Understanding Geophysics: A Comprehensive Overview
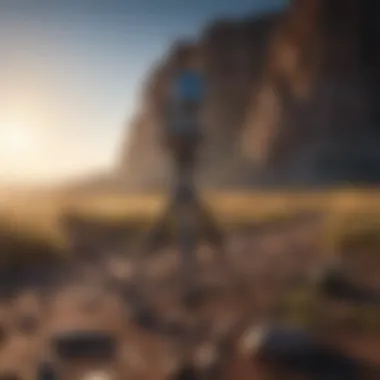
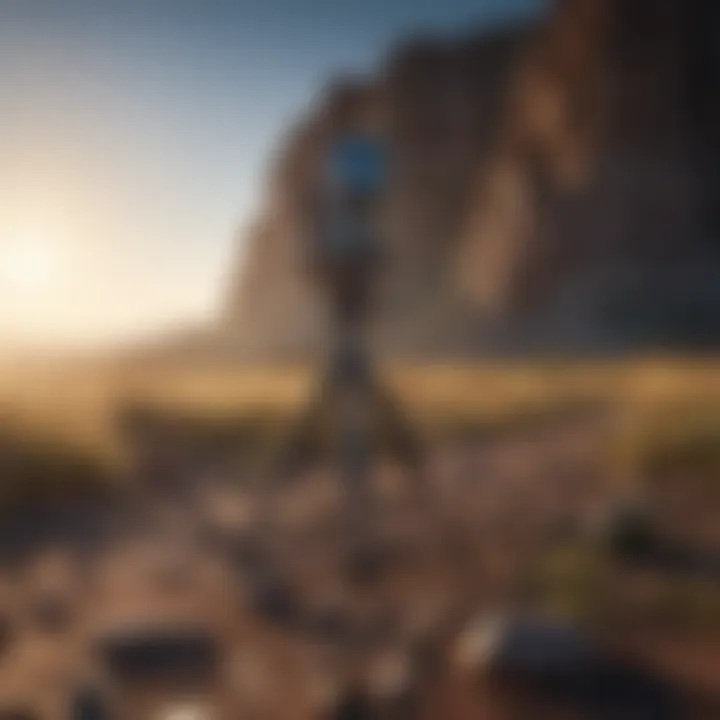
Intro
Geophysics is an expansive field that merges physics, geology, and engineering to study the Earth and its processes. The knowledge gleaned from this discipline supports various scientific and practical applications, including the exploration of natural resources, assessment of environmental settings, and management of natural disasters. Understanding geophysics involves delving into its methodologies, the significance of its findings, and the ongoing research directions that can foster enhancements in our comprehension of the Earth.
This overview serves to bridge gaps in understanding for readers with varied expertise in science, enabling a more accessible exploration of complex geophysical concepts. It discusses key branches of geophysics, methodological approaches, and significant applications. Each section unfolds with relevant details that can enlighten students, researchers, educators, and professionals interested in this vital field.
Methodology
Understanding geophysics requires a structured approach to research and data analysis. The methodology employed in this field encompasses both qualitative and quantitative techniques, enabling geophysicists to obtain comprehensive insights.
Study Design
The design of a geophysical study often includes clearly defined objectives, hypotheses, and methods tailored to specific geological problems. Research can be divided into two primary types:
- Field studies, which involve the collection of data from natural environments.
- Laboratory experiments, which enable controlled analysis of geophysical phenomena.
The choice of study design significantly influences the reliability of results. Each design serves different purposes, allowing researchers to answer varying questions about Earth's structure and processes.
Data Collection Techniques
Data collection in geophysics is diverse, using a variety of instruments and techniques to gather information. Common methods include:
- Seismic surveys, which measure waves traveling through the Earth to unveil subsurface structures.
- Gravitational measurements, which detect variations in mass distribution beneath the Earth's surface.
- Magnetic surveys, which identify changes in the Earth's magnetic field, revealing geological features.
- Electrical resistivity methods, which capture subsurface characteristics based on their resistance to electric currents.
Each technique provides distinct information that researchers can analyze to improve our comprehension of geological settings and processes.
Discussion
The interpretation of results is crucial in geophysics as it connects data to geological phenomena. Insights derived from geophysical studies inform various sectors, including resource management, environmental protection, and disaster preparedness.
