Understanding Lung Ventilation: Mechanisms and Health
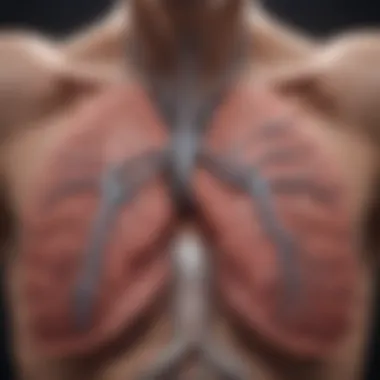
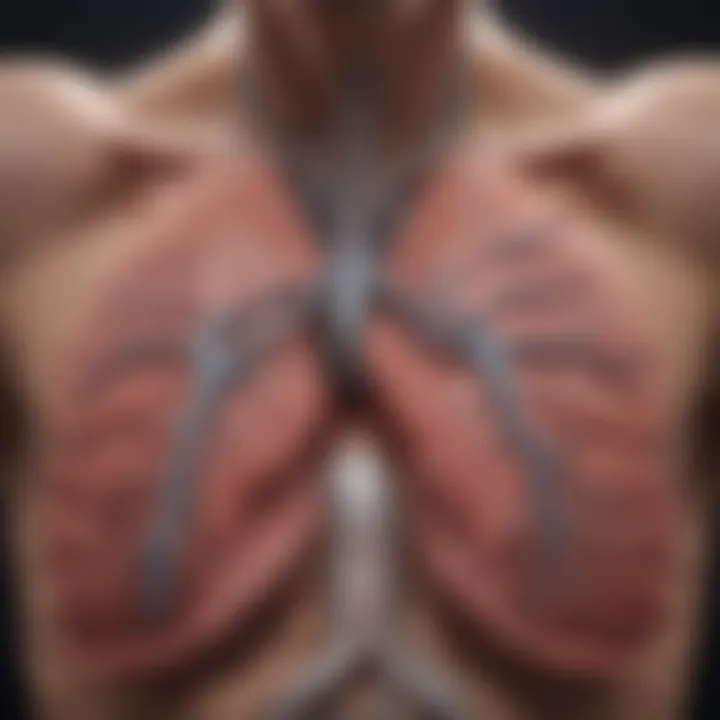
Intro
Ventilation in the lungs is a fundamental process that impacts our overall health. It involves the movement of air into and out of the lungs, allowing for the exchange of oxygen and carbon dioxide. Understanding this process requires an exploration of the underlying physiological mechanisms. Effective ventilation is key for maintaining homeostasis, especially during physical exertion or in high-altitude conditions. This article will examine various aspects of lung ventilation, including its regulation, the roles of specific muscles and anatomical structures, and the potential disorders that can interfere with this critical function.
Methodology
Study Design
A comprehensive overview of lung ventilation calls for a thorough approach that combines both qualitative and quantitative data. Observational studies, along with experimental designs, could provide valuable insights into the physiological aspects of ventilation. This can include examining lung function tests and respiratory rate measurements under varying conditions, such as exercise and altitude.
Data Collection Techniques
Data collection can utilize several techniques, such as:
- Spirometry: This measures the volume and flow of air during inhalation and exhalation, providing key metrics of lung function.
- Blood Gas Analysis: This evaluates the composition of gases in the blood, offering insights into how well oxygen and carbon dioxide are being exchanged in the lungs.
- Imaging Techniques: Chest X-rays or CT scans can help visualize structural abnormalities that might impede ventilation.
- Surveys and Questionnaires: These can assess symptoms and quality of life in individuals with respiratory disorders, shedding light on how various conditions can affect lung ventilation.
Discussion
Interpretation of Results
The results from these studies can shed light on the efficiency of ventilation. If findings reveal deviations in gas exchange or abnormal respiratory patterns, it could indicate underlying issues such as chronic obstructive pulmonary disease or asthma. Understanding these results is crucial for developing effective treatment strategies.
Limitations of the Study
While studying ventilation, several limitations can arise:
- Sample size may not be representative of the broader population.
- Variability in individual physiology can complicate results.
- Environmental factors may influence outcomes and are hard to control.
Future Research Directions
Further research is needed in the following areas:
- Investigating the impact of various interventions on lung ventilation, such as physical therapy and pharmacotherapy.
- Exploring the role of nutrition and hydration in respiratory health.
- Understanding how technological advancements, like artificial intelligence, can improve diagnostics in lung diseases.
Efficient ventilation not only affects physical performance but also plays a crucial role in disease prevention and management.
In summary, by taking a granular look at lung ventilation, we can understand its significance in both healthy and diseased states. This will help us appreciate the intricate interplay between our respiratory system and overall health.
Understanding Lung Ventilation
Understanding lung ventilation is crucial as it lays the foundation for comprehending how our body facilitates breathing. This section will provide insight into what lung ventilation entails, its definition, and its significance in the respiration process. Effective ventilation not only ensures an adequate supply of oxygen but also plays a key role in the removal of carbon dioxide, which is vital for maintaining the body’s pH balance.
