Understanding Electric Car Mechanics and Sustainability
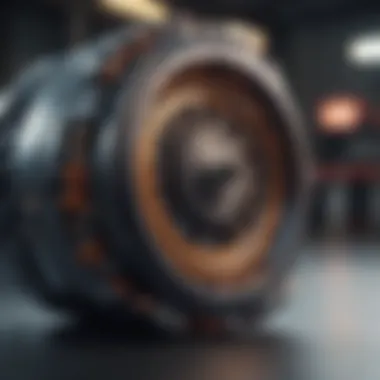
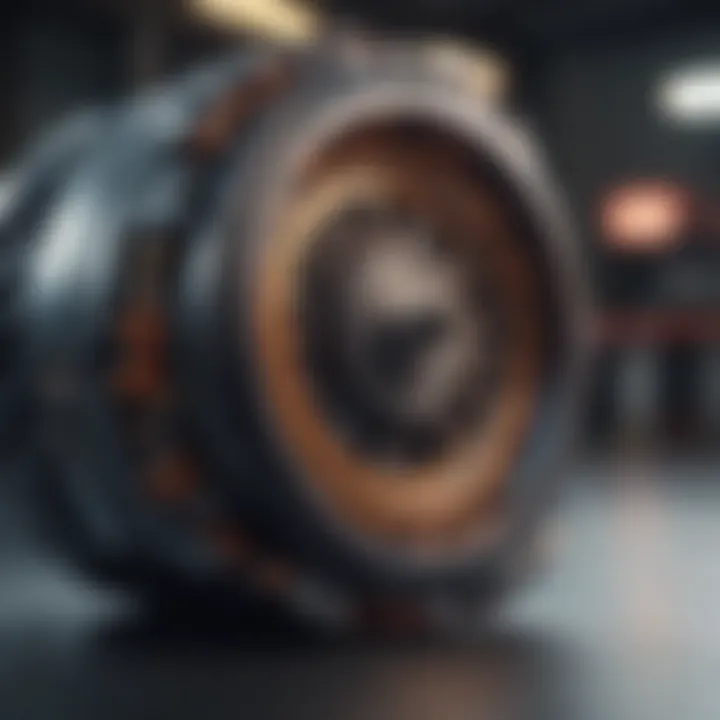
Intro
Electric cars have become a significant focus in the automotive industry. They are often seen as the future of personal and public transportation. Understanding how these vehicles work is crucial for those interested in technology, engineering, and environmental science. This article will clarify the inner workings of electric vehicles and their components, showcasing their impact on sustainability. We'll look at the mechanisms involved in energy conversion, vehicle performance, and how they compare to traditional internal combustion engine vehicles. Additionally, we will touch on the environmental implications of electric car adoption.
Methodology
Study Design
To evaluate the operational mechanisms behind electric cars, a mixed-methods approach is implemented. This combines quantitative data analysis with qualitative insights from experts and users alike. By analyzing performance metrics, battery life, energy efficiency, and user experiences, a comprehensive understanding of electric vehicle functionality can be achieved.
Data Collection Techniques
Data will be collected through various methods, including:
- Surveys: Gathering opinions from electric car users and potential buyers to understand their perspectives and experiences.
- Technical analyses: Evaluating the specifications of various electric car models to spot trends in technology and performance.
- Expert interviews: Discussing insights with automotive engineers and researchers to deepen understanding of emerging technologies.
Discussion
Interpretation of Results
The findings indicate that electric cars utilize several components effectively to achieve efficient functionality. The electric motor converts electrical energy from the battery into mechanical energy, which powers the vehicle. Furthermore, the battery plays a vital role in energy storage, determining the car's range and charging capabilities. Equation-based models can be employed to demonstrate the energy flow within the car.
Limitations of the Study
While the study presents critical insights, there are limitations to consider. The fast-paced development of technology means that data can quickly become outdated. Additionally, varying conditions affecting vehicle performance can impact results, such as temperature and terrain.
Future Research Directions
Future studies could explore innovations in battery technology, like solid-state batteries, which may offer higher energy densities and faster charging times. Another important area is the integration of renewable energy sources for charging infrastructure, embracing a broader scope of sustainability.