Interpretation of Results
Analyzing data demands a rigorous approach. Researchers apply various models and algorithms to interpret results accurately. For instance, seismic data must be processed to differentiate between noise and meaningful signals. Proper interpretation also allows scientists to understand geological features, earthquake risks, and resource availability.
Limitations of the Study
Despite advances in technology and methodology, challenges persist in geophysical research. Limitations may arise from:
- Spatial resolution, which may impact data accuracy.
- Environmental factors that obscure readings.
- Technological constraints that limit measurement capabilities.
These limitations must be acknowledged to contextualize findings and guide future studies.
Future Research Directions
Future advancements in geophysics may focus on:
- Integrating machine learning algorithms to enhance data analysis.
- Developing portable instruments for real-time data collection.
- Collaborative studies that broaden geographic scope and resource sharing.
By advancing methodologies and technologies, the field can become more robust, contributing valuable insights for a variety of applications.
"Geophysics not only enriches our understanding of the Earth but it also plays a pivotal role in addressing pressing global challenges such as climate change and resource management."
In summary, understanding geophysics is integral for various scientific and practical areas, bridging essential knowledge gaps and promoting informed decision-making globally.
Foreword to Geophysics
Geophysics is not merely an academic discipline; it serves as a vital tool in comprehending the very nature of our planet. This section lays the groundwork for understanding its relevance by outlining its significance in various fields such as resource management, environmental studies, and natural disaster mitigation. In a world where resources are becoming scarcer and environmental challenges multiply, geophysics stands at the intersection of natural sciences and practical applications.
Understanding geophysics involves studying the physical processes that govern Earth's internal and external systems. The methods derived from this field provide essential insights into phenomena such as earthquakes, volcanic activity, and climate change, crucial for both academic inquiry and practical problem-solving.
Defining Geophysics
Geophysics combines physics, geology, and mathematics to analyze the planet's structure and its dynamic processes. It primarily focuses on the study of the Earth's physical properties, including gravity, magnetism, and seismic activity. These studies not only enhance our understanding of the planet’s makeup but also aid in the exploration of natural resources like oil, gas, and minerals.
For instance, seismology—a significant branch of geophysics—utilizes seismic waves generated by earthquakes to discern subsurface structures. This knowledge assists in predicting seismic events and assessing areas for potential hazards.
Historical Background
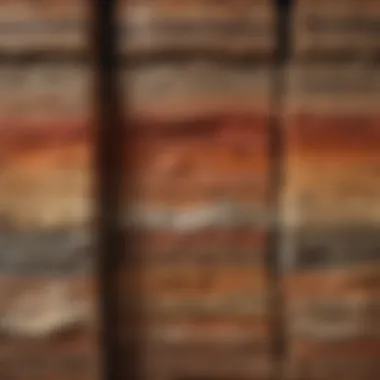
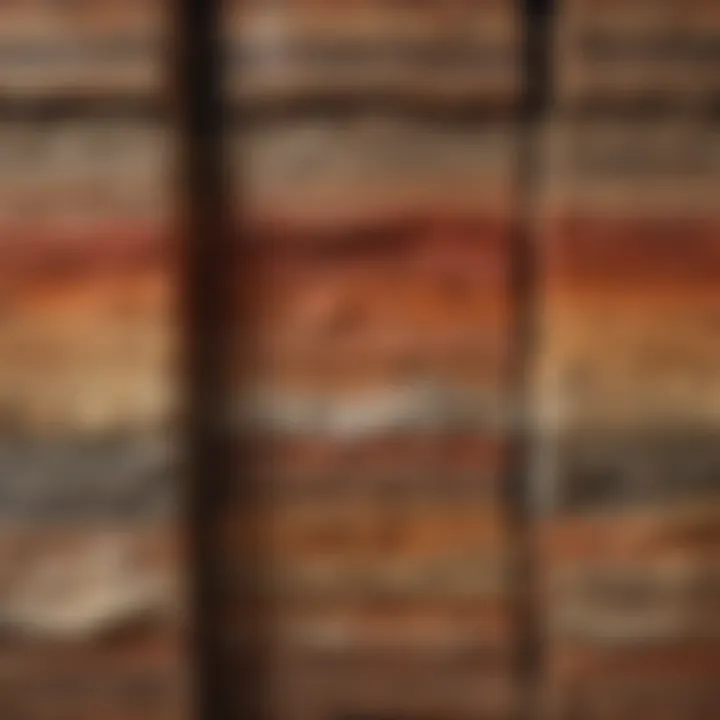
The historical development of geophysics dates back centuries. Early humans attributed natural phenomena to supernatural forces. However, with the advancement of science, there was a shift towards a more systematic exploration of Earth's processes. The 19th century marked a turning point, as pioneers like William Thomson and Henri Becquerel began applying physical principles to study geological phenomena.
