Understanding the Mechanisms of Lithium-Ion Batteries
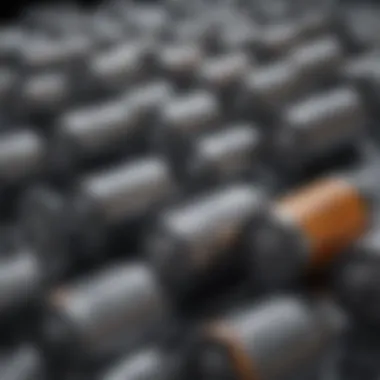
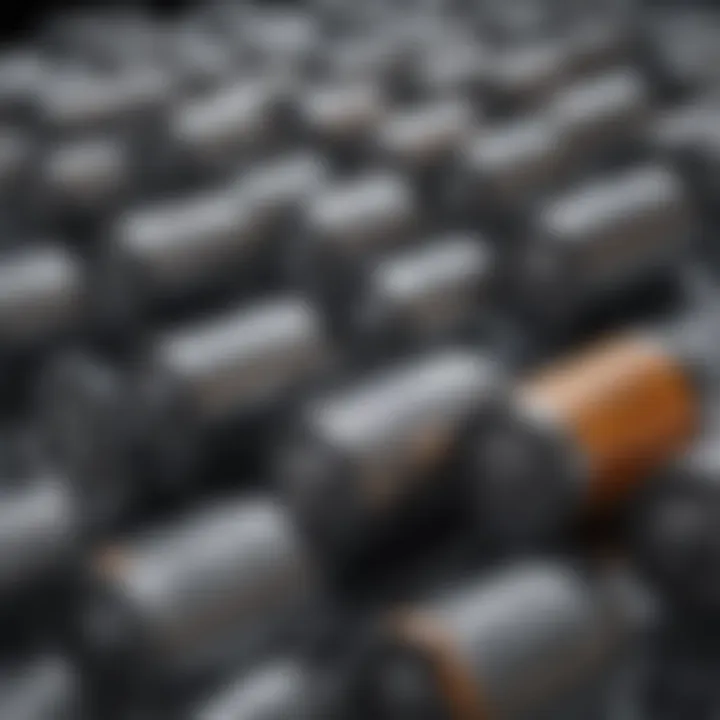
Intro
Lithium-ion batteries have transformed the landscape of portable energy storage. They power a range of devices from smartphones to electric vehicles. Understanding the mechanisms behind these batteries is crucial because it illuminates their efficiency, longevity, and potential for future applications.
The journey into the science of lithium-ion batteries provides insights into their construction and functioning. The article aims to unpack complex topics and present them in an approachable way. By doing this, we strive to cater to students, educators, and professionals who seek a deeper grasp of these vital energy sources.
Methodology
Study Design
This exploration into lithium-ion batteries is based on a comprehensive literature review. Key resources include scientific studies, university publications, and industry reports. This method allows us to assess various components and reactions occurring within lithium-ion batteries.
Data Collection Techniques
Our data collection encompasses information on battery materials, electrochemical behavior, and historical developments. Sources have been selected to provide a well-rounded perspective on how lithium-ion technology has evolved.
Discussion
Interpretation of Results
The review illustrates that lithium-ion technology is built on a few fundamental principles. First, the battery consists of an anode, a cathode, and an electrolyte. Typically, the anode is made from graphite, while the cathode often consists of lithium cobalt oxide or lithium iron phosphate. When the battery charges, lithium ions move from the cathode through the electrolyte to the anode. During discharge, this movement is reversed. This simple yet effective process underpins the functionality of lithium-ion batteries.
"Understanding these core mechanisms is essential for optimizing battery designs and improving energy storage."
Another significant point is the role of chemical reactions in energy transfer. The specific materials used can influence the battery's performance, lifespan, and safety.
Limitations of the Study
While the methodology employed provides substantial insights, there are limitations. The primary concern is that the information may become outdated as technology advances rapidly. New research might introduce alternative materials or methods unaccounted for in this review.
Future Research Directions
Future advancements in lithium-ion technology promise exciting possibilities. Research is ongoing into solid-state batteries, which could improve safety and energy density. Additionally, an emphasis on sustainable practices, including recycling and reducing cobalt usage, is becoming more pertinent.