Definition of Ventilation
Ventilation refers to the process by which air is moved in and out of the lungs. It encompasses two primary phases: inspiration, where air is inhaled, and expiration, where air is exhaled. Volumes of air exchanged during these phases can vary based on several factors, including physical activity levels, lung health, and overall fitness.
Specific parameters define ventilation:
- Tidal Volume (TV): The amount of air inhaled or exhaled in a single breath.
- Respiratory Rate (RR): The number of breaths taken per minute.
- Minute Ventilation: The total volume of air entering and leaving the lungs in one minute, calculated as the product of tidal volume and respiratory rate.
Importance of Ventilation in Respiration
Ventilation plays a critical role in the respiratory process. It is not just about oxygen intake; it also involves efficient gas exchange, which is necessary for cellular function. Proper ventilation helps to:
- Maintain adequate oxygen levels, which is essential for energy production in cells.
- Facilitate the removal of carbon dioxide, a metabolic waste product that can lead to acidosis if not expelled from the body.
In summary, understanding lung ventilation is paramount for anyone studying respiratory physiology. It sets the stage for a deeper exploration of related topics, such as respiratory efficiency, disorders affecting ventilation, and overall health impacts.
Anatomy of the Respiratory System
The anatomy of the respiratory system is fundamental to understanding how ventilation occurs in the lungs. This system comprises several structures that work together to facilitate gas exchange and maintain respiratory efficiency. The major components include the nasal cavity, pharynx, larynx, trachea, bronchi, and lungs. Each element plays a distinct role in the conduction of air and the processes that follow. Knowing these structures is essential for grasping how the lungs operate and how disorders may arise.
Structures Involved in Ventilation
The primary structures involved in ventilation can be categorized into conducting zones and respiratory zones. The conducting zone includes the nasal cavity, pharynx, larynx, trachea, bronchi, and bronchioles. Each of these parts serves to filter, warm, and moisten the air inhaled before it reaches the lungs.
- Nasal Cavity: Air enters through the nasal cavity, which is lined with mucous membranes and cilia. This structure warms and humidifies the incoming air while trapping dust and pathogens.
- Pharynx and Larynx: The pharynx serves as a passageway for both air and food. The larynx, also known as the voice box, contains the vocal cords and protects the trachea against food aspiration.
- Trachea: This is a rigid tube that conducts air down to the bronchi, held open by C-shaped cartilage rings.
- Bronchi and Bronchioles: The trachea bifurcates into the right and left bronchi, which lead to the lungs. Once inside the lungs, bronchi further divide into smaller bronchioles. This branching network increases surface area for gas exchange while regulating airflow.
- Lungs: The lungs reside within the thoracic cavity and contain the alveoli, the air sacs where gas exchange occurs. Each lung is divided into lobes, further optimizing space and function.
These structures are indispensable in creating a pressure differential that allows air movement. Dysfunction in any part of this system can lead to insufficient ventilation and health complications.
Gas Exchange Surfaces
The surfaces where gas exchange occurs are primarily found in the alveoli. These tiny air sacs are surrounded by a network of capillaries. The alveolar walls are thin, allowing for efficient diffusion of oxygen and carbon dioxide.
- Alveolar Structure: The alveoli are lined with a surfactant, which decreases surface tension and prevents collapse. It is crucial for maintaining stability during breathing cycles.
- Capillary Network: Each alveolus has an extensive capillary network. This is where the exchange of gases occurs—oxygen diffuses into the blood while carbon dioxide diffuses out into the alveoli to be exhaled.
The functionality of these gas exchange surfaces is influenced by factors such as surface area, diffusion distance, and the partial pressure of gases. Any alteration in these elements can significantly impact respiratory efficiency and overall health. Understanding these basic anatomical and physiological properties is crucial for deeper study in respiratory medicine and related fields.
Physiology of Ventilation
The physiology of ventilation is fundamental to understanding how the lungs function in health and disease. This section illustrates the intricate mechanics of breathing, the muscles involved, and the physiological principles at play. By exploring these components, one gains insights into respiratory efficiency, the delicate balance of gas exchange, and their importance for overall well-being.
