Understanding Murine Myeloma: Insights and Implications
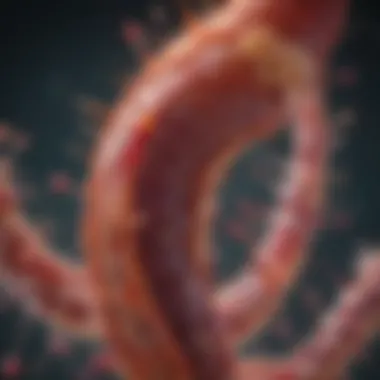
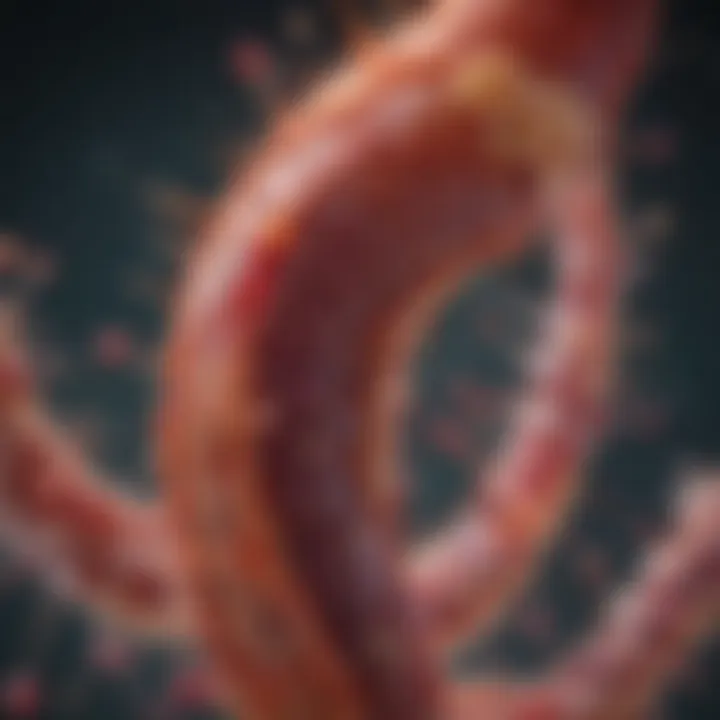
Intro
Murine myeloma serves as a crucial subject in understanding the complexities of multiple myeloma and related diseases. This section sets the stage for discussing various aspects of murine myeloma, including its biological mechanisms, innovative experimental models, and therapeutic implications. By using mouse models to study myeloma, researchers gain insights that are vital for developing effective treatments. This article will guide readers through key topics such as how murine myeloma is studied, the methods employed, the results derived from these studies, and potential future research avenues.
Methodology
Establishing a robust methodology is essential for researching murine myeloma. This entails careful design and strategic data collection techniques to ensure that findings are solid and relevant.
Study Design
In studying murine myeloma, researchers typically adopt a multifaceted approach. Key aspects of study design may include:
- Selecting appropriate murine models that closely mimic human myeloma conditions.
- Employing various induction methods of myeloma in mice, utilizing agents like 5T2MM or a plasmacytoma-inducing virus.
- Utilizing control groups to enhance the validity of results.
- Including a diverse range of tumor cell lines to enhance understanding of disease variability.
Such a framework allows researchers to explore both biological and therapeutic dimensions of murine myeloma effectively.
Data Collection Techniques
To achieve meaningful results, rigorous data collection is fundamental. Common methods of data collection in murine myeloma studies include:
- Histopathological analysis: Examination of histological specimens offers insight into tumor growth and progression.
- Molecular techniques: Using flow cytometry and qPCR to characterize tumor cells at a molecular level.
- In vivo monitoring: Utilizing imaging techniques like bioluminescence to track tumor growth over time.
- Data analysis software: Implementing statistical tools to evaluate treatment efficacy and disease outcomes systematically.
These techniques collectively contribute to a comprehensive understanding of murine myeloma.
Discussion
The interpretation of results derived from murine myeloma studies can lead to significant conclusions. However, it is important to note the limitations and potential future research directions.
Interpretation of Results
Findings from murine myeloma models offer comparative insights into human disease mechanisms. For example, studies show that specific genetic interactions and micro-environmental factors play essential roles in tumor progression. These interpretations help refine existing therapeutic strategies and inform future clinical trials.
Limitations of the Study
Despite their advantages, murine models also present challenges. Some notable limitations include:
- Species differences: Differences between murine and human biology may affect the translatability of findings.
