represent the biological reality of a sample, providing insights that can influence clinical decisions and scientific conclusions. Understanding how to interpret these results is not merely academic; it's crucial for making informed decisions about patient care and advancing research.
Understanding Ct Values
The Ct value, or Cycle threshold value, serves as a cornerstone in the interpretation of RNA PCR results. Essentially, it indicates the number of cycles needed for the fluorescent signal to exceed the background level, reflecting the quantity of RNA present in the sample. A lower Ct value suggests a higher amount of RNA, while a higher Ct indicates less RNA.
To better explain this:
- Low Ct Value: This often signifies a viral load high enough to suggest active infection. For instance, in the case of a patient diagnosed with COVID-19, a Ct value of below 30 might lead clinicians to confirm ongoing viral replication.
- High Ct Value: Conversely, a Ct value above 35 could lead one to consider the possibility of a low viral load or residual material from a past infection, rather than active disease.
It’s worth noting that Ct values can differ based on the specific assay used, the target RNA, and the sample type. This variability makes it essential for healthcare providers to understand the context in which these values are generated. Furthermore, comparing Ct values across different tests or platforms without proper calibration can lead to misleading conclusions.
Positive and Negative Controls
In any laboratory setting, controls form an undeniable part of ensuring validity. Positive and negative controls are fundamental components of RNA PCR testing, as they help ascertain that the assay is functioning correctly.
- Positive Controls: These are samples known to contain the target RNA. If the PCR test fails to detect the target in a positive control, it raises immediate red flags about the assay's integrity. Such failures may stem from problems during sample preparation or PCR setup.
- Negative Controls: On the flip side, negative controls do not contain the target RNA. They help identify contamination or false-positive results. If a negative control shows a signal, it suggests that something has gone awry—be it cross-contamination, reagent issues, or improper methodology.
Incorporating both types of controls creates a safety net, ensuring that the results of the RNA PCR test are not just significant but reliable. A result without controls is akin to a ship sailing without a compass, just inviting trouble.
"Control samples are not just technicalities; they’re essential for the credibility of PCR results."
While interpreting RNA PCR results, the overall context is likewise vital. The clinical picture, patient history, and corroborative tests all work hand in hand to provide a comprehensive understanding of the situation at hand. The interplay between Ct values and the reliability of controls underscores a robust framework for accurate diagnostics—paving the way for precise interventions in varied settings.
In summary, decoding the nuances of RNA PCR results requires not just knowledge but also a degree of intuition. As science continues to advance, the methods and technologies will evolve, yet the principles of interpretation will remain integral to making informed decisions in both the clinical and research landscapes.
Challenges and Limitations
When discussing RNA PCR testing, it's crucial to consider the challenges and limitations that arise in its application. The test is a powerful ally in molecular diagnostics, but it is not without its pitfalls. Understanding these intricacies provides a more rounded view of how RNA PCR fits into the larger landscape of diagnostic technologies.
Potential for False Positives and Negatives
One of the most significant concerns surrounding RNA PCR tests is their susceptibility to false positives and negatives. A false positive occurs when the test incorrectly indicates the presence of the target RNA, while a false negative suggests the opposite— that the target RNA is absent when it is, in fact, present. This can have dire consequences in a clinical setting, leading to inappropriate treatment decisions.
False positives can arise from several sources, such as contamination during sample handling or the presence of non-specific amplifications, where other similar sequences are mistaken for the target. Similarly, false negatives often stem from poor sample quality, insufficient target RNA quantity, or the presence of inhibitors in the sample that can affect the PCR reaction.
The reliability of the test hinges on meticulous adherence to protocols. To mitigate these risks, laboratories typically utilize control samples and follow stringent quality control measures. Training personnel to recognize and prevent common pitfalls is vital to maintaining test accuracy.
The accuracy of results from RNA PCR tests is only as solid as the methods and protocols employed during the entire testing process.
Sampling Errors and Quality Control
The procedure of collecting and preparing samples for RNA PCR is as critical as the test itself. Sampling errors can occur at numerous points, from the initial collection of the sample to its transportation and storage.
If samples are not collected correctly or if they degrade before testing, this could lead to unrepresentative results. For instance, if a sample stored at room temperature is used in place of one kept at cooler temperatures, the integrity of the RNA present may be compromised. This degradation can lead to both false negatives and an underestimation of viral loads, which may further complicate treatment plans.
Quality control measures, such as verifying that samples are processed within a specific time frame and preserved under appropriate conditions, are essential to minimizing these errors. Moreover, laboratories often have standard operating procedures that guide personnel through the correct protocols, which in turn helps maintain the reliability of results. Regular audits and validation of techniques also play a key role in ensuring consistent quality across tests.
Overall, while RNA PCR testing is an invaluable tool in diagnostics and research, it is critical to stay vigilant about its limitations. Adequate training, robust protocols, and constant quality control can make a considerable difference in improving the reliability and accuracy of these tests.