In summary, understanding lithium-ion batteries requires a multi-faceted approach. As technology continues to evolve, so too will the methods and materials used in battery development.
Preface to Lithium-Ion Batteries
Lithium-ion batteries have transformed the way we store and use energy, propelling advancements in various sectors, including consumer electronics and electric vehicles. Understanding the mechanisms behind these batteries is crucial for anyone involved in fields related to technology, engineering, or environmental sustainability. Their increasing utilization underlines the importance of comprehending how they function and their potential impact on society.
This section will delve into the fundamental aspects of lithium-ion batteries. It aims to provide a clear definition and overview, along with a historical perspective to contextualize their development. By examining these elements, readers can grasp not just how lithium-ion batteries work but also why they are significant today.
Definition and Overview
Lithium-ion batteries are rechargeable batteries that use lithium ions as the key component of their electrochemistry. These batteries consist of a positive electrode, known as the cathode, and a negative electrode, referred to as the anode. During operation, lithium ions move between the electrodes through an electrolyte, enabling the storage and release of energy. Common cathode materials include lithium cobalt oxide, while graphite is a typical material for the anode.
One of the main advantages of lithium-ion technology is its high energy density, which allows for more energy to be stored in a smaller volume compared to other battery types. This makes them a preferred choice for portable devices like smartphones and laptops, as well as for larger applications such as electric vehicles.
Historical Development
The evolution of lithium-ion batteries dates back to the 1970s. Initially, researchers experimented with various chemistries, exploring different materials for electrodes and electrolytes. The first commercial lithium-ion battery was introduced by Sony in 1991, marking a significant milestone in battery technology. This battery was revolutionary, as it offered a viable solution to the limitations of earlier rechargeable batteries, like nickel-cadmium and nickel-metal hydride batteries.
Since then, research has progressed rapidly, driven by the demand for longer-lasting and more efficient energy storage solutions. Areas that have seen notable innovation include improved safety features and advanced charging technology. As our reliance on battery-powered devices continues to grow, understanding the historical context of lithium-ion batteries helps us appreciate the advancements that have shaped this essential technology.
Basic Components
The basic components of lithium-ion batteries are critical to understanding their overall functionality and efficiency. Each part plays a specific role that contributes to the energy storage processes, reliability, and performance of the battery. Analyzing these components helps in appreciating how they work together to enable the diverse applications we see today, from powering smartphones to enabling electric vehicles.
Cathode Materials
The cathode material is pivotal. Most lithium-ion batteries use cathodes made from lithium cobalt oxide, lithium iron phosphate, or lithium manganese oxide. The choice of cathode material impacts battery capacity, voltage, and safety. For example, lithium cobalt oxide is known for its high energy density but is less stable thermally compared to lithium iron phosphate.
**Considerations:
- Energy Density:** Higher energy density means more energy stored for a given weight.
- Thermal Stability: Ensuring the battery does not face overheating risks.
- Cycle Life: Some materials degrade faster than others, affecting overall lifespan.
Anode Materials
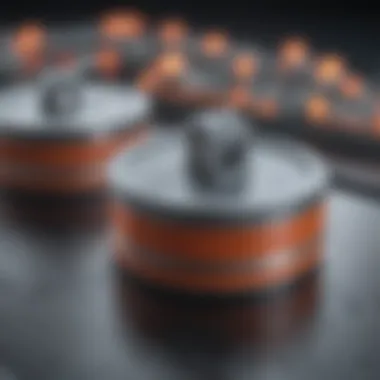
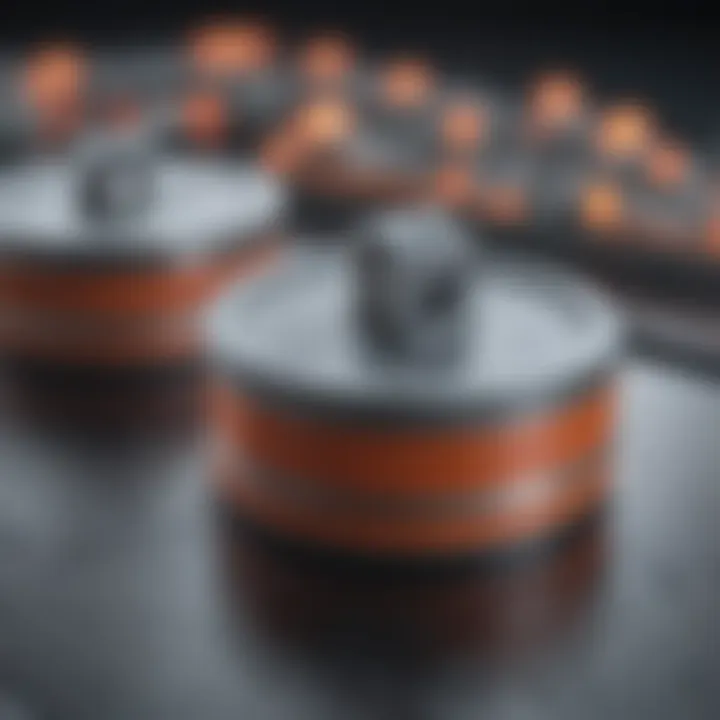
The anode in a lithium-ion battery typically consists of graphite or silicon. Graphite is the most common choice due to its proven performance and availability. However, silicon offers significantly higher capacity. Challenges arise as silicon expands and contracts during charge and discharge cycles, which can lead to mechanical stress and reduced lifespan.