The introduction of seismic instruments in the early 20th century was crucial. It allowed scientists to measure seismic waves accurately, leading to breakthroughs in understanding earthquakes. The progression continued through the latter half of the 20th century, with technologies such as satellite imaging and computer simulations revolutionizing geophysical research.
Today, geophysics is instrumental in addressing pressing global issues. With its historical evolution and ongoing advancements, it provides the foundational knowledge necessary for tackling contemporary challenges, particularly in the context of climate change and resource management.
Branches of Geophysics
Understanding the different branches of geophysics is crucial for anyone studying the Earth’s physical processes. These branches not only illuminate various aspects of the planet but also enable professionals to apply specific methods to solve practical problems. From resource exploration to environmental concerns, each branch provides unique insights and tools.
Seismology
Seismology focuses on the study of earthquakes and the propagation of seismic waves through the Earth. By analyzing the characteristics of these waves, seismologists can infer the internal structure of Earth, identify seismic hazards, and provide crucial data for constructing earthquake-resistant buildings. Real-time monitoring systems are essential here, employing instruments such as seismographs. These measurements can help predict seismic activities, thus aiding in disaster preparedness.
Studies in seismology are fundamental not just for hazard assessment but also for understanding plate tectonics, which reveal how continents and ocean floors change over time. The relationship between seismic activity and urban planning cannot be overlooked, as leading to smarter and safer designs in earthquake-prone areas.
Geomagnetism
Geomagnetism is the study of Earth's magnetic field, which varies in intensity and direction. This branch uses instruments like magnetometers to map the geomagnetic field, which assists in navigation and in understanding the geology of regions.
The magnetic field is not constant; it is affected by solar activity and interactions with the Earth’s atmosphere. Understanding these variations has implications for satellite operations, communication systems, and even power grid stability. Geological interpretations derived from geomagnetic studies can reveal ancient volcanic activity and tectonic shifts, offering context for historical geological events.
Gravity Methods
Gravity methods involve measuring the gravitational field of Earth. Changes in this field can indicate variations in subsurface density, which helps in locating natural resources like oil, gas, and minerals. Techniques such as gravimetry enable geophysicists to create detailed maps of underground formations.
These methods are vital for hydrocarbon exploration, as they allow for the identification of potential drilling sites without invasive techniques. Gravity anomalies can also provide insights into tectonic settings and mineral deposits, making this branch essential for resource management and development.
Electromagnetic Methods
Electromagnetic methods use the principles of electromagnetism to investigate subsurface properties. This approach operates through the measurement of the electromagnetic fields emitted by the Earth or induced by external sources. Techniques like ground-penetrating radar (GPR) and frequency-domain electromagnetic surveys are prominent here.
These methods are effective for environmental assessments, revealing contamination levels in soils and aquifers. Industries benefit from electromagnetic surveys by locating valuable resources or understanding the site conditions before construction projects commence.
Hydrogeophysics
Hydrogeophysics combines geophysical methods with hydrology to evaluate groundwater resources. This field applies techniques such as electrical resistivity and seismic methods to map groundwater aquifers and enhance our understanding of water flow and quality.
Proper management of water resources is essential for sustainability. Information from hydrogeophysical studies helps in identifying contamination sources and predicting groundwater behavior in response to environmental changes. This branch is indispensable in areas facing water shortages or pollution.
Near-Surface Geophysics
Near-surface geophysics focuses on the upper layers of the Earth, typically the top few hundred meters. This branch applies various geophysical techniques to investigate shallow geological features, which is crucial for urban development, archaeological studies, and environmental assessments.