- Model robustness: Certain models might not replicate the full spectrum of human myeloma variability.
- Ethical considerations: The use of animals in research raises ethical discussions regarding the welfare of the subjects.
Future Research Directions
Looking ahead, several avenues hold promise for advancing understanding of murine myeloma:
- Development of more refined models that encompass genetic diversity seen in human patients.
- Investigating new therapeutic agents within the murine context to establish clinical relevance.
- Exploring combination therapies using murine models to enhance treatment efficacy.
Through these future research efforts, we can hope to bridge the gap between murine findings and human clinical outcomes, ultimately contributing to more effective treatments for myeloma.
Foreword to Murine Myeloma
Murine myeloma, a significant area of study in biomedical research, serves as a vital model for understanding human multiple myeloma and related disorders. This section emphasizes the importance of murine myeloma by offering a closer look at its definition and historical context. Understanding murine myeloma can illuminate the mechanisms behind disease progression and foster the development of effective treatment strategies.
Definition and Overview
Murine myeloma refers to a malignancy originating in mouse plasma cells. It is characterized by abnormal proliferation of these cells, leading to various health complications, including bone lesions and immune system dysfunction. The use of murine models is crucial in scientific research, as they mirror many aspects of human disease, enabling researchers to test hypotheses and observe outcomes in a controlled environment.
Murine myeloma is typically induced in laboratory settings, allowing for a thorough investigation of the disease's biological underpinnings and potential therapeutic approaches. The relevance of murine myeloma models lies not only in their genetic similarity to human myeloma but also in their practical advantages. Researchers can manipulate these models genetically, introducing specific mutations or leveraging pharmacological agents to assess their effects.
Historical Context
The study of murine myeloma has evolved significantly over the past century. The first notable murine model was introduced in the early 1960s with the discovery of the C57BL/KaLwRij mouse model, which exhibited spontaneous myeloma development. This was a turning point, providing researchers with a reliable system to study the pathology of myeloma and test novel treatment strategies.
Over the decades, numerous advancements in molecular biology and genetic engineering have further refined these models. For instance, transgenic mouse models now enable the investigation of specific genes and their roles in the development and progression of myeloma. This historical evolution highlights the trajectory of murine myeloma research, illustrating how these models contribute to our ongoing understanding of cancer biology.
"Murine myeloma allows for a controlled environment to study disease mechanisms and test therapies efficiently."
In summary, understanding murine myeloma encompasses both foundational knowledge and the historical perspective that frames current research efforts. This foundation is essential for exploring more complex biological interactions and therapeutic implications as discussed in subsequent sections.
Biological Basis of Murine Myeloma
Understanding the biological basis of murine myeloma is fundamental to deciphering its pathogenesis and progression. This knowledge allows researchers to explore therapeutic strategies effectively. Murine models replicate many human myeloma characteristics, thus offering insights into cellular behaviors and molecular interactions in a controlled environment.
Cellular Composition
Plasma Cells
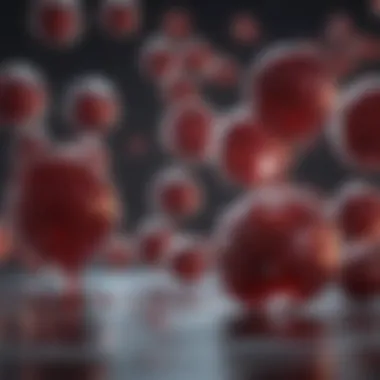
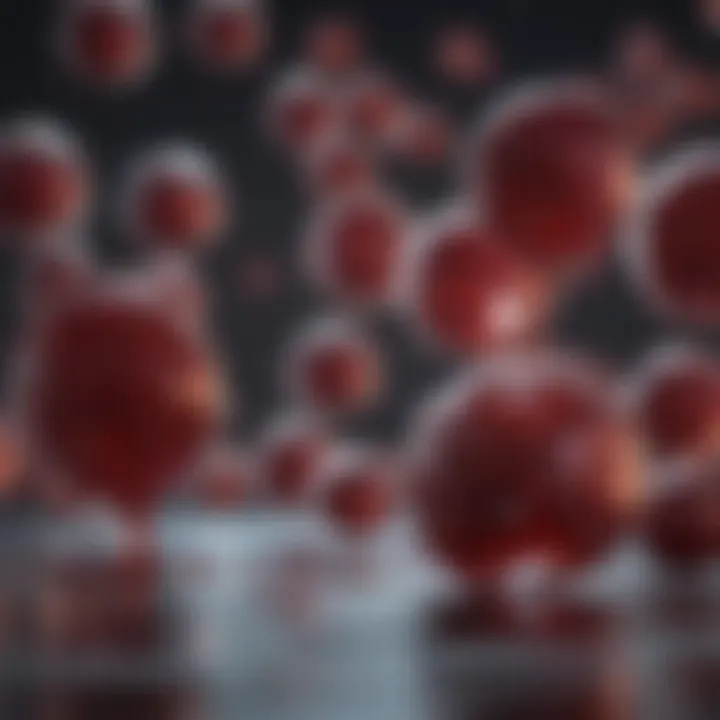
Plasma cells are essential components of the murine myeloma landscape. These cells derive from B lymphocytes and are responsible for producing antibodies. The key characteristic of plasma cells is their ability to secrete large quantities of immunoglobulins. This feature makes plasma cells significant for studying humoral immune response in myeloma. Furthermore, their longevity can contribute to tumor persistence in the microenvironment.