**Key Aspects:
- Capacity Improvement:** Silicon could potentially increase capacity but needs innovative solutions to manage its physical changes.
- Cost Efficiency: Material cost can dictate anode choice for large-scale applications.
Electrolyte Composition
The electrolyte facilitates the movement of lithium ions between the anode and cathode. Liquid electrolytes are commonly made from lithium salts dissolved in organic solvents. Solid electrolytes are emerging as a safer, more stable option but come with their own set of challenges. The composition directly influences battery performance, safety, and longevity.
**Important Factors:
- Ionic Conductivity:** Higher conductivity allows for faster charge and discharge.
- Stability: The electrolyte must withstand various operating temperatures without breaking down.
- Safety: Non-flammable electrolytes help mitigate fire risks.
Separator Functions
The separator is a crucial component that prevents the anode and cathode from contacting each other while allowing lithium ions to flow freely. Typically made from porous polyethylene or polypropylene, the separator must ensure mechanical integrity while maintaining high ionic conductivity. Its effectiveness is essential for preventing short circuits, which could lead to failure or dangerous incidents.
**Key Functions:
- Safety:** Prevents internal short circuits, enhancing overall battery safety.
- Ionic Conductivity: Good separators maximize ion flow while blocking electrons.
In summary, the basic components of lithium-ion batteries—cathode, anode, electrolyte, and separator—work in concert. The choice of materials and designs significantly affects performance characteristics, safety, and longevity. A deep understanding of these components lays the foundation for further exploration into battery mechanisms and innovations.
Operational Mechanism
The operational mechanism of lithium-ion batteries is fundamental for understanding their functionality and the role they play in contemporary technology. This section provides critical insights into the charge and discharge cycles that define the operation of these batteries. It elaborates on how energy is stored, utilized, and managed within the system. By grasping these mechanisms, one can comprehend the efficiency, longevity, and overall behavior of lithium-ion batteries. This knowledge is essential for students, researchers, and professionals who work with or study energy storage solutions.
Charge Cycle Overview
During the charge cycle, lithium ions move from the cathode to the anode through the electrolyte. The process starts when an external power source, like a charger, applies a voltage to the battery. This voltage forces electrons to flow through the external circuit. As electrons travel towards the anode, lithium ions migrate toward the anode through the electrolyte and the separator. This movement causes the anode material, typically graphite, to absorb the lithium ions, storing energy in the process. The charging efficiency is critical, as it determines how effectively the battery can convert electrical energy into stored chemical energy. Factors such as temperature, charge voltage, and the nature of the electrolyte can significantly influence this efficiency.
Discharge Cycle Overview
When the battery is in use, the discharge cycle occurs. Here, the process is essentially the opposite of charging. Lithium ions leave the anode and move back to the cathode through the electrolyte. This ion movement is facilitated by the flow of electrons back through the external circuit, generating electric current that powers devices. The discharge process is where the battery releases its stored energy. Key considerations for the discharge cycle include the rate at which energy is consumed and how it affects the battery's life span. High discharge rates can lead to increased wear on the battery, while lower rates tend to prolong its usable life.