"Understanding the mechanisms at play in electric vehicles provides insight into their efficiency and potential for future transportation."
Foreword to Electric Cars
The exploration of electric cars is crucial, given their rapidly increasing significance in the modern automotive landscape. As traditional fossil fuel-based vehicles face scrutiny for their environmental impact, electric vehicles (EVs) present a viable and sustainable alternative. Understanding the mechanisms that drive these vehicles goes beyond mere curiosity; it is fundamental for comprehending how they contribute to reducing carbon emissions and advancing energy efficiency.
Electric cars comprise various components and technologies that work harmoniously to provide a clean and efficient mode of transportation. This article will delve into these critical elements, including electric motors, battery technology, and energy management systems. Each of these plays a pivotal role in the overall functionality and efficiency of electric vehicles.
Definition and Basic Functionality
Electric cars, simply put, are vehicles that utilize electric energy stored in batteries to power an electric motor. Unlike conventional vehicles powered by internal combustion engines, electric cars convert stored electrical energy into mechanical energy to propel the vehicle. This basic mechanism allows for a quieter, smoother driving experience while also emitting zero tailpipe pollutants.
The simplicity of electric cars can be highlighted through two primary functionalities:
- Charging: This involves connecting the vehicle to a power source, allowing it to recharge its battery. Charging methods can vary from standard wall outlets to more sophisticated fast-charging stations.
- Energy Usage: Once charged, the electric motor draws energy from the battery and converts it into motion. This process involves power electronics, which control the flow of electricity between the battery and the motor, ensuring efficient use of energy.
Overall, electric vehicles operate under the principle of energy conservation and efficiency. By significantly reducing reliance on fossil fuels, they not only offer economic benefits in terms of lower fuel costs but also lead to a marked decrease in greenhouse gas emissionsβessential in the fight against climate change.
"Electric vehicles are not just a trend; they represent a transformation in how we think about personal and public transport."
Studying electric cars leads to a deeper understanding of sustainable practices in transportation and the technological advancements that support these innovations.
Key Components of Electric Vehicles
Understanding the key components of electric vehicles is critical for grasping how these modern machines operate. The components include electric motors, battery packs, and power electronics. Each part plays a vital role in ensuring that electric cars function efficiently and meets consumer expectations. A clear comprehension of these elements reveals the benefits and challenges associated with electric vehicles, thus providing insight into the future of automotive technology.
Electric Motor
The electric motor serves as the heart of an electric vehicle. It converts electrical energy into mechanical energy to propel the vehicle forward. This transformation relies on electromagnetic principles. The most common types of electric motors used in vehicles are permanent magnet synchronous motors and induction motors.
Permanent magnet synchronous motors are known for their high efficiency and torque density. This results in better performance while extending the range of the vehicle. On the other hand, induction motors are quite robust, require less maintenance, and are often more cost-effective. Each type has its benefits, affecting overall efficiency and power delivery. For consumers, this means that different vehicles can be tailored to meet various performance preferences.
Battery Pack
Battery packs are fundamental to electric vehicles, storing the energy needed for operation. The most widely used battery type in electric vehicles today is the lithium-ion battery. These batteries are chosen for their high energy density and relatively long life cycle.
Battery packs also require effective management systems to ensure safety and efficiency. Battery management systems monitor charge levels and temperature, optimizing performance and health. Whenever an electric vehicle is charging, the sustainability of its battery pack heavily influences its overall environmental footprint. Consumers should consider the battery size and its impact on range and charging time. In this way, the battery pack is essential not just for performance but also for sustainability.
Power Electronics
Power electronics manage the flow of electrical energy within electric vehicles. They control the conversion of electricity from the battery to the electric motor and vice versa. This section includes inverters, converters, and the power distribution unit.
Inverters convert direct current (DC) from the battery to alternating current (AC) for the motor. Meanwhile, converters can regulate power supply to various vehicle components, ensuring that everything operates under appropriate conditions. Properly designed power electronics can lead to greater efficiency and enhance the driving experience. They are integral in achieving the seamless operation that drivers expect from electric vehicles today.
"A well-integrated power management system is crucial for the reliability and performance of electric vehicles."