A unique aspect of plasma cells is their high rate of proliferation, which can lead to the accumulation of genetic mutations. This is a double-edged sword; while mutations might drive tumor growth, they also provide targets for therapeutic intervention. The proliferation of plasma cells can lead to increased tumor burden, making it necessary to monitor their behavior closely in experimental models.
Bone Marrow Microenvironment
The bone marrow microenvironment plays a crucial role in the development and survival of myeloma cells. It consists of various cell types, extracellular matrix components, and soluble factors that influence tumor progression. One key feature of the bone marrow microenvironment is its supportive role in maintaining plasma cell survival. This aspect is vital to the persistence of murine myeloma.
Moreover, the unique interaction between myeloma cells and the bone marrow niche can induce changes in the microenvironment, creating a positive feedback loop that fosters tumor growth. Understanding these dynamics is essential as it presents both opportunities and challenges for treatment. For example, targeting these interactions could lead to novel therapeutic strategies. However, the complexity of these relationships also complicates research efforts.
Genetic and Epigenetic Factors
Mutations in Myeloma
Genetic mutations are pivotal in the transformation of normal plasma cells into malignant myeloma cells. These mutations often affect key signaling pathways that control cell growth and survival. The prominent characteristic of mutations in myeloma is their heterogeneity, which reflects the diverse nature of the disease. This heterogeneity complicates treatment, as different mutations may respond variably to therapies.
A significant advantage of studying mutations in murine models is the ability to correlate specific genetic alterations with disease phenotypes. This correlation aids in understanding the stages of myeloma progression, thus facilitating the development of targeted treatments. However, not all mutations have clear therapeutic implications, which can limit their utility in some cases.
Epigenetic Modifications
Epigenetic modifications provide another layer of complexity in myeloma biology. These changes affect gene expression without altering the DNA sequence, thus playing a significant role in tumorigenesis. Key features of epigenetic modifications include DNA methylation and histone modifications. These modifications can either activate or silence genes crucial for cell survival or apoptosis.
Studying epigenetic changes in murine myeloma models is beneficial as it can reveal novel therapeutic targets. Additionally, these modifications can serve as biomarkers for disease progression. However, the reversibility of epigenetic modifications poses both opportunities and challenges for therapy, as reprogramming the epigenome may lead to unintended consequences.
Understanding the biological basis of murine myeloma is crucial for developing targeted therapies that can reshape treatment paradigms and improve patient outcomes.
Models of Murine Myeloma
Models of murine myeloma serve as a critical tool in understanding the complexities of multiple myeloma. These models mimic the disease's genetic and phenotypic characteristics, allowing for in-depth exploration of disease mechanisms and potential treatment approaches. By utilizing different models, researchers can evaluate various aspects of myeloma biology, assess drug efficacy, and uncover new therapeutic targets.
Selecting appropriate models aids in reducing the translational gap between laboratory research and clinical applications. Models can be classified into spontaneous and induced types. Each model presents specific benefits and limitations, which are essential to consider in research. These models will be discussed in the following sections, emphasizing their unique contributions to advancing our understanding of murine myeloma.
Spontaneous Models
Spontaneous models of murine myeloma arise naturally in specific mouse strains. These models help researchers observe the disease progression and its interaction with the immune system in a more physiological context.
C57BL/KaLwRij Mouse Model
The C57BL/KaLwRij mouse model is widely recognized for its significant contribution to studies on murine myeloma. This model exhibits unique tumorigenic characteristics, which closely resemble the features of human multiple myeloma. One key characteristic of the C57BL/KaLwRij mouse is its propensity to develop myeloma spontaneously, providing researchers with a resource that reflects the disease's natural occurring instances.