Mechanics of Breathing
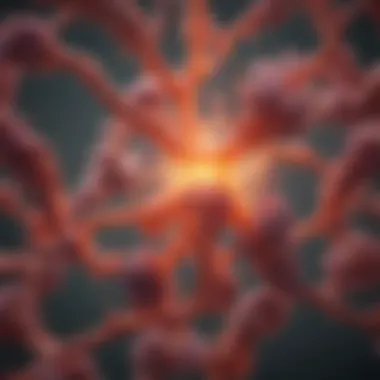
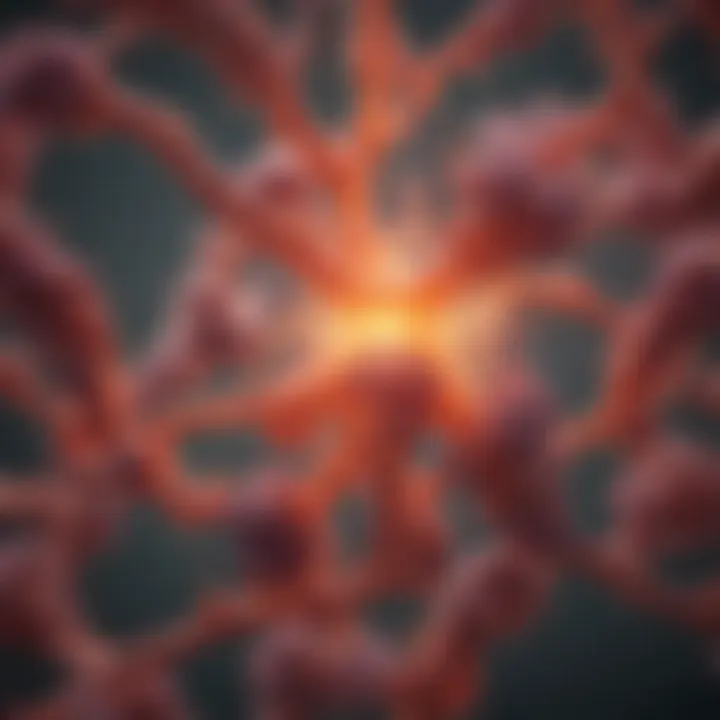
Breathing is a mechanical process, primarily driven by pressure changes within the thoracic cavity. When inhalation occurs, the diaphragm contracts and moves downward. This action increases the volume of the thoracic cavity, causing intrapleural pressure to decrease. As a result, air from the external environment enters the lungs to equalize the pressure.
During exhalation, the diaphragm relaxes, and the elasticity of the lung tissue helps expel air. The rib cage also plays a role here; the intercostal muscles contract to lift the ribs during inhalation and relax during exhalation.
Proper mechanics of breathing are crucial for maintaining adequate gas exchange and ensuring oxygen delivery throughout the body.
Factors such as body position, lung compliance, and the presence of respiratory diseases can influence the mechanics of breathing. In healthy individuals, these cycles occur seamlessly, but any disruption can lead to hypoventilation or hyperventilation, affecting gas exchange efficiency.
Role of Diaphragm and Intercostal Muscles
The diaphragm and intercostal muscles are the primary muscles involved in the process of ventilation. The diaphragm is a dome-shaped muscle that separates the thoracic cavity from the abdominal cavity. Its movement is essential for creating negative pressure during inhalation.
Intercostal muscles, located between the ribs, assist the diaphragm by expanding and contracting the rib cage. They are divided into internal and external intercostals:
- External intercostals: These muscles facilitate inhalation by lifting the rib cage upward and outward, aiding thoracic expansion.
- Internal intercostals: These muscles help with forced exhalation by pulling the rib cage down and inward, which reduces the thoracic volume.
These muscles work in concert, enabling smooth transitions between inhalation and exhalation. If either muscle group is weakened or compromised—due to conditions like myasthenia gravis or spinal cord injuries—the efficiency of breathing can decrease. Understanding the roles of these muscles is vital for diagnosing and managing respiratory issues.
In summary, the physiology of ventilation relates closely to the functional aspects of breathing. It emphasizes the need for effective mechanics and the intricate roles muscles play in this process. A robust understanding of these elements is essential for professionals in healthcare and research fields, as it provides a framework for addressing respiratory disorders.
Regulatory Mechanisms of Ventilation
Understanding the regulatory mechanisms of ventilation is critical for comprehending how the body maintains effective gas exchange. These mechanisms ensure that ventilation matches the metabolic demands of tissues and supports homeostasis. The body must constantly adjust breathing patterns based on varying physical activities and environmental conditions. By examining neural and chemical controls of breathing, one can appreciate how intricate and responsive the ventilation system is.
Neural Control of Breathing
Neural control plays a vital role in the regulation of breathing patterns. The respiratory center in the brainstem, particularly in the medulla oblongata and pons, coordinates the automatic processes of inhalation and exhalation. This center integrates sensory information from various body systems and sends appropriate signals to the respiratory muscles.
Key elements in neural control include:
- Central Pattern Generators: Neurons in the medulla help generate rhythmic breathing patterns. They initiate and regulate breathing rates based on body needs.
- Chemoreceptors: Located in the carotid arteries and the aorta, these receptors detect changes in blood chemistry, including carbon dioxide and oxygen levels. The information leads to adjustments in ventilation to restore balance.
- Higher Brain Centers: Thoughts, emotions, and reflexes can also influence breathing. For example, stress or exercise can heighten breathing rates, showcasing the link between neural functions and ventilation.
"The control of breathing is an exquisite balance between voluntary actions and automatic responses to physiological signals."