Advancements in RNA PCR Technology
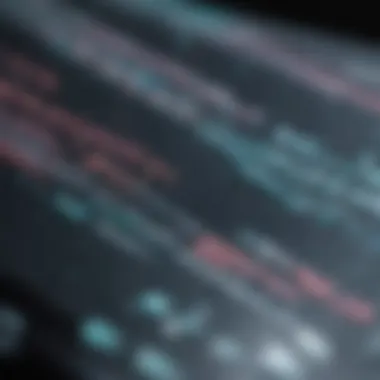
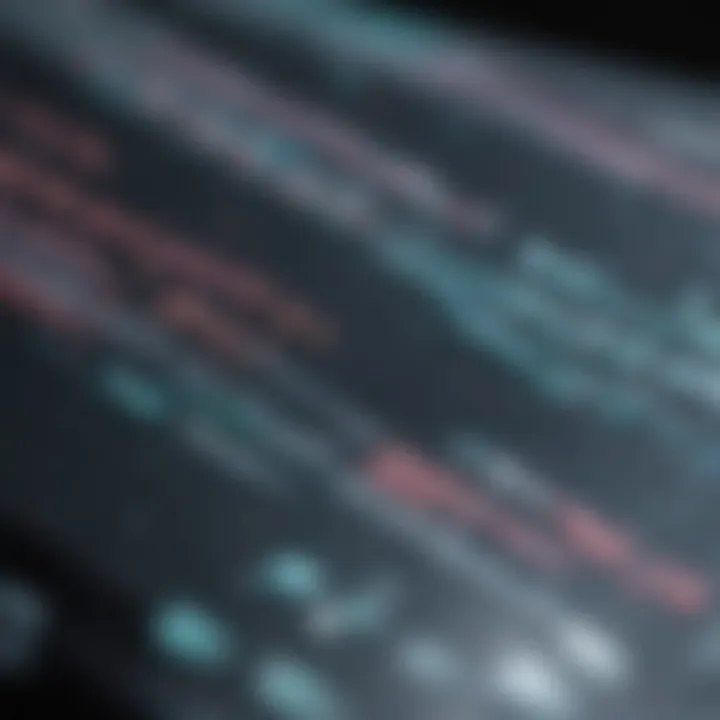
The rapid evolution of RNA polymerase chain reaction (PCR) technology stands as a testament to the dynamic nature of molecular biology. These advancements are crucial for both diagnostic and research applications. By enhancing the accuracy, sensitivity, and speed of RNA detection, modern innovations are pushing the boundaries of what is possible in laboratories around the globe. Understanding these advancements not only highlights their significance in today’s scientific landscape but also offers insights into future trends that may shape healthcare and research methodologies.
Digital PCR Innovations
Digital PCR is carving out a new niche in the world of molecular diagnostics. Unlike traditional PCR, which provides a relative measure of the target RNA, digital PCR quantifies RNA molecules by partitioning samples into thousands of individual reactions. This method is pivotal for achieving ultra-sensitive detection of low-abundance RNA targets.
With the application of microfluidics, samples are divided into nano-droplets, allowing for precise analysis. This partitioning technique results in a robust sensitivity that can identify rare genetic variants or trace viral RNA in complex biological samples.
Some advantages of digital PCR include:
- Increased precision: By measuring individual reactions, it minimizes variation and enhances reproducibility.
- Quantitative results: Absolute quantification of nucleic acids allows for better comparison across different experiments.
- Lower sample volume requirements: Works well even with limited samples, which is especially important in clinical settings where materials may be scarce.
The application of digital PCR can also simplify the workflow in many laboratory processes, thus making significant strides in the efficiency of RNA studies.
Integration with Next-Generation Sequencing
The fusion of RNA PCR with next-generation sequencing (NGS) is another groundbreaking development. This integration allows researchers to overcome the limitations of traditional sequencing methods. RNA PCR enriches the samples for deeper and more comprehensive insights into gene expression.
Combining these technologies leads to a more nuanced understanding of complex biological systems by correlating specific RNA molecules with their functional outcomes. For example, it enhances the detection of transcript variants which might be pivotal in understanding diseases at a molecular level.
Some of the crucial aspects of this integration are:
- High throughput: Facilitates the sequencing of multiple RNA samples simultaneously, thus speeding up the research process.
- Improved detection capability: Allows for the discovery of novel RNA transcripts that could be missed in traditional methods, opening doors for new biomarkers.
- Comprehensive data analysis: The large datasets generated can be mined for patterns that reveal insights into disease progression or responses to therapy.
"Integration with next-generation sequencing is revolutionizing our approach towards understanding RNA biology, creating opportunities for groundbreaking discoveries in medicine and beyond."
The advances in RNA PCR technology, particularly through digital innovations and integration with sequencing methods, not only improve the efficiency of existing workflows but also broaden the scope of research possibilities. As these technologies continue to evolve, the implications for healthcare and scientific research will undoubtedly be profound.