The Role of Electric Motors
Electric motors serve as the heart of electric vehicles, converting electrical energy into mechanical power. Their functionality fundamentally impacts efficiency, performance, and the overall driving experience. Understanding electric motors is crucial for grasping how electric vehicles operate and how they differ from traditional combustion engines.
Electric motors are celebrated for their ability to deliver torque almost instantaneously. This characteristic ensures that electric cars can accelerate swiftly, offering remarkable responsiveness to driver commands. Moreover, the design of electric motors allows for a compact and lighter structure compared to a conventional engine setup, which benefits the vehicleβs overall weight and agility.
Types of Electric Motors
There are several types of electric motors employed in electric vehicles, each with distinct advantages. The most common types include:
- DC Motors: These motors are simple in design and relatively inexpensive. They can be categorized into brushed and brushless variants. Brushless DC motors are more efficient and require less maintenance.
- AC Induction Motors: Often used by larger manufacturers, like Tesla, this type harnesses alternating current to create a rotating magnetic field. They boast significant durability and reliability but can be more complex.
- Permanent Magnet Synchronous Motors (PMSMs): These motors use permanent magnets to maintain rotor positioning, which enhances efficiency. However, the need for rare earth materials can present supply chain challenges.
Understanding these types highlights how manufacturers choose motor designs based on specific performance criteria, cost, and application considerations.
Motor Efficiency and Performance
Motor efficiency is critical to the performance of electric vehicles. It directly influences how much energy from the battery translates into usable power for propulsion.
An efficient motor converts a higher percentage of electrical energy into mechanical energy. Factors that affect efficiency include:
- Load Conditions: Motors perform optimally under specific load conditions. Operating outside these conditions can decrease efficiency.
- Temperature: Higher temperatures can affect resistance in the motor windings, leading to energy losses. Adequate thermal management systems are essential for maintaining performance.
- Speed: Efficiency often varies with speed, as different motors have optimal points where their performance peaks.
Moreover, enhancements in motor design and technology are continually improving efficiency. For example, advanced control systems can optimize performance by adjusting the motor's operation to meet varying driving conditions.
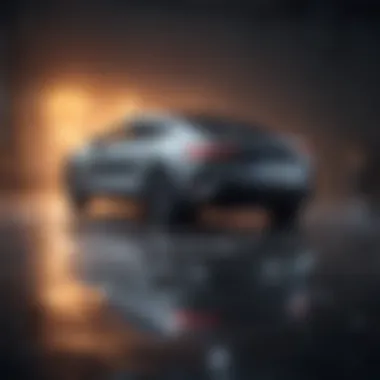
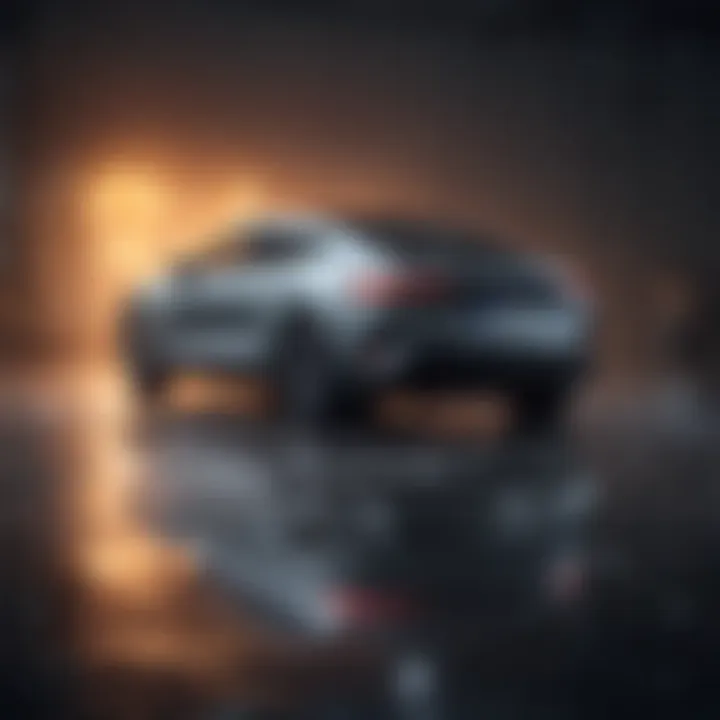
"Motor efficiency is the key to maximizing the range of electric vehicles, allowing more distance to be covered per charge."