Maintaining proper ventilation is a dynamic process that requires constant adjustment to both internal and external stimuli.
Chemical Regulation of Ventilation
Chemical regulation complements neural control, ensuring that ventilation reflects the metabolic state of the body. This mechanism primarily involves monitoring the levels of carbon dioxide, oxygen, and pH in the blood.
- Carbon Dioxide Levels: An increase in carbon dioxide levels can lead to acidosis, prompting the respiratory center to increase the rate and depth of breathing. This expels more CO2 and helps normalize blood pH.
- Oxygen Levels: While the body relies more on CO2 levels for regulation, low oxygen levels can trigger an increase in respiratory rate as well. This response is crucial in conditions of hypoxia, helping to restore oxygen balance.
- pH Levels: The body's acid-base balance is crucial for normal function. Changes in pH are often linked to CO2 levels. Thus, breathing adjusts to correct any imbalances detected by chemoreceptors.
Chemically mediated responses provide an essential and rapid way for the body to adapt to varying needs. These regulatory mechanisms illustrate the precision of homeostasis and the significance of efficient gas exchange in overall health.
Factors Affecting Lung Ventilation
Understanding factors affecting lung ventilation is crucial for grasping its role in respiratory health. Effective ventilation is key to ensuring the body receives sufficient oxygen and expels carbon dioxide. Multiple elements interact to influence how well the lungs function. These factors can have significant implications for various populations, including athletes, individuals with respiratory conditions, or anyone living at high altitudes.
Intrapleural Pressure
Intrapleural pressure plays an essential role in maintaining lung ventilation. It is the pressure within the pleural cavity, which is the space between the lungs and the chest wall. This pressure remains negative under normal conditions, which helps keep the lungs inflated.
When the intrapleural pressure decreases during inhalation, it creates a gradient that allows air to flow into the lungs. Conversely, any significant changes in this pressure can lead to atelectasis, where sections of the lung collapse. Conditions such as pneumothorax, where air enters the pleural space, can drastically affect intrapleural pressure, leading to reduced ventilation.
Proper maintenance of intrapleural pressure is critical for respiratory efficiency and overall lung health.
Tidal Volume and Respiratory Rate
Tidal volume refers to the amount of air inhaled or exhaled in a single breath during normal rest. It is a direct indicator of the efficiency of ventilation. In contrast, respiratory rate is the number of breaths taken per minute. Both tidal volume and respiratory rate are dynamic and can change based on various factors such as exercise, altitude, and health status.
The relationship between tidal volume and respiratory rate can be summarized as follows:
- Increased physical activity often leads to an increase in both tidal volume and respiratory rate. This allows for greater oxygen intake to meet the body’s elevated demands.
- At high altitudes, reduced oxygen levels may cause a change in both parameters as the body tries to adapt.
In clinical settings, monitoring these factors can help in assessing lung function and determining the presence of any ventilation disorders.
Overall, recognizing how these elements interact helps to appreciate their importance in both health and disease.
Measurement of Ventilation
Measuring ventilation is crucial for understanding respiratory health. It provides data on how well air is moving in and out of the lungs. This measurement helps in diagnosing respiratory disorders and monitoring treatment effectiveness. Accurate assessment can guide clinical decisions and improve patient outcomes.
Ventilation measurement typically consists of several techniques. Each of them has specific benefits and considerations. Evaluating the method chosen is essential to ensure reliable results. The importance of these measurements cannot be overstated, as they relate directly to the efficiency of gas exchange and overall body functioning.
Spirometry Techniques
Spirometry is one of the most common techniques for measuring ventilation. This method assesses how much air a person can inhale and exhale, along with how quickly they can do so. It involves using a spirometer, a device that records the volume and flow of air during breathing.
There are different types of spirometry tests, such as:
- Forced Vital Capacity (FVC): Measures the total amount of air exhaled.
- Forced Expiratory Volume in one second (FEV1): Records the volume of air exhaled in the first second of forced exhalation.
- Peak Expiratory Flow Rate (PEFR): Tests how fast air can be blown out.
These measurements reveal valuable information about lung function. They can help identify conditions like asthma, chronic obstructive pulmonary disease (COPD), and restrictive lung diseases. The results can indicate if the airways are obstructed and if lung capacity is compromised.