Methods such as resistivity and seismic reflection are commonly employed. By understanding subsurface conditions, professionals can mitigate risks in construction and manage resources effectively. Near-surface studies also play a role in detecting landfills and evaluating their potential impact on surrounding areas.
Each branch of geophysics offers unique tools and perspectives. Understanding these variations helps students, researchers, and professionals navigate the complexities of the Earth's processes and apply their findings to real-world applications.
Geophysical Techniques and Instruments
Geophysical techniques and instruments form the backbone of geophysical studies. They facilitate the collection, analysis, and interpretation of subsurface data. With advances in technology, the methods have become more sophisticated, allowing for higher accuracy and better insights into the Earth’s structure. This section dives into the specific techniques and instruments that are pivotal for geophysical research.
Field Surveys
Field surveys are among the primary methods used in geophysics. They involve direct, field-based measurements across specific geographic locations. The objectives can range widely, such as identifying natural resources, understanding geological formations, or assessing environmental conditions.
Common techniques during field surveys include seismic refraction, resistivity surveys, and ground-penetrating radar. Each technique has its advantages. For example, seismic refraction is useful for mapping subsurface layers based on their sonic velocities. Ground-penetrating radar can reveal details about the shallow subsurface, including the presence of hazards or archaeological features.
In conducting a field survey, careful planning is essential, including choosing appropriate sites and methods based on the specific goals of the study. Field surveys not only provide vital data but also add to the understanding of localized geological conditions.
Geophysical Imaging
Geophysical imaging transforms raw data into visual representations of the Earth’s subsurface. This helps researchers visualize complex geological structures and make informed decisions. Imaging techniques can include seismic imaging, electrical resistivity imaging, and magnetic imaging.
In seismic imaging, waves generated by controlled sources are recorded by sensors on the surface. Data processing algorithms convert these signals into images that display subsurface features.
Electrical resistivity imaging involves measuring the resistance of subsurface materials to electric currents. This helps identify variations in soil and rock properties. Magnetic imaging can uncover variations in the Earth's magnetic field, revealing the presence of different geological materials.
These imaging techniques are crucial for applications in natural resource exploration, site assessments, and environmental monitoring. They provide a clearer picture of the subsurface, allowing for more strategic planning and implementation of projects.
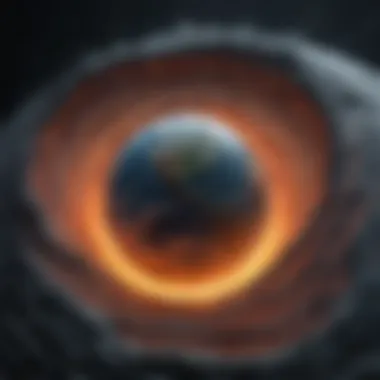
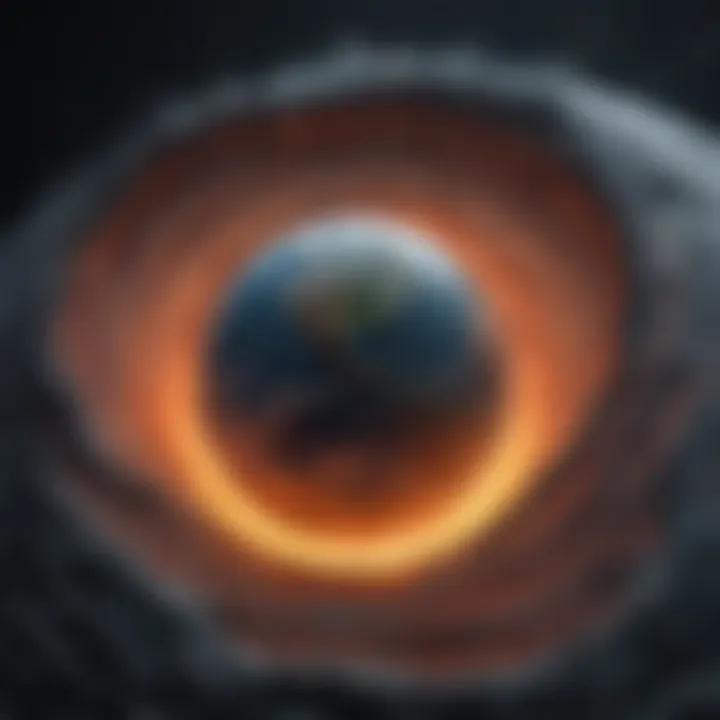
Data Acquisition and Processing
Data acquisition and processing are critical steps in geophysical research. This stage involves collecting raw data from various techniques and converting it into usable information. The collection process must be meticulous to ensure high-quality data that can be reliably interpreted.