Understanding Battery Technology
Batteries are the lifeblood of electric vehicles (EVs). They determine range, performance, and efficiency. Understanding battery technology is crucial to grasp the full scope of electric car functionality.
Modern electric vehicles rely on rechargeable lithium-ion batteries. This chemistry is favored for its high energy density and longevity. The efficiency of batteries directly influences the overall driving experience. A well-designed battery ensures that energy is stored effectively and released on demand, impacting both speed and distance.
Battery Chemistry
The concept of battery chemistry is central to how electric cars operate. Lithium-ion batteries consist of cathodes, anodes, electrolyte, and separator. The cathode often uses lithium cobalt oxide, while graphite is common for anodes. These materials contribute to the batteryβs ability to store and discharge energy.
Advantages of lithium-ion technology include:
- High energy density: More energy is stored per unit weight.
- Efficiency in charging and discharging: Allows for faster charging times and more miles driven.
- Lower self-discharge rates: Retains charge when not in use.
Challenges are also present. For instance, lithium-ion batteries can experience thermal runaway, which poses a safety risk. Research continues to address these issues, focusing on developing more stable materials and chemistries.
Charging Cycles and Longevity
Charging cycles are a key factor influencing battery life. A single cycle encompasses a full discharge followed by a full recharge. Each battery has a finite number of cycles before performance declines. Typically, lithium-ion batteries can endure between 500 to 1500 cycles, depending on usage and conditions.
To prolong battery longevity, consider these aspects:
- Optimal charging habits: Frequent, partial charges help maintain capacity.
- Temperature control: Storing batteries within ideal temperature ranges preserves performance.
- Avoid deep discharges: Keeping battery levels above 20% is recommended.
Regular monitoring can extend the lifespan, allowing users to maximize their vehicles.
Battery Management Systems
Battery Management Systems (BMS) play a critical role in the performance and safety of electric vehicle batteries. The BMS monitors individual cells, balancing charge and discharge to ensure uniformity across the pack. It protects against overcharging, overheating, and deep discharging. This system enhances the reliability of the battery and the vehicle as a whole.
Key functions of a Battery Management System include:
- Cell monitoring: Ensures each cell operates within safe limits.
- Thermal management: Maintains optimal operating temperatures.
- State of Charge (SoC) calculations: Assess the remaining energy and estimate range.
Energy Conversion in Electric Vehicles
The process of energy conversion is central to the functionality of electric vehicles. Without an efficient mechanism to transform stored electric energy into motion, electric cars would not provide the performance and convenience they do today. Understanding this conversion not only reveals the technology behind electric vehicles but also emphasizes their potential benefits over traditional combustion engines.
Electric vehicles rely on electric motors, rather than internal combustion engines, to convert electrical energy stored in batteries into mechanical energy. This transformation occurs through the principles of electromagnetism, where electric current generates a magnetic field, causing the motor to spin. The efficiency of this conversion greatly impacts the overall performance of the vehicle, determining factors such as acceleration, top speed, and range.
Considering the growing demand for sustainable transportation solutions, evaluating energy conversion mechanisms is crucial. Improved designs and technologies can increase the efficiency of energy use, ultimately supporting greater sustainability and enhancing the driver experience.
From Electric Energy to Motion
The process of converting electric energy into motion begins with the battery pack supplying power to the electric motor. When the driver presses the accelerator, a signal is sent to the power electronics, which manage the flow of electricity. This control system ensures that the right amount of energy is provided to the motor, optimizing performance depending on the vehicle's requirements.
The motor converts electrical energy into mechanical energy via rotation. There are different types of electric motors, including DC motors and AC motors, each with its own advantages and suitable applications. For instance, synchronous AC motors provide high efficiency and torque at various speeds, making them ideal for many electric vehicle models.
This transformation process is typically very efficient, often reaching over 90% in many modern vehicles. This efficiency is in stark contrast to traditional internal combustion engines, which may only convert approximately 20% of the energy in fuel into usable motion, with the remainder lost as heat. Thus, electric vehicles showcase significant advancements in energy use and management.