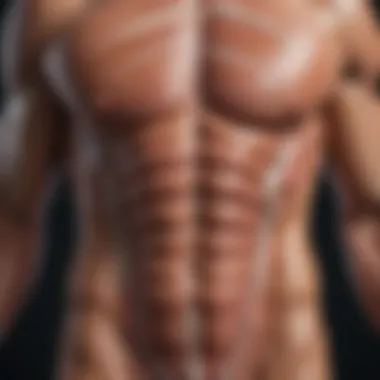
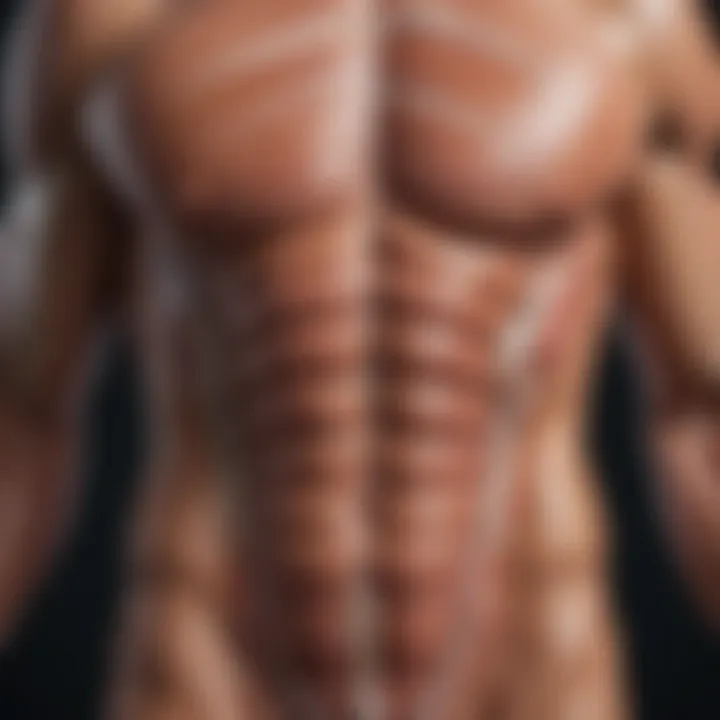
Normal Values and Interpretation
Interpreting the results from spirometry requires knowledge of normal values, which are determined based on age, gender, height, and ethnicity. A fundamental understanding of these normal values is paramount for accurate assessments.
Key spirometry values include:
- FVC: A normal range indicates good lung capacity.
- FEV1/FVC Ratio: This ratio helps distinguish between obstructive and restrictive lung disease. A lower ratio often suggests an obstructive problem.
- PEFR: Shows how effectively a person can exhale air.
Healthcare professionals analyze these results in conjunction with clinical symptoms. Deviations from normal values can signal various health issues. Close attention must be given to any parameters outside the expected range, as they guide further investigation or intervention.
Accurate measurement and interpretation of ventilation are vital for effective disease management and improving patient care systems. Proper knowledge ensures a higher quality of life for affected individuals.
Ventilation Disorders
Ventilation disorders represent a critical aspect of respiratory health, directly impacting the efficiency and effectiveness of gas exchange in the lungs. Understanding these disorders is essential, as they can significantly alter an individual's quality of life and may lead to serious health complications if left untreated. By examining various conditions, we can elucidate the mechanisms that underlie ventilation disorders and identify strategies for management and treatment.
Chronic Obstructive Pulmonary Disease (COPD)
Chronic Obstructive Pulmonary Disease (COPD) is a progressive lung disease characterized by airflow obstruction and difficulty in breathing. COPD includes chronic bronchitis and emphysema, both of which contribute to reduced lung ventilation. Several factors can lead to COPD, with tobacco smoke being the primary cause.
In individuals with COPD, the airflow limitation is not fully reversible and tends to worsen over time. Symptoms often include persistent cough, excessive sputum production, and shortness of breath. The disease can drastically reduce exercise capacity, affecting daily living activities.
Management of COPD involves a combination of lifestyle changes, medication, and pulmonary rehabilitation. Common treatment options include:
- Bronchodilators: These help to relax and open the airways.
- Corticosteroids: These reduce inflammation and help manage symptoms.
- Oxygen therapy: This supports those with severe COPD who struggle to maintain adequate oxygen saturation.
Reducing exposure to irritants and implementing effective management strategies can lead to improved quality of life for patients with COPD.
Asthma and Related Conditions
Asthma is a chronic inflammatory disorder of the airways, leading to recurrent episodes of wheezing, breathlessness, chest tightness, and coughing. This condition is often triggered by allergens, irritants, and respiratory infections, which can aggravate airway hyperresponsiveness.
In asthmatic patients, airways become narrowed due to inflammation and bronchoconstriction, causing difficulty with ventilation. The severity of asthma can vary greatly among individuals, with symptoms ranging from mild to life-threatening.
Management and control strategies for asthma include:
- Inhaled corticosteroids: These are the most effective long-term control medications, reducing inflammation.
- Short-acting beta-agonists: Also known as rescue inhalers, these provide quick relief of asthma symptoms.
- Avoidance of triggers: Identifying and avoiding allergens or irritants can significantly reduce the frequency of asthma attacks.
Monitoring asthma symptoms and maintaining a personalized action plan is vital in preventing severe exacerbations.
Restrictive Lung Diseases
Restrictive lung diseases encompass a broad spectrum of conditions that impair lung expansion, leading to decreased lung volume and, consequently, impaired ventilation. These conditions can be classified into intrinsic and extrinsic categories. Intrinsic restrictive lung disease is often due to lung tissue itself being affected, while extrinsic factors may include chest wall deformities or neuromuscular disorders.