The primary advantage of utilizing the C57BL/KaLwRij model lies in its ability to reproduce various stages of disease progression accurately. This characteristic makes it a beneficial choice for studying therapeutic responses. However, the model has a limitation in that it may not fully replicate the heterogeneity seen in human myeloma cases.
AKR Mouse Model
The AKR mouse model is another well-established model in murine myeloma research. This model is characterized by a high incidence of myeloma development. The AKR mouse's key characteristic is its genetic predisposition to spontaneous tumor formation, which provides researchers with a system to explore genetic factors influencing myeloma.
A unique feature of the AKR model is its immune system profile, which reflects many aspects of immune response observed in human myeloma. This offers a significant advantage for studying treatments that harness immune mechanisms. However, like other spontaneous models, it presents challenges in replicating the vast heterogeneity found in human counterparts.
Induced Models
Induced models of murine myeloma are generated by exposure to various substances or genetic modifications. These models allow for controlled experimentation, providing insight into tumorigenesis processes and therapeutic interventions.
Chemical Induction
Chemical induction involves administering carcinogens to create a myeloma environment in mice. This approach helps researchers establish a consistent and reproducible model for studying disease mechanisms. One prominent advantage of chemical induction is its ability to mimic the environmental factors that can contribute to the development of myeloma.
This model allows scientists to assess specific chemical interactions with biological processes. However, environmental factors' controlled nature may sometimes limit the model's relevance to natural disease pathways.
Transgenic Models
Transgenic models are engineered to express specific genetic alterations associated with myeloma. This model allows researchers to analyze the effects of particular genes on disease progression and treatment response. The primary characteristic of transgenic models is their ability to replicate specific genetic mutations closely linked to human myeloma cases.
These models offer a clear advantage for understanding the molecular mechanisms driving myeloma and potential targeted therapies. Yet, introducing genetic modifications may yield results that do not fully represent the heterogeneous nature of human myeloma.
Xenograft Models
Xenograft models use human myeloma cells implanted into immunocompromised mice, providing a platform for evaluating human tumor biology in a living organism. These models are crucial for assessing treatment efficacy in human-like conditions.
Human Myeloma Cell Lines
Using human myeloma cell lines in xenograft models provides unique insight into tumor behavior and therapeutic response dynamics. The most notable characteristic of these models is their ability to directly test human-derived treatments.
The advantage lies in replicating human myeloma's complexity and aggressiveness. However, results may not always reflect the interactions of a fully functional immune system, as the mice used are immunocompromised.
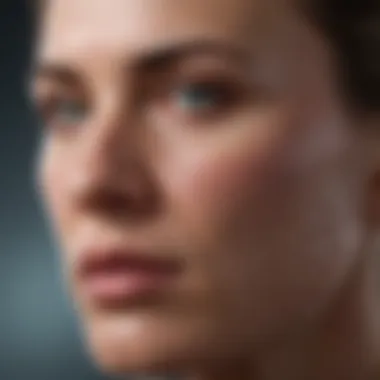
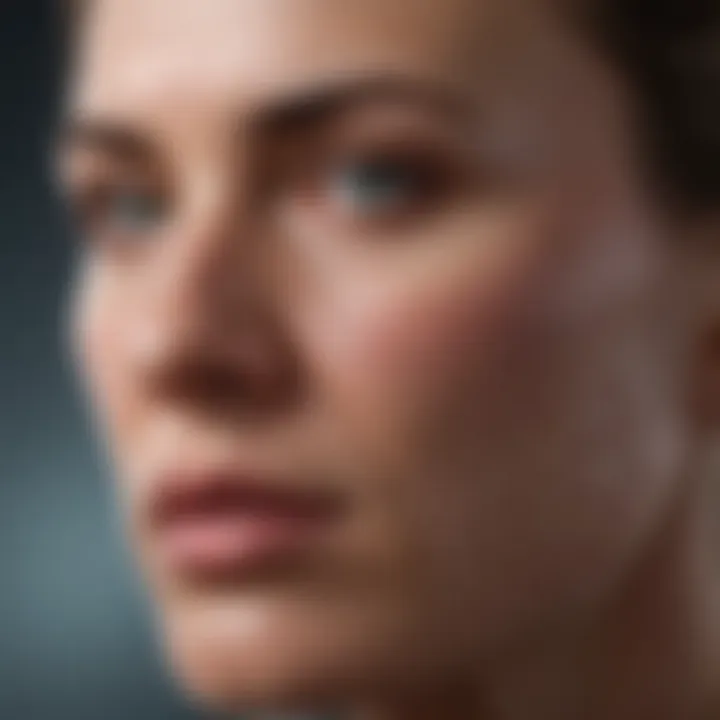
In Vivo Studies
In vivo studies entail researching the live interaction of treatments and tumors in a whole organism. This approach contributes significantly to understanding and developing novel therapies for myeloma. One of the key advantages of in vivo studies is the ability to observe the entire biological context, including immune responses.