Once data is collected, processing methods come into play. Signal processing techniques, filtering, and inversion algorithms are used to refine the data. Signals may contain noise, which needs to be mitigated for accurate interpretation. Moreover, advanced computational methods are increasingly used to handle the large datasets typical in geophysical studies.
The integration of geographic information systems (GIS) with geophysical data also enhances mapping and analysis. This allows for a comprehensive assessment of the data within a broader context, revealing spatial relationships that might otherwise be overlooked.
Overall, successful data acquisition and processing lay the foundation for any meaningful geophysical analysis. They ensure that the findings are based on robust data, leading to accurate conclusions and applications.
"Data processing and imaging are not just techniques; they are essential elements that enable geophysicists to make sense of the complexities beneath the Earth's surface."
In summary, geophysical techniques and instruments are integral to the field of geophysics. The careful application of field surveys, effective imaging practices, and robust data acquisition and processing methods lead to substantial insights into the Earth's subsurface. These elements together advance our understanding of complex geological phenomena, ultimately aiding in decision-making across various scientific and practical realms.
Applications of Geophysics
Geophysics holds a crucial place in numerous fields that extend beyond traditional Earth sciences. Its applications span across resource exploration, environmental monitoring, geotechnical engineering, and natural hazard assessment. Each of these areas reflects the versatility of geophysics in addressing real-world challenges and enhancing our understanding of Earth’s processes. As the need for sustainable practices and effective management of natural resources grows, so does the significance of geophysical applications.
Natural Resource Exploration
Natural resource exploration is one of the most prominent applications of geophysics. This includes oil and gas, minerals, and water resources. Through techniques such as seismic reflection, electromagnetic surveys, and gravity measurements, geophysicists can locate deposits beneath the Earth’s surface without invasive drilling. For example, seismic methods help in mapping subsurface geology to identify potential hydrocarbon reservoirs. This process not only saves costs but minimizes environmental impact, making it essential in today’s resource-hungry world.
Moreover, the identification of groundwater resources through geophysical methods can provide communities with much-needed water supply. The integration of geophysical data with geological models assists in making accurate predictions about where resources might be located.
Environmental Monitoring
The role of geophysics in environmental monitoring cannot be understated. It helps track and assess various environmental factors, including soil contamination, groundwater movement, and seismic activity related to natural disasters. Geophysical instruments enable the detection of pollutants in soils and aquifers, which can lead to timely remediation efforts.
In addition, monitoring subsurface changes over time can provide important data related to climate change, such as land subsidence or increased flooding risks. By analyzing this information, policymakers can develop strategies for sustainable land use and habitat preservation. The proactive nature of these efforts reflects a commitment to environmental stewardship.
Geotechnical Engineering
Geotechnical engineering relies heavily on geophysical techniques to assess the physical and mechanical properties of Earth materials. This application ensures the stability and safety of structures built on or in the ground. By employing methods like ground-penetrating radar and resistivity imaging, engineers can characterize soil layers and identify potential issues such as sinkholes, weak layers, or groundwater flow.
For instance, before constructing a building, geophysicists conduct surveys to analyze the ground conditions. These investigations not only help in designing foundations but also in risk management for construction projects. Therefore, integrating geophysics in engineering practices contributes to safer infrastructures.
Natural Hazard Assessment
Lastly, the assessment of natural hazards is a vital application of geophysics. It involves the study of earthquakes, landslides, tsunamis, and volcanic activity. Seismology, a key branch of geophysics, provides insights into earthquake mechanisms and potential future events.