Regenerative Braking Systems
Regenerative braking systems represent a significant advancement in the energy conversion processes of electric vehicles. This system allows vehicles to recover some energy during the braking process, rather than losing it entirely as heat, as is typical in conventional braking systems.
When the driver applies the brakes, the electric motor can be switched to operate in reverse mode. In this mode, instead of consuming energy, the motor acts as a generator that converts kinetic energy from the vehicle's motion back into electrical energy. This recovered energy is then fed back into the battery pack for later use, enhancing the overall efficiency of the vehicle.
The benefits of regenerative braking are considerable. Not only does it extend the range of electric vehicles by maximizing energy use, but it also reduces wear on brake components, leading to lower maintenance costs over the vehicle's lifespan. However, the effectiveness of regenerative braking can vary based on various factors like road conditions and driving patterns, which should be considered to optimize its usage.
Regenerative braking systems can capture up to 30% of the energy typically lost during braking, significantly contributing to an electric vehicle's range and efficiency.
Charging Infrastructure
Charging infrastructure is the backbone of electric vehicle (EV) adoption. As electric cars become more prevalent, the availability and efficiency of charging stations play a crucial role in the ease of integration into daily life. For drivers, knowing where they can charge their vehicle affects purchasing decisions. A well-developed charging network can alleviate concerns about range and accessibility, making electric vehicles a more attractive option.
Types of Charging Stations
There are several types of charging stations designed to meet various needs and situations:
- Level 1 Charging Stations: These stations use standard household outlets and are typically the slowest option. They are ideal for overnight charging at home.
- Level 2 Charging Stations: With higher voltage, these units are commonly found in public spaces like shopping centers and parking garages. They provide a balance between speed and accessibility, usually taking a few hours to fully charge an EV.
- DC Fast Charging Stations: These are the fastest charging options, capable of significantly reducing charging time. They are often located along major highways, enabling long-distance travel.
Each type of charging station serves a unique purpose. Level 1 is suitable for home use, while Level 2 and DC Fast Charging facilitate more immediate needs during travel.
Charging Speed and Accessibility
Charging speed varies among the different types of stations. While Level 1 may take up to 24 hours for a complete charge, Level 2 typically charges an electric car in four to six hours. Conversely, DC Fast Charging can boost an EVβs battery to 80% in as little as 30 minutes, appealing to users needing quick turnaround times.
Accessibility is equally important. Urban areas must prioritize station placement to serve a larger number of drivers effectively. Considerations include traffic patterns, urban planning, and proximity to amenities. As the market for electric cars grows, ensuring sufficient, strategically placed charging stations becomes essential for supporting widespread adoption.
Properly designed charging infrastructure can increase the adoption rate of electric vehicles and contribute to more sustainable urban environments.
Comparative Performance Metrics
Comparative performance metrics form a crucial part of understanding electric vehicles. It provides a quantitative basis for assessing how electric cars measure up against traditional combustion engine vehicles. These metrics help consumers, researchers, and manufacturers make informed decisions based on efficiency, usability, and environmental impact.
One important metric is range, which directly influences how practical an electric vehicle is for everyday use. Consumers often prioritize the distance an electric car can travel on a single charge. Thus, understanding a vehicle's range directly impacts purchase decisions and overall consumer confidence. The range is influenced by battery capacity, vehicle weight, and driving conditions.
Additionally, efficiency becomes pivotal in performance analysis. Electric vehicles often demonstrate a higher energy efficiency than their gasoline counterparts, converting a greater percentage of energy from the battery to actual driving power.
"Performance metrics not only guide consumer choices but also help in shaping manufacturer strategies and policies."
Range and Efficiency Analysis
Range and efficiency are often discussed together because they are interdependent. The range of an electric vehicle can vary significantly based on several factors, such as the capacity of the battery pack, weight of the vehicle, and driving habits. For instance, aggressive acceleration and high speeds can deplete battery energy faster, thus reducing the effective range.
A well-engineered electric vehicle might have a range of over 300 miles per charge, making it suitable for long-distance travel. In combination, a high-efficiency motor and battery technology can contribute to an optimal range. Manufacturers are constantly evaluating battery technology, with advancements in lithium-ion batteries bringing significant improvements in both energy density and longevity.