Common intrinsic restrictive diseases include pulmonary fibrosis and sarcoidosis. Symptoms often include:
- Shortness of breath during exertion.
- A persistent dry cough.
- Fatigue or weakness.
Key interventions for restrictive lung diseases may involve:
- Pulmonary rehabilitation: This program helps improve lung function and quality of life.
- Medications: Corticosteroids or antifibrotic drugs can help manage symptoms and slow disease progression.
- Oxygen therapy: This assists patients who are experiencing low blood oxygen levels.
The management of restrictive lung diseases requires a comprehensive approach tailored to individual needs, emphasizing early detection and intervention.
Ventilation and Exercise
Ventilation and exercise is a vital topic regarding the efficiency of the respiratory system. As physical activity increases, the body demands more oxygen. This demand requires adjustments in ventilation to ensure that gas exchange remains optimal. Understanding how ventilation changes during various forms of exercise can enhance athletic performance and inform recovery strategies in clinical settings.
Changes in Ventilation During Physical Activity
During exercise, several physiological mechanisms alter ventilation rates to meet the body's increased need for oxygen. Initially, breathing frequency increases, allowing more air to enter the lungs. This increase starts even before physical activity begins, a phenomenon known as the anticipatory rise.
As exercise progresses, tidal volume, the amount of air inhaled or exhaled in one breath, also increases. Together, these changes raise minute ventilation, which is the total volume of air breathed in one minute. Essential factors that impact these changes include:
- Intensity of Exercise: Higher intensity leads to a disproportionate rise in ventilation.
- Type of Activity: Endurance activities, like running, often lead to different ventilatory patterns compared to high-intensity interval training.
- Fitness Levels: Fitter individuals may show more efficient ventilatory responses.
These adjustments ensure that muscles receive adequate oxygen and can expel carbon dioxide effectively. However, inefficient ventilation can lead to fatigue and decreased performance.
Ventilatory Threshold and Endurance
Ventilatory threshold refers to the point during exercise where ventilation begins to rise at a faster rate than oxygen uptake. Recognizing this threshold is crucial for athletes and individuals aiming to improve their endurance.
- Mechanisms Behind Ventilatory Threshold: The body’s metabolic demands during vigorous activity shift, moving from primarily aerobic metabolism to anaerobic metabolism. This leads to an accumulation of carbon dioxide and lactic acid in the blood. The need to expel these waste products thus elevates the rate of ventilation significantly.
- Training Implications: Understanding and identifying an individual’s ventilatory threshold can aid in the development of tailored training programs. Training just below this threshold can enhance endurance capacity and delay the onset of fatigue.
- **Testing: ** Athletes often undergo specific tests to identify their ventilatory threshold, thus customizing their training regimens appropriately.
Overall, the interplay between ventilation and exercise highlights the importance of a well-functioning respiratory system in maintaining athletic performance and overall health. Efficient ventilation ensures that physical activity can be conducted at optimal levels, supporting both immediate performance and long-term aerobic capacity.
Optimal ventilation is essential not only for athletic performance but also for overall health and wellness, particularly in populations that may experience respiratory issues.
By studying these interactions, students, researchers, and practitioners can develop a more in-depth understanding of human physiology in both health and disease.
Influence of Altitude on Ventilation
Understanding the influence of altitude on ventilation is crucial for comprehending how the human respiratory system adapts to varying environmental conditions. At higher altitudes, the decrease in atmospheric pressure leads to reduced oxygen availability, which can significantly impact breathing patterns and gas exchange efficiency. This section will explore how hypoxia affects the body and the acclimatization processes that occur in response to these challenges.
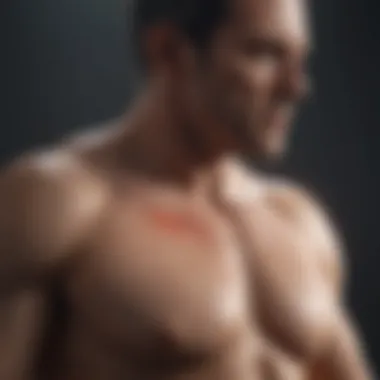
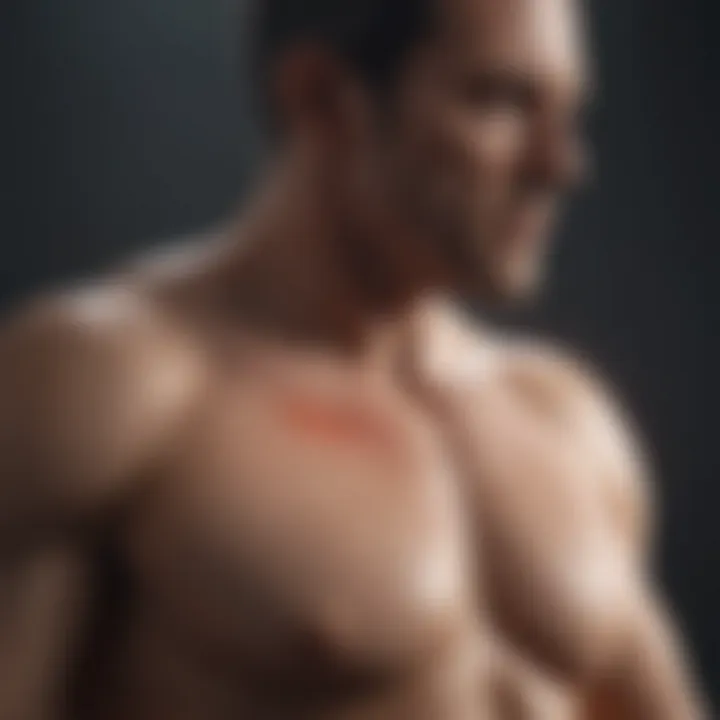
Hypoxia and its Effects
Hypoxia refers to a state where the body or a region of the body is deprived of adequate oxygen. It is a prevalent condition at high altitudes due to the lower partial pressure of oxygen in the atmosphere. The body responds to hypoxia in several ways, some of which are immediate while others take time to develop.