However, controlling experimental conditions can be challenging. Variability in the host response may introduce unpredictability in results.
Models of murine myeloma play a pivotal role in advancing our understanding of the disease. Each model type presents unique contributions and challenges. As research progresses, these models will continue to offer valuable insights into improving diagnosis and treatment for myeloma.
Pathophysiology of Murine Myeloma
The pathophysiology of murine myeloma is critical for understanding the disease's development and progression. It highlights essential interactions within the tumor microenvironment and the immune responses elicited by myeloma cells. Grasping these elements helps researchers develop better models and treatments, ultimately influencing therapeutic strategies. The complexity of murine myeloma's pathophysiology underscores its relevance as a research model for human disease.
Tumor Microenvironment Interaction
The tumor microenvironment plays a significant role in murine myeloma. It consists of various cellular and non-cellular components, such as stromal cells, extracellular matrix proteins, and soluble factors. These components create a niche that supports the growth and survival of myeloma cells.
Key interactions include:
- Stromal Cells: These cells provide critical support to myeloma cells. They secrete growth factors that promote proliferation and survival, thus influencing tumor aggressiveness.
- Cytokines: A range of cytokines are involved in shielding myeloma cells from apoptosis. They can create an immunosuppressive environment, ultimately hindering effective anti-tumor immune responses.
- Extracellular Matrix: The extracellular matrix can be modified in ways that favor myeloma cell adhesion, migration, and invasion. This dynamic between the matrix and tumor cells can promote disease unfoldment and metastasis into other tissues.
Studying these interactions in murine models allows for insights into therapeutic targets that can disrupt this supportive microenvironment. This knowledge may inform strategies like metronomic chemotherapy or interventions targeting specific cytokines.
Immune Response to Myeloma
The immune response to murine myeloma is complex and often ineffective against the tumor. Myeloma cells can evade immune detection and suppress the immune system. Understanding these mechanisms can lead to enhanced therapeutic approaches.
Several elements characterize the immune response:
- Tumor Antigens: Myeloma cells express specific antigens that can trigger an immune response. However, they often mutate, leading to changes that help them evade detection.
- T-Cell Activation: The activation of T-cells is crucial for targeting tumors. In myeloma, T-cell responses are often suppressed, diminishing their ability to respond effectively to the tumor.
- Immune Checkpoints: Myeloma exploits immune checkpoint pathways, like PD-1/PD-L1 interactions. This exploitation allows myeloma cells to escape immune surveillance.
By unraveling these immune responses, researchers can explore immunotherapy options, such as monoclonal antibodies or checkpoint inhibitors. These therapies hold the potential to augment the immune system's ability to target and destroy myeloma cells effectively.
"The interaction of murine myeloma with its microenvironment and the immune system forms the basis for exploring potential therapeutic options and understanding disease dynamics."
In summary, the pathophysiology of murine myeloma encompasses intricate relationships between tumor cells, their microenvironment, and the immune system. Continued research in this area presents invaluable opportunities for advancing therapeutic designs and improving outcomes for multiple myeloma patients.
Diagnostic Approaches
The diagnostic approaches in murine myeloma research serve as a critical foundation for understanding the complexities of the disease. They not only aid in identifying the presence and progression of myeloma but also inform treatment strategies. Accurate diagnosis allows for effective monitoring of therapeutic responses, ultimately influencing patient outcomes. Murine models are particularly insightful in this regard, providing researchers with tools to develop and evaluate various diagnostic methods that can be translated to human medicine.
Biomarkers for Diagnosis
Serological Markers
Serological markers play a pivotal role in the diagnosis of murine myeloma. These are substances found in the blood that can signal the presence of myeloma cells or the disease itself. One key characteristic of serological markers is their ability to provide quantitative measurements, which can be essential for assessing disease severity and progression. For instance, elevated levels of immunoglobulin proteins or light chains can indicate active myeloma. The popularity of serological markers lies in their non-invasive nature, allowing for repeated testing without significant discomfort to the subject.