Role of Lithium Ions
Lithium ions serve as the cornerstone of the functionality of lithium-ion batteries. Their ability to easily intercalate into anodes and cathodes allows for efficient charge and discharge cycles. Notably, the lightweight nature of lithium contributes to the overall energy density of the battery, making it suitable for various applications. As lithium ions travel between the anode and cathode, they facilitate the flow of electrons which, in turn, powers electrical devices. The specific behavior and movement of these ions under different conditions affect the battery's performance and stability, highlighting their importance in battery design and improvement.
Understanding the mechanisms behind the operational processes of lithium-ion batteries is key to advancing energy storage technologies and improving their sustainability.
Energy Density and Efficiency
Energy density and efficiency are fundamental attributes that directly influence the performance and applicability of lithium-ion batteries. Understanding these concepts is critical for evaluating battery technologies, especially as demand for more efficient energy storage solutions grows. Energy density is primarily defined as the amount of energy that can be stored per unit weight or volume. Higher energy density implies that a battery can store more power without increasing its physical size or weight, a significant factor for portable devices and electric vehicles.
Factors Affecting Energy Density
Several factors impact the energy density of lithium-ion batteries, contributing to their overall performance:
- Material Composition: Different materials used for the cathode and anode play a crucial role. For instance, lithium cobalt oxide provides high energy density but is not as stable or safe as alternatives like lithium iron phosphate, which has lower energy density.
- Electrolyte Properties: The choice of electrolyte impacts both the chemical reactions within the battery and the ions' mobility. A more conductive electrolyte can enhance the battery's ability to store energy efficiently.
- Design Innovations: Advances in battery design, including electrode thickness and porosity, can significantly affect energy density. Thinner electrodes can allow for greater capacity, as they minimize the amount of non-active material.
- Temperature Effects: Energy density can also vary with temperature, influencing the battery's performance in different environments. Operating outside optimal temperature ranges can reduce energy efficiency, leading to potential losses in capacity.
The interplay of these factors underscores the need for ongoing research and development to enhance energy storage technologies for a variety of applications.
Comparison with Other Battery Technologies
When comparing lithium-ion batteries with other battery technologies, several key differences emerge:
- Nickel-Metal Hydride (NiMH): NiMH batteries generally have lower energy capacity than lithium-ion batteries. While they can provide more stable discharge rates, their size and weight often hinder them in portable applications.
- Lead-Acid Batteries: Though lead-acid batteries are still widely used due to their low cost and simplicity, they have significantly lower energy density than lithium-ion batteries. This makes them less suitable for modern applications where space and weight are critical.
- Solid-State Batteries: A promising alternative, solid-state batteries offer potential for higher energy density and improved safety. While still largely in the experimental phase, they are regarded as the next frontier for energy storage.
"The efficiency of energy storage technologies determines their viability in a world increasingly reliant on portable power and renewables."
Further exploration and innovation are vital to maintaining the competitive edge of lithium-ion technology in an evolving energy landscape.
Challenges and Limitations
Understanding the challenges and limitations of lithium-ion batteries is crucial for anyone involved in their development and application. These issues affect performance, lifespan, safety, and environmental impact. As such, addressing these challenges is vital for the continued advancement of lithium-ion technology. This section highlights key degradation mechanisms and safety concerns that accompany lithium-ion batteries.