Geophysical models also help map hazardous areas, allowing for better preparedness and response plans. For example, predictive models can evaluate the likelihood of landslides in certain terrains, aiding in disaster risk reduction strategies. Timely information derived from geophysical assessments can save lives and minimize damage during natural disasters.
"Understanding the applications of geophysics allows us to harness its tools for a sustainable future, enhancing our capacity to manage Earth’s resources responsibly."
In summary, the applications of geophysics are varied and impactful. From resource exploration to environmental health, its techniques provide critical insights that inform decisions across different sectors. As challenges such as climate change and urbanization increase, geophysics will remain a key player in shaping our understanding and managing our environment effectively.
Geophysical Modeling and Simulations
Geophysical modeling and simulations play a crucial role in the field of geophysics. They allow scientists and researchers to understand complex earth processes, predict outcomes, and analyze various scenarios effectively. Modeling can simplify intricate geological structures, making them easier to study and interpret. This section outlines the importance of these practices, touching upon their specifics, benefits, and considerations.
Theoretical Foundations
The theoretical foundations of geophysical modeling are built on established scientific principles. They rely on mathematical frameworks that help describe geological phenomena. For instance, wave propagation, fluid dynamics, and heat transfer theories are commonly applied in modeling. These theories form a basis for understanding how different physical processes interact within the Earth.
Several fundamental equations play a significant role. The wave equation, for example, is vital in seismology. It describes how seismic waves travel through different types of media. Understanding these principles is essential for developing accurate models that can simulate real-world conditions.
Additionally, theoretical foundations inform the assumptions made in simulations. The models can represent certain idealizations of the Earth, thus guiding how scientists approach geological phenomena. It is important for researchers to continually refine their models based on new data or evolving theories.
Numerical Methods
Numerical methods are the computational techniques utilized to solve mathematical models when analytical solutions are challenging or impossible to obtain. In geophysical modeling, these methods are imperative for simulating physical processes at scale. They include methods such as finite difference, finite element, and boundary element techniques, among others.
These methods allow for the approximation of complex systems, enabling researchers to effectively consider large datasets and numerous variables. Numerical methods thus contribute significantly to the accuracy and reliability of geophysical simulations.
The use of computer algorithms also streamlines the modeling process. For instance:
- Finite Difference Methods are often used for time-dependent problems, like seismic wave propagation.
- Finite Element Methods allow for greater flexibility in dealing with irregular geometries, making them suitable for various geological structures.
- Boundary Element Methods help reduce computational load by focusing only on surface interactions.
Through these numerical methods, scientists can generate predictive models that help in understanding subsurface structures or predicting future geological events.
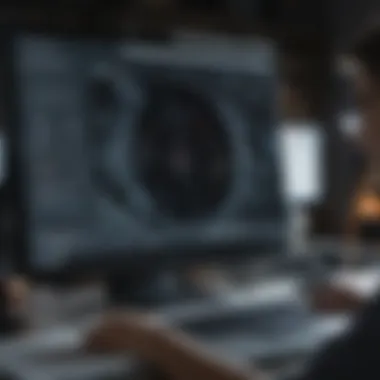
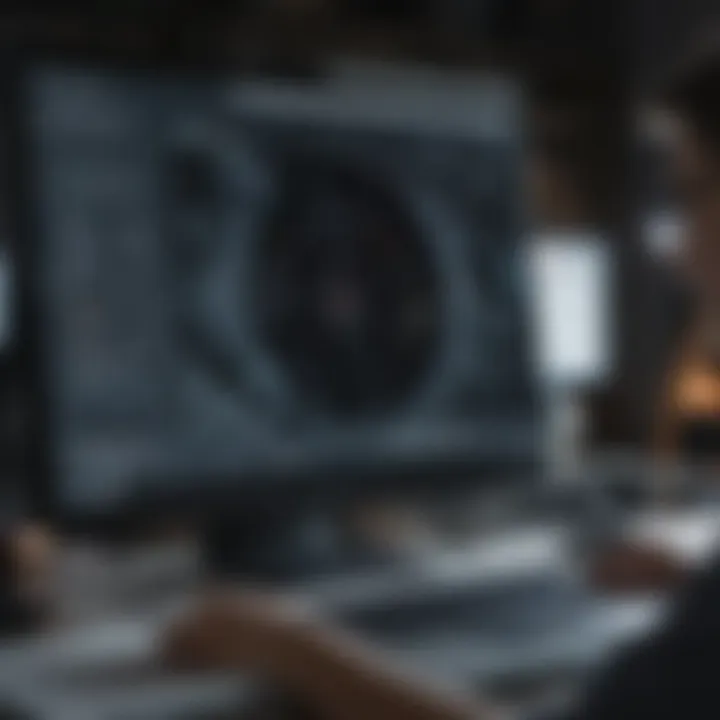
"Understanding the interplay of modeling and real-world data is essential for advancing geophysical research. The techniques and modeling practices developed now will pave the way for future discoveries in Earth sciences."