To illustrate, consider the following aspects influencing range and efficiency:
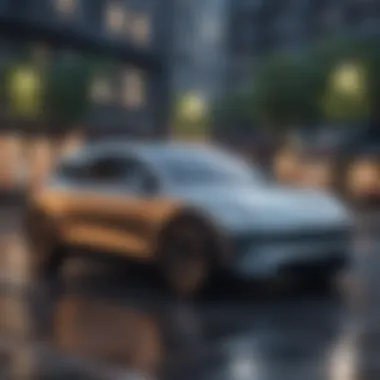
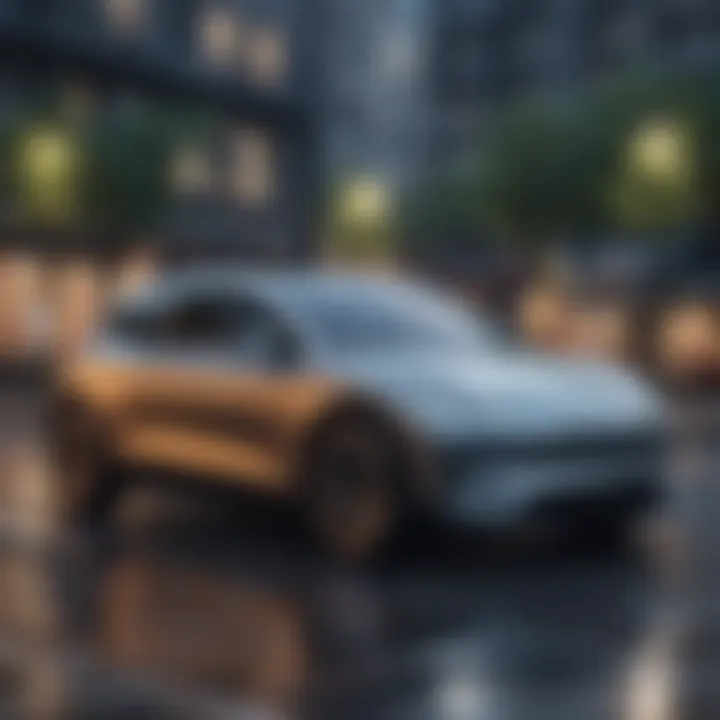
- Battery chemistry and capacity
- Weight distribution and aerodynamics
- Driving conditions and styles
Acceleration and Handling
Acceleration and handling are essential performance metrics that set electric vehicles apart from traditional vehicles. Electric motors typically deliver maximum torque instantly, resulting in swift acceleration. This characteristic offers a unique driving experience, often leading to quicker off-the-line speeds.
Handling is another crucial aspect influenced by the vehicle's design and weight distribution. With batteries usually located at the floor level, electric vehicles offer a lower center of gravity, enhancing stability during turns and curves.
These features result in a unique driving dynamics, making electric vehicles not only efficient but also exhilarating to drive. As automakers continue to innovate, the balance of speed, handling, and controllability will further define the performance landscape of electric vehicles.
Environmental Implications
The environmental implications of electric cars are integral to understanding their role in contemporary transportation. The shift from traditional internal combustion engines to electric vehicles (EVs) is not just a technological advancement but a necessity in addressing global environmental challenges. Electric cars present a compelling solution to reduce harmful emissions, alleviate urban air pollution, and combat climate change. However, it is important to analyze their lifecycle and overall impact to comprehend their full environmental promises and pitfalls.
Lifecycle Assessment of Electric Vehicles
A lifecycle assessment (LCA) of electric vehicles involves evaluating the environmental impact of a vehicle from production through to disposal. This approach highlights various stages:
- Manufacturing Phase: The production of electric cars, particularly battery production, entails significant energy use and resource extraction. Lithium-ion batteries, commonly used in EVs, require materials such as lithium, cobalt, and nickel. Mining these materials can result in ecological degradation and pollution.
- Operational Phase: Once in use, electric vehicles offer substantial benefits. They produce zero tailpipe emissions, which improves air quality, especially in densely populated areas. The electric energy they consume can be sourced from renewable resources, further minimizing their carbon footprint.