However, while serological markers are beneficial, they come with limitations. One unique feature of these markers is that they can sometimes yield false positives or negatives, complicating the diagnostic process. Additionally, certain markers may vary widely among individual mice, leading to discrepancies in interpretation. Thus, while they are indispensable tools, caution and rigorous validation are necessary when relying on serological markers for diagnostic purposes.
Imaging Techniques
Imaging techniques are another essential component in the diagnostic landscape of murine myeloma. Techniques such as magnetic resonance imaging (MRI) or positron emission tomography (PET) are particularly useful for visualizing tumor development and assessing the overall burden of the disease. The key characteristic of imaging techniques is their ability to provide a comprehensive view of the tumor's location and size, which is vital for effective treatment planning.
These techniques are increasingly regarded as beneficial choices for researchers studying myeloma because they allow for real-time monitoring of tumor response to therapy. A notable advantage of imaging techniques is their capacity to detect changes before they are reflected in serological markers. However, these methods can be expensive and require specialized equipment, which may be a barrier for some research facilities. Moreover, complications may arise due to the need for anesthesia and restraint during imaging procedures, which could potentially affect the subject's health.
Histopathological Assessment
Histopathological assessment is a cornerstone of diagnosing murine myeloma. This method involves the microscopic examination of tissue samples to identify specific cellular characteristics associated with myeloma. Through histopathology, researchers can visualize the morphology of plasma cells and assess their distribution within the bone marrow environment. This technique provides profound insights into the pathological state of the disease, enabling a clear understanding of its progression.
Histopathological techniques are particularly integral when validating the findings obtained through serological markers and imaging methods. They allow for a comprehensive evaluation that combines biochemical indicators with histological evidence, offering a more holistic view of the myeloma's behavior and its implications.
Moreover, these assessments can uncover insights into the tumor microenvironment, thus enhancing the overall understanding of disease mechanisms. However, histopathological assessment requires careful preparation of tissue samples and may rely on subjective interpretation, which can introduce variability. Ensuring standardization in this practice is crucial to maintaining consistency in diagnostic outcomes.
Current Therapeutics in Myeloma Research
Current therapeutics in myeloma research plays a critical role in addressing this complex condition. Understanding the available treatment options offers insights into the evolving landscape of myeloma therapies. Various strategies have emerged, enhancing the effectiveness of treatment and improving patient outcomes. Recent years have seen advancements in pharmacological interventions, highlighting the importance of exploring both chemotherapy and immunotherapy.
Chemotherapy and Targeted Therapy
Chemotherapy remains a staple in myeloma management, aimed at rapidly dividing cancer cells. Traditional chemotherapeutic agents, such as melphalan and cyclophosphamide, contribute significantly to the overall treatment protocols. Many patients benefit from these agents, particularly during the early stages of the disease.
Targeted therapy, on the other hand, represents a shift from conventional chemotherapy. This approach focuses on specific genetic markers and pathways involved in myeloma progression. For instance, proteasome inhibitors like bortezomib target the proteasome's function, which is often upregulated in myeloma cells. These therapies have revolutionized treatment modalities, offering options that are more aligned with the patient's genetic profile and the tumor's biology.
Immunotherapy Approaches
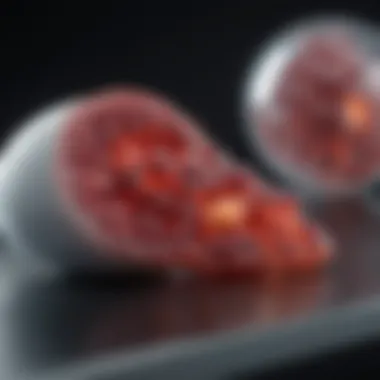
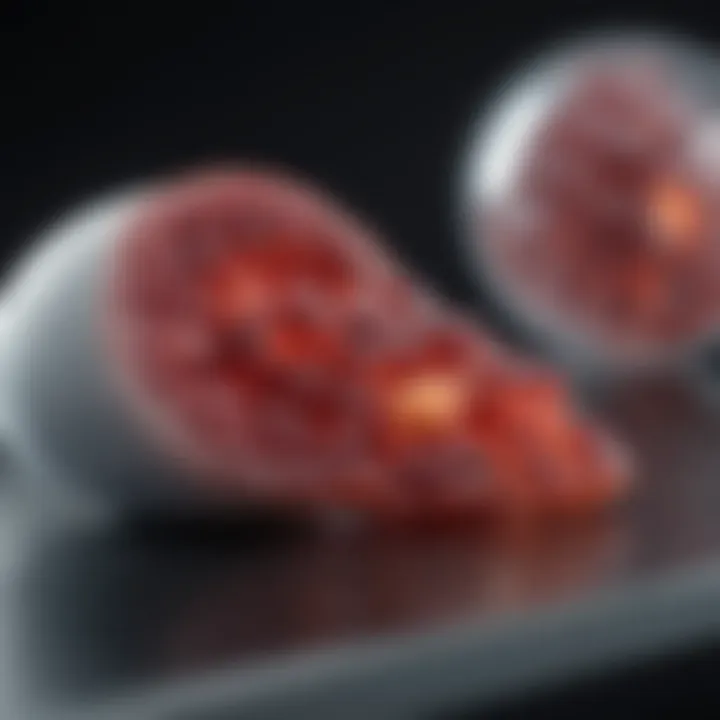
Immunotherapy has emerged as a promising frontier in myeloma treatment. By leveraging the body’s immune system, these therapies aim to recognize and eliminate myeloma cells more effectively than traditional methods. Two prominent approaches in this realm include monoclonal antibodies and CAR T-cell therapy.