Challenges and Limitations
The field of geophysics, while rich and expanding, faces several challenges and limitations that can impact both research outcomes and practical applications. Understanding these obstacles is essential for scientists, policymakers, and communities that rely on geophysical data. Addressing these issues not only enhances the quality of geophysical research but also ensures more accurate interpretations and informed decision-making in various applications.
Data Uncertainty
Data uncertainty arises from multiple sources, significantly affecting geophysical investigations. Measurements can be influenced by instrument error, environmental conditions, and the inherent variability in geological materials. For example, seismic wave data can be muddied by noise or reflections from nearby structures, which complicates interpretation.
Factors contributing to data uncertainty include:
- Instrument calibration: Improper calibration can lead to inaccurate readings.
- Sampling strategies: Inadequate or biased sampling can misrepresent geological features.
- Operator error: Skill and experience of personnel can variability be large on data quality.
Mitigating data uncertainty is paramount for producing reliable results. Researchers often employ statistical methods and advanced algorithms to analyze data collaboratively. Additionally, ongoing advances in technology, such as high-resolution sensors and improved data processing techniques, aim to reduce uncertainties and enhance the robustness of geophysical results.
Crucially, acknowledging and quantifying uncertainties helps in making better-informed decisions in resource management and hazard assessment. Without this consideration, misinterpretations can lead to severe consequences in areas like oil exploration or earthquake preparedness.
Interpretative Challenges
Interpretative challenges are an unavoidable aspect of working with geophysical data. The complexity of geological formations often means that multiple interpretations can exist for the same set of data. This multifaceted nature of geophysics requires practitioners to apply judgment and expertise when drawing conclusions.
A few significant challenges include:
- Geological complexity: The Earth's subsurface features are often intricate and not easily understood.
- Model assumptions: Interpretations typically rely on simplifying assumptions that may not hold true in every case.
- Interdisciplinary knowledge: Geophysicists often must blend knowledge from geology, physics, and engineering, which can lead to varying conclusions depending on one's background.
It is essential for geophysicists to remain vigilant about these challenges. Continual dialogue within the scientific community, coupled with advancements in modeling and simulation tools, fosters a better understanding of subsurface processes. Collaboration across disciplines enhances the interpretation, leading to nuanced insights that improve the reliability of conclusions drawn from geophysical data.
"As geophysical techniques evolve, so too must our approach to data interpretation, recognizing the limitations inherent in our methods and embracing collaboration to overcome them."
In summary, the challenges of data uncertainty and interpretative complexities highlight the need for careful analysis and critical thinking in geophysics. Addressing these limitations is crucial for advancing our understanding of the Earth's processes and the practical applications designed to mitigate risks associated with natural resources and hazards.
Future Directions in Geophysics
Geophysics is a dynamic field that constantly evolves in response to technological advances and societal needs. The future direction of geophysics is crucial as it shapes how scientists and researchers will tackle pressing global challenges. This section will explore essential aspects that inform the upcoming trajectories in geophysical research, underscoring the integration of new technologies and collaborative approaches.
Technological Innovations
As we gaze into the future of geophysics, technological innovations stand out as a driving force. Developments in computational power and data processing allow for more complex simulations and models. These enhancements have made it easier to analyze vast datasets collected through various geophysical methods.
- Remote Sensing: The advent of advanced satellite technologies has revolutionized how geophysicists gather data. High-resolution imagery can now monitor geological changes across large areas, from land subsidence to glacier retreat, offering insights that were previously difficult to obtain.