One immediate response to hypoxia is an increase in respiratory rate. The body attempts to intake more oxygen by breathing faster and deeper. This adaptation, however, may not be sufficient in extremely high altitudes, which can lead to a condition known as altitude sickness.
"Altitude sickness can manifest as headaches, nausea, and fatigue, and can range from mild to severe, requiring prompt descents in serious cases."
Additionally, prolonged exposure to hypoxia triggers physiological changes. The body starts producing more red blood cells to enhance oxygen-carrying capacity. This process takes days to weeks, illustrating the body’s remarkable ability to adapt to challenging conditions.
Acclimatization Processes
Acclimatization is the body's long-term adjustment to reduced oxygen levels associated with high altitudes. Various adaptive mechanisms come into play to maintain adequate oxygen delivery to tissues.
Some key acclimatization processes include:
- Increased Breathing Rate: As mentioned, the initial response to altitude is hyperventilation. Over time, the body lowers the hyperventilation response while maintaining adequate oxygen saturation.
- Erythropoiesis: There is a significant increase in the production of erythropoietin (EPO), which stimulates the production of red blood cells in the bone marrow. More red blood cells lead to better oxygen transport throughout the body.
- Metabolic Adjustments: The body may also shift its metabolism from using predominantly aerobic pathways to anaerobic pathways during high-altitude exertion, allowing for energy production despite lower oxygen availability.
These acclimatization processes not only mitigate the effects of hypoxia but also enhance individual performance at high altitudes. Athletes, for example, often train at altitude to take advantage of the increased red blood cell count that enhances endurance capacity when they return to lower elevations.
Understanding these adaptations is vital for professionals working in sports medicine and high-altitude environments. Awareness of hypoxia and acclimatization can guide training routines and safety measures for individuals exposed to high altitudes, whether in recreational settings or competitive sports.
The Role of Ventilation in Homeostasis
Ventilation is not merely a mechanical act, but a vital process that plays a crucial role in maintaining homeostasis within the body. Homeostasis refers to the stability and balance of physiological systems, and efficient ventilation directly influences this balance through various mechanisms. Understanding the role of ventilation helps illuminate its impact on acid-base balance and metabolic processes.
Maintaining Acid-Base Balance
The body's pH levels are tightly regulated. A normal pH range is between 7.35 and 7.45, and even slight deviations can lead to significant health issues. Ventilation assists in regulating carbon dioxide (CO2) levels in the blood, which, in turn, affects the acid-base balance. When ventilation is efficient, CO2 is expelled adequately, preventing its buildup.
Decreased ventilation can lead to hypercapnia, a condition characterized by high CO2 levels. This condition may result in a decrease in blood pH, leading to acidosis. Conversely, hyperventilation may cause a rapid decrease in CO2, leading to alkalosis. Thus, the respiratory center of the brain adjusts breathing rates based on CO2 concentrations, illustrating the link between ventilation, gas exchange, and acid-base homeostasis.
Impact on Metabolism
Ventilation also has a profound effect on metabolic functions. The process of respiration ensures that oxygen is available for metabolic activities in cells. Oxygen is needed to convert nutrients into energy through aerobic metabolism. Inadequate ventilation reduces oxygen supply, which can cause inadequate energy production.
Moreover, during periods of high metabolic demand, such as exercise, the body requires more oxygen. This demand results in increased ventilation to ensure that oxygen intake meets metabolic needs. Additionally, a proper ventilatory function allows for the removal of metabolic waste products, like CO2, maintaining not just energy levels, but also functioning of vital organs.
Understanding the importance of sufficient ventilation is critical in both health and disease management.
Utilizing this knowledge can lead to advancements in medical treatments and strategies for improving respiratory health.