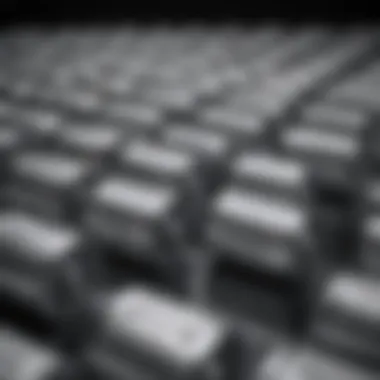
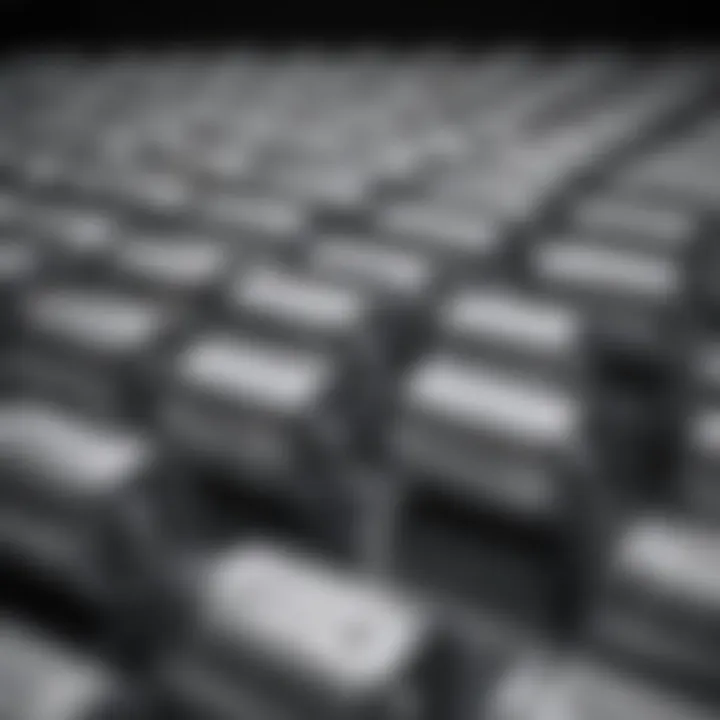
Degradation Mechanisms
Lithium-ion batteries are subjected to various degradation mechanisms that can negatively impact their performance over time. One of the primary reasons for degradation is electrode material wear. When charging and discharging, the electrode materials can experience structural changes. These changes may lead to a decrease in conductive pathways, ultimately limiting the efficiency of the battery.
Another significant factor is solid electrolyte interphase (SEI) growth. The SEI layer forms on the anode during the charging cycles. While this layer is essential for lithium ion transfer, excessive growth can hinder performance. The thicker the SEI layer becomes, the more lithium ions are lost to irreversibility, which can shorten battery life.
Additionally, thermal degradation can significantly influence battery efficiency. High temperatures can accelerate all degradation mechanisms. This condition can lead to increased resistance, reduced capacity, and even catastrophic failures in certain situations. Therefore, managing temperature is a critical consideration in lithium-ion battery design.
"Degradation mechanisms are intrinsic challenges that must be navigated to optimize lithium-ion battery performance and longevity."
To mitigate these degradation concerns, researchers are experimenting with advanced materials and electrolyte formulations. Innovations may include the development of silicon-based anodes to enhance capacity or solid-state electrolytes to reduce thermal risks. However, the transition from traditional lithium-ion designs to more advanced versions will require rigorous research and testing.
Safety Concerns
Safety remains a foremost concern in the use of lithium-ion batteries. The potential for overheating, fire, or even explosion can arise from various internal and external factors. The thermal runaway phenomenon is one of the most dangerous risks. It occurs when a battery overheats, causing a chain reaction that leads to further heating and release of flammable gases.
The construction of lithium-ion batteries also contributes to safety issues. Separator failures can lead to direct contact between the cathode and anode, increasing the likelihood of short circuits. These shorts can initiate thermal runaway if not managed properly. Battery management systems are crucial in preventing such events, as they monitor and control charge levels and temperatures.
Furthermore, inadequate formulation of electrolytes and improper manufacturing processes can introduce defects that compromise safety. Establishing stringent manufacturing standards and protocols is essential to minimize these risks. This includes rigorous stress testing and quality assurance methodologies.
While the industry has made progress in enhancing safety features, continued research is needed. Innovative designs, such as safer chemistries and better energy management systems, are essential to mitigate these threats and increase consumer confidence in lithium-ion technology. Safety is not merely a design consideration but a pivotal factor in the evolution of lithium-ion batteries.
Applications of Lithium-Ion Batteries
Lithium-ion batteries have become the backbone of numerous modern technologies. Their lightweight nature combined with high energy density makes them essential in many applications. Understanding where and how these batteries are employed provides insight into their significance in today’s world. The relevance of lithium-ion batteries cannot be understated—whether driving consumer electronics, powering electric vehicles, or enabling energy storage systems, their widespread adoption reflects a shift towards efficiency and sustainability in various sectors.
Consumer Electronics
In the realm of consumer electronics, lithium-ion batteries are ubiquitous. These batteries power smartphones, laptops, tablets, and many other portable devices. Their high energy density allows for longer usage times, essential in a market where consumer demand is for products that last longer without frequent recharging.