Monoclonal Antibodies
Monoclonal antibodies are engineered to target specific antigens present on the surface of myeloma cells. They provide a tailored approach to treatment, minimizing off-target effects. One significant characteristic of monoclonal antibodies is their ability to engage the immune system, enhancing its response against malignancies. These agents have gained popularity due to their favorable safety profile and effective use in various stages of myeloma.
A unique feature of monoclonal antibodies is their capacity to deliver cytotoxic agents directly to tumor cells. This mechanism not only maximizes treatment efficacy but also reduces systemic toxicity. Despite these advantages, challenges exist, including potential resistance mechanisms and the need for combination strategies to avoid relapse.
CAR T-Cell Therapy
CAR T-cell therapy represents another transformative approach in myeloma management. This technique involves reengineering a patient’s T-cells to express chimeric antigen receptors that specifically target myeloma cells. The key characteristic of CAR T-cell therapy lies in its personalized nature. This therapy is tailored specifically for the individual patient, which enhances the likelihood of success.
The unique feature of this therapy is its capacity for long-term monitoring, as modified T-cells can persist in the body for extended periods. This allows them to respond swiftly should the disease re-emerge. However, CAR T-cell therapy also poses challenges, such as the potential for severe cytokine release syndrome and neurotoxicity. Balancing these risks with the benefits is crucial for optimizing treatment outcomes.
Current advances in myeloma therapeutics highlight the dynamic nature of research in this area. Approaches combining traditional methods with cutting-edge therapies could lead to better patient outcomes and survival rates.
Both chemotherapy and immunotherapy represent pivotal components in the therapeutic landscape of murine myeloma research. As scientists continue to explore innovative treatments, these strategies will play a vital role in enhancing the understanding and management of this complex disease.
Challenges in Murine Myeloma Research
Understanding the challenges in murine myeloma research is critical for several reasons. This field has substantial implications for not only advancing our knowledge of multiple myeloma but also in developing therapeutic strategies that can be translated to human patients. Working with murine models can yield valuable insights, yet there are inherent difficulties. Recognizing these challenges allows researchers to refine their methodologies, enhance their experimental designs, and ultimately improve their findings.
Translatability to Human Myeloma
Despite the utility of murine models, the translatability of findings from these models to human myeloma presents a significant challenge. Murine myeloma does not entirely replicate the complexity observed in human disease. For instance, differences in immune response, tumor growth, and microenvironmental factors can affect how well results in mice predict potential outcomes in human patients.
Common discrepancies include:
- Genetic Differences: Genetic variations exist between murine models and human patients, affecting disease progression and treatment responses.
- Microenvironment: While murine models can exhibit a tumor microenvironment similar to humans, it often lacks the full complexity of human bone marrow. Consequently, responses to therapies may not align.
To address these issues, researchers must remain vigilant about the limitations and strengths of murine models. Collaborative efforts and improved experimental designs may help bridge the gap for better translatable results.
Ethical Considerations in Research
The ethical considerations surrounding murine myeloma research are paramount. Firstly, the use of animals in research prompts a demand for clear justifications regarding the necessity of such models in studying complex diseases like myeloma. Scientists have the responsibility to demonstrate that the potential benefits of their research outweigh the ethical concerns involved.