- Sensor Technology: New sensors are being designed for precision and sensitivity. For instance, micro-electromechanical systems (MEMS) are making their way into seismic sensors, allowing for better detection of earth movements.
- Machine Learning and AI: Incorporating algorithmic techniques is enhancing data interpretation. AI can identify patterns in data that humans might overlook, leading to more accurate predictions and assessments in areas such as resource exploration and hazard detection.
"Technological innovations in geophysics not only improve data accuracy but also expand the scope of research possibilities, pushing boundaries of what is achievable in this field."
Interdisciplinary Collaboration
The complexities surrounding geophysical phenomena require a fresh look at interdisciplinary collaborations. Combining expertise across different fields strengthens geophysical research.
- Geology and Environmental Science: Integrating geological knowledge helps contextualize geophysical data, leading to a thorough understanding of the Earth's subsurface. This allows for better predictions regarding natural resources and environmental health.
- Computer Science: The synergy between geophysical research and computer science is essential for processing and analyzing large datasets. As tools evolve, the collaboration broaden exploration horizons.
- Social Sciences: In addressing natural hazards, input from sociology and psychology helps in understanding community risks and disaster preparedness, ensuring that geophysical findings translate into actionable strategies.
In summary, the future of geophysics promises an intricate framework where technological innovations and collaborative effort push forward the understanding of Earth's processes, addressing contemporary challenges effectively. The focus on diverse and innovative approaches will likely yield better solutions and more profound insights into how our planet operates.
Closure
The conclusion of this article serves multiple purposes, centralizing the insights gained throughout the discussion on geophysics. It synthesizes the critical elements presented, reinforcing the value that geophysics holds in various fields. The importance of summarizing key insights cannot be understated as it provides clarity, allowing readers to retain the most pertinent information. By reflecting on what has been covered, we not only confirm the relevance of the topics discussed but also highlight the ongoing significance of geophysical research in addressing complex earthly challenges.
Another essential aspect of this conclusion is that it provides a moment for reflection. Readers are encouraged to consider how geophysics influences their understanding of natural phenomena. This section aims to merge theoretical knowledge with real-world applications, ensuring that its relevance touches practical fields such as resource exploration, environmental monitoring, and hazard assessment.
Ultimately, the conclusion reiterates the notion that geophysics is not just an academic pursuit but a vital tool for sustainable development. As society faces increasing environmental challenges, the insights and methodologies provided by geophysics will become even more crucial.
Summarizing Key Insights
In summarizing the key insights from this article, several points stand out:
- Definition and Scope: Geophysics involves studying the Earth's physical properties and processes, bridging the gap between geology and physics.
- Diverse Branches: The field is divided into various branches like seismology, geomagnetism, and others, each playing a role in understanding Earth’s dynamics.
- Techniques and Instruments: Numerous techniques and instruments are employed in geophysical investigations, including field surveys and imaging systems that enhance data clarity.
- Applications of Geophysics: The practical applications stretch across natural resource exploration, environmental protection, and disaster management, highlighting its utility in tackling global challenges.
- Future Directions: Innovations in technology and a push for interdisciplinary collaboration indicate future advancements in geophysical research.
This summary serves to consolidate previously discussed points, facilitating a robust understanding of what geophysics encompasses and why it matters in current and future scientific endeavors.
The Importance of Geophysics
Geophysics plays a pivotal role in understanding the planet we inhabit. Its importance can be distilled into a few core areas:
- Resource Management: Geophysics aids in exploration for vital resources such as oil, gas, and minerals. This is essential for energy security and economic stability.
- Environmental Protection: Through monitoring and assessment, geophysics contributes to preserving natural ecosystems and managing natural hazards. Understanding the subsurface can inform better decisions regarding land use and environmental conservation.
- Risk Assessment: Natural hazards, including earthquakes and volcanic eruptions, require precise understanding to mitigate risks. Geophysical techniques can provide crucial data for early warning systems.
- Climate Change Insights: Geophysics increasingly informs climate studies, helping scientists track changes in Earth’s systems and predict future trends.
In summary, the influence of geophysics extends far beyond academic circles. It shapes policies, drives economic strategies, and fosters a sustainable future. With ongoing research and technological advancements, its significance will only continue to grow.