- Key benefits include:
- Lightweight: The reduced weight compared to other battery technologies facilitates portability in devices.
- High Efficiency: Lithium-ion batteries exhibit low self-discharge rates, meaning they retain their charge longer when not in use.
- Compact Size: They can be manufactured in various sizes, making them suitable for a wide range of applications.
This critical reliance on lithium-ion technology contributes to innovations in design and performance, enabling manufacturers to push the boundaries of what these devices can achieve.
Electric Vehicles
Electric vehicles (EVs) represent one of the most significant applications of lithium-ion batteries. As the world moves toward greener alternatives to traditional fuel engines, lithium-ion technology is central to this transition. Batteries in EVs need to deliver high power output and energy storage, characteristics that lithium-ion batteries excel at.
- Factors that highlight their importance in EV applications:
- Rapid Charging: Technology advancements have led to faster charging solutions, making EVs more practicable for everyday users.
- Range Capabilities: Modern lithium-ion batteries can now provide drivers with ranges that rival conventional internal combustion engines, increasing consumer acceptance of electric vehicles.
- Performance: The ability to sustain high performance over varied conditions—cold weather, heat, and long travels—undoubtedly enhances the EV appeal.
The shift towards lithium-ion batteries is pivotal for the automotive industry’s efforts in reducing carbon emissions and enhancing overall vehicle efficiency.
Energy Storage Systems
Energy storage systems, particularly for renewable energy sources like solar and wind, are increasingly reliant on lithium-ion battery technology. These systems enable the storing of electrical energy for later use, addressing the intermittent nature of renewable power generation.
- Important aspects include:
- Grid Stabilization: Lithium-ion batteries help smooth out fluctuations in power demand, ensuring a stable supply.
- Scalability: These systems can be scaled up or down depending on the specific needs of a facility or home, making them versatile in various situations.
- Cost-Effectiveness: As production technologies for lithium-ion batteries improve, the cost continues to decline, making energy storage more accessible.
Energy storage solutions not only enhance the efficiency of renewable energy but also play a vital role in achieving a sustainable energy future.
"The integration of lithium-ion technology in consumer electronics, electric vehicles, and energy storage systems represents a significant stride toward create a more sustainable and efficient future."
In summary, the applications of lithium-ion batteries cover a breadth of essential technologies that influence daily life. Their benefits in terms of performance, efficiency, and capacity indicate a vital role in future technological advancements. As research continues, we can expect these rechargeable batteries to evolve further, perpetuating their importance in diverse applications.
Recent Advances in Lithium-Ion Technology
Recent advances in lithium-ion technology represent pivotal developments within the field of energy storage. This section will explore key innovations, notably solid-state batteries and improved charging technologies, highlighting their significance and potential influences on the future of lithium-ion batteries. These advances aim to increase overall performance and safety while enhancing the efficiency of energy storage solutions.
Solid-State Batteries
Solid-state batteries are a promising evolution of traditional lithium-ion batteries. Unlike conventional batteries that use liquid electrolytes, solid-state batteries employ solid electrolytes. This shift can offer several benefits.
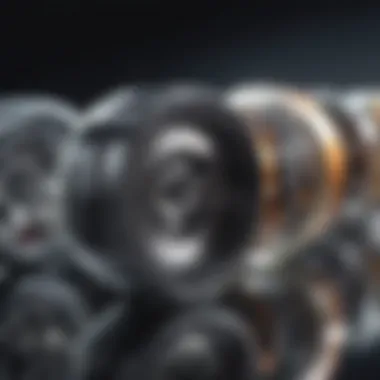
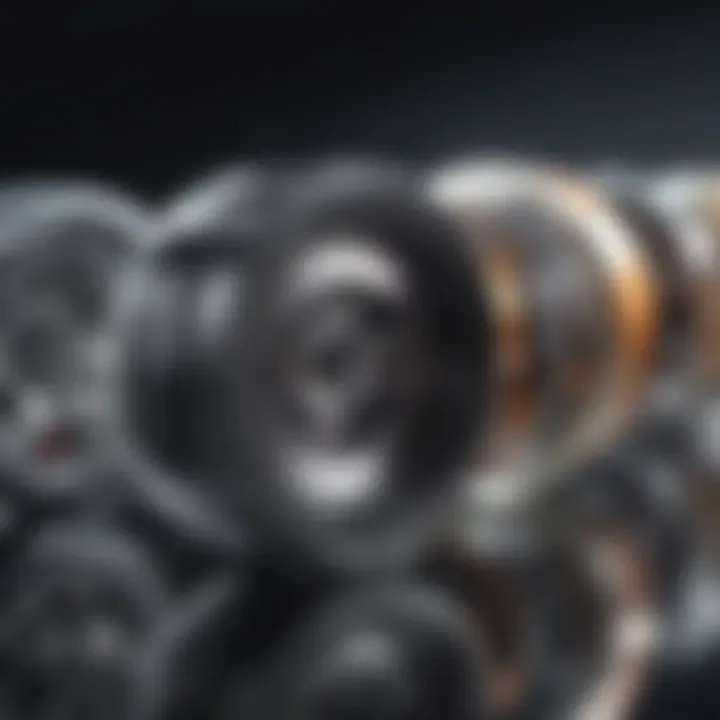
- Safety: Solid-state batteries have a lower probability of catching fire because they do not contain volatile liquids.