Key ethical considerations include:
- Animal Welfare: Researchers must prioritize humane treatment and care for laboratory mice. Protocols should ensure minimal suffering, with oversight by institutional animal care committees.
- Scientific Justification: Each study must have solid grounding in scientifically sound rationale. Researchers need to articulate how murine models will contribute meaningful insights into human myeloma, rather than using them for exploratory studies without clear aims.
By adopting stringent ethical guidelines, researchers can mitigate ethical concerns while ensuring that their work contributes valuably to the understanding of myeloma advancements.
Future Directions and Research Opportunities
The ongoing exploration of murine myeloma presents an essential pathway for advancing our understanding of this complex disease. The study of murine models provides key insights that can guide therapeutic developments and improve patient outcomes. It enables researchers to evaluate not just current treatment modalities but also innovate emerging therapies that can address the unique challenges posed by myeloma. As the scientific community delves deeper into murine myeloma, several areas of future research opportunities become increasingly relevant.
Emerging Treatments
The development of emerging treatments is a frontier for murine myeloma research. Researchers are now exploring various avenues, including novel drug combinations and targeted therapies. For instance, small molecule inhibitors and next-generation immunotherapies have shown promising results in preclinical studies. Antibody-drug conjugates, which link potent drugs to antibodies targeting specific myeloma markers, are becoming topics of intense investigation. Trials investigating these treatments may reveal more effective therapeutic strategies utilizing murine models to predict human responses.
Furthermore, myeloma's heterogeneity necessitates a continuous effort to identify new targets. This includes exploring pathways that are actually critical for tumor survival. With advances in high-throughput sequencing, researchers can uncover unique molecular signatures that guide the development of precise treatments. The use of murine models remains pivotal to validate these findings and transition them from laboratory to clinic effectively.
Personalized Medicine in Myeloma
The concept of personalized medicine is gaining momentum in the treatment of myeloma. The heterogeneity of tumors signifies a distinct need for tailored therapies that consider individual patient profiles, including genetic and epigenetic factors. Murine models can play a significant role in this paradigm by helping researchers develop stratified treatment protocols based on the biological characteristics of each patient's disease.
For instance, integrating genomic data allows for the discovery of specific mutations associated with different myeloma subtypes. By employing murine models expressing these mutations, researchers can simulate drug responses and evaluate efficacy before advancing to clinical trials. This approach not only enhances treatment efficiency but also minimizes adverse effects through more accurate patient selection.
Epilogue
The conclusion of this article serves as a significant reflection on the value of murine myeloma research. It encapsulates the essential findings discussed throughout the sections without reiterating every detail. This synthesis highlights the intricate biology of murine myeloma and elucidates its role as a valuable tool in experimental research.
Furthermore, it emphasizes the necessity of continued exploration within this domain. Understanding murine models helps to bridge the gap between basic science and clinical application, which is foundational for the advancement of therapeutics in multiple myeloma. This ongoing research can potentially lead to innovative treatment strategies, benefiting current patient care and outcomes.
Additionally, the importance of ethical considerations is also underlined. As research progresses, it becomes critical to balance scientific inquiry with moral responsibility toward the use of animal models.
Summary of Findings
This article detailed various critical aspects of murine myeloma, elucidating the underlying biological mechanisms. Key findings include:
- Biological Basis: The cellular composition and genetic factors contribute significantly to murine myeloma pathology.
- Experimental Models: An analysis of spontaneous, induced, and xenograft models demonstrates their significance for research purposes.
- Therapeutics: Current therapies, including chemotherapy and immunotherapy, exemplify potential pathways to improve treatment outcomes.
- Research Challenges: Issues like translatability to human conditions and ethical concerns present ongoing challenges that require careful consideration.
The synthesis of these findings reinforces the relevance of murine myeloma as a crucial area in biomedical research, opening pathways for future research avenues.
Importance of Murine Models
Murine models play a pivotal role in our understanding of myeloma and similar diseases. Their importance can be summarized as follows:
- Representing Disease: These models closely mimic the human disease process, providing invaluable insights into its pathophysiology and progression.
- Testing Therapies: Murine models are critical in evaluating the efficacy and safety of new therapeutic agents before clinical trials in humans.
- Genetic Studies: The ability to manipulate the murine genome allows researchers to explore the impact of specific genetic changes on myeloma development and response to treatment.
- Cost-Effectiveness: Working with murine models is often more practical and cost-effective than other experimental approaches.
The utilization of murine models contributes significantly to the understanding and potential treatment of myeloma, making them indispensable in ongoing research.