Ethical Considerations
Ethical considerations in RNA PCR testing are increasingly critical as the technology both matures and expands its applications. These considerations ensure that the rights and well-being of individuals are protected, especially when these tests resemble genetic assessments. In an era where data can be easily, and rapidly shared, safeguarding personal information can be a delicate balancing act between scientific progress and individual rights.
Data Privacy in Genetic Testing
Data privacy remains a paramount concern in any discussion surrounding RNA PCR testing. When a test is conducted, results often encompass sensitive information about an individual’s health and, by extension, their genetic makeup. This kind of information, if mishandled, could lead to significant repercussions ranging from discrimination by employers to privacy violations. Patients often enter the arena trusting that their data will be kept under lock and key - a trust that can quickly erode if mishaps occur.
The implications of revealing such data are multifaceted. For example, suppose someone tests positive for a hereditary condition. In that case, the knowledge may impose limitations on their access to certain job opportunities or insurance coverage. Therefore, ensuring robust data protection measures is not just a regulatory obligation but a moral one pertaining to how individuals are treated across various spheres of life.
Moreover, developing clear policies on data storage, who can access the data, and the longevity of data retention is vital. Transparency regarding these issues can foster trust within the community, allowing individuals to feel more comfortable not only undergoing testing but also sharing their results in the context of research.
Informed Consent Issues
Informed consent serves as the cornerstone of ethical medical practice, especially in genetic testing paradigms such as RNA PCR. Patients must be fully aware of what they are consenting to when they undertake these tests. This isn’t just about signing on the dotted line; it’s a thorough understanding of the process, potential outcomes, and the implications these results hold for them and their families.
The notion of informed consent takes on a more profound significance when linked to RNA PCR. The stakes are often high—characters that influence present and future health can emerge from test results, demanding a nuanced approach. However, several barriers can undermine the process:
- Complexity of Information: Genetic testing can be daunting for those without a scientific background. Often, terminology can put up a confusing facade that obscures understanding.
- Pressure and Emotional Impact: When faced with a health scare or family history of disease, individuals may feel pressure to participate in testing without comprehensively grasping what is at stake.
- Trust in Providers: A prevailing issue can also be the perceived authority of healthcare professionals. Patients might simply trust their doctors without fully understanding the implications.
To alleviate these concerns, it is essential for healthcare professionals to engage in candid discussions with patients regarding the scope and ramifications of RNA PCR tests. Clear language, ample opportunity for questions, and a supportive environment can uplift the practice of informed consent to its rightful place in the healthcare system. As a result, individuals would not only consent with knowledge, but also with the peace of mind that their autonomy is respected.
“Informed consent isn’t just a form—it's a conversation that honors the individual's right to understand their health.”
Evaluating these ethical considerations ensures that the community reaps the benefits of RNA PCR testing without compromising personal rights or privacy. By fostering a culture of openness and respect, we maintain the integrity of scientific advancements while prioritizing the dignity of individuals.
Future Directions for RNA PCR Testing
The field of RNA PCR testing is entering a new chapter, one that blends innovation with practicality. This subsection digs into the trajectory of this technology and how it is perking the ears of those in both clinical and research environments. The advances on the horizon hold a promise of boosting diagnostic precision and expanding research applications.
Potential in Personalized Medicine
Personalized medicine tailors treatment to individual patients, and RNA PCR is at the forefront of this revolution. The ability to analyze genetic information through this technique allows for highly customized healthcare solutions. For instance, RNA PCR can help identify specific mutations in cancers, providing insights into the most effective treatments for a particular patient’s tumor profile.
Some key benefits of this application include:
- Targeted therapies: Identifying suitable drugs for specific genetic markers can lead to more effective patient outcomes.
- Reduced trial-and-error: Directing treatments based on genetic profiles eliminates some of the guesswork traditionally involved in medicine.
- Monitoring disease progression: Continuous RNA analysis can provide a roadmap for how a disease evolves in response to treatment, allowing for timely adjustments.
As the technology advances, the integration of RNA PCR in routine screenings is likely to rise. This means that everyone might have access to tailored health insights, leading to early intervention and improved health outcomes.
Expanding Research Frontiers
The potential of RNA PCR extends well beyond personal healthcare to the broad landscape of scientific research. New techniques are cropping up that enhance sensitivity and specificity, making RNA PCR an indispensable tool for researchers. Behavioral nuances of pathogens and disease mechanisms can be dissected and understood with unprecedented clarity.
Here are some areas where RNA PCR is expected to make waves:
- Viral genomic studies: Understanding viral evolution and transmission dynamics through precise RNA profiling can help in outbreak containment and vaccine development.
- Microbiome research: Using RNA PCR to analyze microbial communities allows scientists to explore their relationship with human health.
- Environmental applications: Monitoring RNA sequences in environmental samples can aid in tracking the health of ecosystems and detecting pathogens in various habitats.
"The future is not about knowing everything but knowing what to know," a wise person once said. In the realm of RNA PCR, embracing this mindset could spur breakthroughs that we haven't even imagined yet.