- Energy Density: They can achieve higher energy densities, allowing for longer-lasting batteries in smaller packages. This is especially relevant in applications such as electric vehicles.
- Lifecycle: The solid electrolyte can enhance the lifecycle of batteries by minimizing degradation processes.
These features position solid-state batteries as viable alternatives to enhance the safety and performance of energy storage systems, making them a focus of ongoing research.
Improved Charging Technologies
Improved charging technologies are essential for maximizing the utility of lithium-ion batteries. Recent developments in this area have focused on increasing charging speeds without compromising battery life or safety.
- Fast Charging: New charging protocols enable batteries to attain significant charge levels in a fraction of the time compared to earlier models. This is critical for consumer electronics and electric vehicles, where time efficiency is paramount.
- Smart Charging: Integration of intelligent algorithms can optimize charging processes based on environmental conditions and user patterns. This improves efficiency and prolongs battery lifespan.
- Temperature Management: Enhanced systems manage battery temperature, ensuring safe charging even at increased speeds. This can prevent overheating and extend usability.
Environmental Impact
Lithium-ion batteries play a vital role in contemporary energy solutions. Their functionality underpins various technologies, from everyday consumer electronics to electric vehicles. However, the environmental effects of their production, usage, and disposal warrant serious consideration. Understanding these impacts is essential to promote sustainable practices within the battery industry.
Recycling Challenges
Recycling lithium-ion batteries poses significant challenges. Firstly, the processes required to efficiently recover materials are complex. The battery's intricate components, including lithium, cobalt, and nickel, must be carefully extracted. Each material often requires distinct recycling methods, which adds to the technical difficulty.
Moreover, many consumers are unaware of how to properly recycle batteries. This lack of knowledge leads to improper disposal, increasing environmental risks. When batteries are discarded in landfills, they can leak harmful substances into the soil and water systems. This creates potential hazards not only for local ecosystems but also for human health.
Another challenge is the economic aspect of recycling. Current methods can be costly. As a result, some companies may find it more lucrative to mine for new materials instead of recycling existing ones. Thus, improving recycling technology while reducing costs is crucial for a sustainable approach to battery management.
Sustainability Considerations
Sustainability in lithium-ion battery production and use has become a focal point in battery technology discussions. One major element is the sourcing of raw materials. The extraction of lithium, cobalt, and other metals can lead to ecological degradation. Industrial operations often consume vast amounts of water and energy, impacting local communities and natural habitats.
Efforts to increase sustainability include exploring alternative materials. For example, some researchers are investigating the use of sodium-ion batteries, which could reduce reliance on lithium while being less harmful to the environment. This shift may also alleviate pressures on lithium-rich regions.
Additionally, promoting the development of circular economy principles could enhance sustainability. In a circular economy, battery life cycles would be extended through refurbishing and reusing. As a result, products would use fewer raw materials and produce less waste.
"Transitioning to renewable resources and recycling methods is crucial for reducing the environmental impact of lithium-ion batteries."
Future Outlook
The future of lithium-ion batteries is a topic of critical importance in the realm of energy solutions. As society strives for sustainability, efficient energy storage becomes increasingly necessary. Lithium-ion batteries are already pivotal in numerous technologies, from portable electronics to electric vehicles. However, ongoing advancements promise to enhance their efficiency, sustainability, and overall utility.