Clinical Implications of Ventilation
Understanding ventilation goes beyond basic physiology. It has crucial clinical implications, influencing how healthcare providers approach various respiratory conditions. Efficient ventilation is essential for maintaining adequate gas exchange, necessary for oxygen delivery and carbon dioxide removal. Disruptions in ventilation can lead to significant health issues, underscoring the importance of accurate assessment and management in clinical settings.
Assessment of Ventilation in Clinical Settings
In clinical practice, assessment of ventilation plays a critical role in diagnosing and managing respiratory disorders. Various methods are utilized to evaluate how well a patient is ventilating, including:
- Spirometry: This common test measures the volume of air inhaled and exhaled. It provides insight into lung function, helping to identify obstructive or restrictive patterns. Normal spirometry results guide clinicians in determining further steps for patient care.
- Blood Gas Analysis: Arterial blood gases are essential for understanding the efficiency of ventilation at a metabolic level. It reveals oxygen and carbon dioxide levels in the blood, indicating how well the lungs perform gas exchange.
- Peak Flow Measurement: This assessment is often used in asthma management. It quantifies how quickly air can be expelled from the lungs, helping to monitor airway function over time.
Accurate assessment of ventilation allows healthcare professionals to tailor therapies effectively. Early identification of problems can lead to better outcomes and prompt interventions.
Treatment Approaches for Ventilation Disorders
Ventilation disorders present a variety of challenges for healthcare providers. Treatment strategies need to be individualized, often involving a combination of medical and sometimes surgical interventions. Key treatment approaches include:
- Bronchodilators: These medications relax the muscles surrounding the airways. They are essential in managing conditions like Chronic Obstructive Pulmonary Disease (COPD) and asthma, as they enhance airflow and improve overall ventilation.
- Corticosteroids: Used to reduce inflammation in the airways, corticosteroids help to manage conditions characterized by chronic inflammation. They improve lung function and subsequently enhance ventilation.
- Oxygen Therapy: In cases of severe hypoxia, supplemental oxygen can improve ventilation effectiveness by increasing available oxygen for gas exchange.
- Pulmonary Rehabilitation: This multidisciplinary approach focuses on improving the physical and emotional well-being of patients with lung diseases. It includes exercise training, nutritional advice, and education about disease management.
Additionally, non-invasive ventilation devices may be used for patients with acute respiratory failure. These strategies can significantly improve ventilation and enhance quality of life for individuals with compromised respiratory function.
Overall, understanding clinical implications of ventilation is crucial for timely and effective treatment, leading to improved patient outcomes in various respiratory disorders.
By maintaining a keen focus on the clinical aspects of ventilation, healthcare providers can greatly enhance the care they offer to patients suffering from respiratory issues.
Future Research Directions in Ventilation
The field of lung ventilation remains ripe for exploration and advancement. Future research directions are essential not only for understanding the complexities of respiratory mechanics but also for addressing clinical challenges associated with various ventilation disorders. Focused studies can lead to innovations that enhance both diagnostic and therapeutic approaches, ultimately improving patient outcomes. Several important aspects of future research in ventilation merit attention.
Innovations in Ventilation Measurement
Accurate measurement of ventilation is critical for diagnosing and managing respiratory disorders. Advances in technology can enhance the precision of these measurements. Traditional methods, such as spirometry, have limitations that newer technologies aim to overcome.
Recent innovations include:
- Non-invasive Measurement Techniques: Developments in technologies that allow for easy, accurate, and quick assessments without discomfort to the patient.
- Wearable Devices: The emergence of sensor technology capable of continuously monitoring ventilation parameters in real-time can show promise in both clinical and non-clinical environments. These devices allow tracking of factors like tidal volume and respiratory frequency, emphasizing individual variations.
- Integration of Machine Learning: Utilizing machine learning algorithms can help interpret data from various measurements, predicting fluctuations in ventilation due to various triggers.
These innovations could make it feasible to gain insights into ventilation under different conditions. For example, it can help assess how factors such as exercise or altitude affect ventilation metrics.
Potential Therapies for Ventilation Disorders
Therapeutic strategies for ventilation disorders need continuous research for development. Existing treatment methods often fall short, especially in chronic conditions. New therapies could enhance lung function and improve the quality of life for many patients.
Some promising research areas include:
- Gene Therapy: Investigating gene therapy techniques can offer insights into correcting specific genetic defects linked to certain ventilatory disorders.
- Regenerative Medicine: The usage of stem cells has potential for repairing lung tissue damaged by diseases like COPD or asthma. Early research is promising but needs further exploration.
- Targeted Pharmacological Treatments: Developing medications that better target respiratory pathways to reduce inflammation or dilate airways may lead to more effective treatments with fewer side effects.
"Investing in future research directions will enhance our understanding and capability to treat ventilation-related disorders efficiently and effectively."
In sum, pursuing these areas compellingly illustrates the importance of research in ventilation. New discoveries may lead to significant improvements in understanding, measuring, and treating disorders that affect millions worldwide.