Trends in Research and Development
Research into lithium-ion technology currently focuses on various trending areas aimed at addressing existing limitations. These include improving energy density, extending battery life, and increasing safety. Specific developments such as the use of silicon in anodes instead of conventional graphite can potentially yield significant gains in capacity. Another riveting avenue is the exploration of solid-state batteries, which utilize a solid electrolyte instead of liquid, showing promise for higher energy densities and improved safety features.
- Silicon Anodes: Researchers are working on silicon-based anodes to replace or supplement graphite. Silicon can theoretically store ten times more lithium per gram compared to graphite.
- Solid-State Batteries: These batteries are being developed to eliminate flammable liquid electrolytes with solid materials. This not only enhances safety but may also allow for greater energy density.
- Fast-Charging Technologies: Research is tackling the efficiency and speed of charging processes, aiming to reduce the time taken to recharge lithium-ion batteries significantly.
Moreover, companies and laboratories are investing in advanced recycling techniques to recover valuable materials from spent batteries. This is crucial as demand for lithium and cobalt continues to rise, and sustainable practices become a global imperative. Improving recycling processes mitigates the environmental impact associated with raw material extraction.
Potential for Alternative Technologies
While lithium-ion technology holds a dominant position today, exploring alternatives is equally vital for a comprehensive energy strategy. Potential alternatives include sodium-ion, lithium-sulfur, and flow batteries. Each of these technologies presents unique advantages and challenges, warranting thorough investigation.
- Sodium-Ion Batteries: With sodium being abundantly available, this technology aims to reduce dependency on lithium. They can work effectively in certain applications, but energy density remains a concern.
- Lithium-Sulfur Batteries: These batteries offer the prospect of significantly higher energy capacities. However, they face challenges related to cycle stability and efficiency that researchers are actively addressing.
- Flow Batteries: Suitable for larger-scale energy storage, they have great potential for renewable energy applications, even though they may not replace lithium-ion batteries in portable electronics.
As the world increasingly shifts towards renewable energy sources, understanding the capacity and viability of these alternative battery technologies is essential. This exploration not only aids in identifying viable substitutes but also fosters innovations that could lead to the next generation of energy storage systems.
"The evolution of battery technology is a vital cornerstone in the advancement of sustainable energy, and understanding future trends is crucial for both industries and consumers."
In summary, the future outlook for lithium-ion batteries encapsulates a blend of enhancing current technology while probing alternative options. Continuous investment in research and development will drive greater efficiency and effectiveness in energy storage, paving the way for more sustainable solutions.
End
The conclusion of this article is pivotal in tying together the extensive examination of lithium-ion batteries. This section highlights not only what has been discussed but also frames the relevance of lithium-ion technology in the wider context of energy solutions. As we move deeper into an era that prioritizes sustainability and efficient energy use, lithium-ion batteries stand out as crucial tools. Their versatility in various applications, from consumer electronics to electric vehicles, underlines their significance in modern technology.
Summary of Key Points
The key points outlined in this article include:
- Definition and Mechanism: Understanding of what lithium-ion batteries are, including their basic components such as cathodes, anodes, electrolytes, and separators.
- Operational Cycles: The detailed processes during charge and discharge cycles that illustrate how energy is stored and released.
- Applications: Insight into various fields where lithium-ion batteries are utilized, emphasizing their role in advancing technology in consumer electronics and renewable energy systems.
- Challenges: Acknowledgment of current limitations and difficulties that the lithium-ion technology faces, including safety concerns and degradation mechanisms.
- Future Prospects: Exploration of recent advancements and potential future innovations that could further enhance the performance and sustainability of lithium-ion batteries.
This summary serves as a foundation for understanding the full impact of lithium-ion technology in daily life and broader energy strategies.
Final Thoughts on Lithium-Ion Batteries
Lithium-ion batteries continue to evolve, reflecting the growing demands for cleaner and more efficient energy solutions. Their capacity for high energy density and efficiency makes them a preferred choice in many sectors. As industries push for better performance and sustainability, ongoing research into chemistry and materials will be critical. Innovations like solid-state batteries and improved charging methods promise not only to address current limitations but also to enhance overall sustainability in energy storage.
The evolution of lithium-ion technology is essential to meet future energy demands while mitigating environmental impacts.