- End-of-Life Phase: At the end of an electric vehicle's life, recycling processes for batteries and other components are crucial. Proper disposal or repurposing can mitigate landfill waste and encourage sustainable practices in materials recovery.
In summary, while electric vehicles are often promoted as clean alternatives, a comprehensive lifecycle assessment reveals that their production and recycling still present challenges. This evaluation is vital to enhance development processes that reduce environmental harm at all stages.
Impact on Air Quality and Carbon Emissions
Electric vehicles significantly contribute to improved air quality by eliminating exhaust emissions. Traditional vehicles release harmful pollutants, including nitrogen oxides and particulate matter, which contribute to smog and respiratory issues in urban environments.
"Switching to electric vehicles can lead to a marked decrease in air pollution, benefiting public health and enhancing overall quality of life."
The reduction in carbon emissions is another critical advantage. While the electricity used may still produce some emissions, many regions are increasingly powering their grids with renewable energy sources like wind, solar, and hydroelectric power. This transition diminishes the carbon footprint associated with electric vehicle usage.
- Key elements of the impact:
- Decreased dependency on fossil fuels for transportation.
- Improved health outcomes because of better air quality.
- Adoption of renewable energy sources leading to lower carbon emissions.
As electric vehicles become more prevalent, their combined effects on air quality and greenhouse gas emissions can contribute significantly to mitigating climate change. However, it is essential to continue monitoring the environmental impacts associated with their production and energy sourcing. Achieving optimal benefits requires ongoing advancements in technology, recycling methods, and clean energy integration.
Future Trends in Electric Vehicle Technology
The advancement of electric vehicle technology is pivotal to the future of transportation. It shapes the automotive landscape and defines the standards for sustainable mobility. In this section, we explore two critical themes: the advancements in battery technology and the integration of autonomous systems. These elements are interconnected with ongoing research, regulatory influences, and market demands. By understanding these trends, stakeholders can prepare for the evolving nature of electric vehicles and their wider adoption in society.
Advancements in Battery Technology
Battery technology has progressed significantly over the past years. Innovations in this area are vital for enhancing the performance and viability of electric vehicles. Lithium-ion batteries dominate the current market, but researchers are exploring alternatives that could offer better energy density, charging speeds, and longevity. Solid-state batteries, for instance, show immense promise. These batteries use a solid electrolyte instead of a liquid one, potentially leading to increased energy density and improved safety.
Key advancements include:
- Higher Energy Density: Increasing the amount of energy that can be stored in a given volume. This can extend the range of electric vehicles, alleviating concerns over "range anxiety" among consumers.
- Fast Charging Technologies: Development of technologies that could charge batteries in minutes rather than hours, making electric vehicles more convenient for everyday use.
- Recycling and Sustainability: Improvements in battery recycling processes are essential as demand grows. This ensures that valuable materials are reused and reduces the environmental impact of battery production.
Constant innovation in battery technology will be essential for the widespread adoption of electric vehicles. It will create opportunities for enhancing user experience and minimizing ecological footprints.
Integration of Autonomous Systems
The integration of autonomous systems into electric cars is another transformative trend. This synergy can lead to enhanced safety, convenience, and efficiency on the roads. Utilizing advanced sensors, cameras, and AI, electric vehicles can be equipped with features such as adaptive cruise control and automated parking.
Moreover, autonomous systems complement electric vehicle architecture. Here are some factors to consider:
- Safety: Autonomous driving technologies can reduce human error, one of the leading causes of accidents. Enhanced safety systems will encourage more people to transition to electric vehicles.
- Traffic Management: Self-driving cars can communicate with each other and infrastructure, optimizing traffic flow and reducing congestion. This connection can lead to more efficient use of roadways.
- User Experience: As driving becomes more automated, passengers in electric vehicles will have the opportunity to engage in other activities, transforming their travel experience.
The blend of electric vehicle technology with autonomous systems not only offers practical benefits but also reflects a shift in how we perceive mobility in the future. As research continues, these advancements are expected to evolve further, shaping the next generation of electric vehicles.
Regulatory and Economic Factors
The regulatory and economic factors play a crucial role in accelerating the adoption of electric vehicles. Governments and industry stakeholders create frameworks that influence the development, manufacturing, and sales of electric cars. Understanding these factors can provide key insights into market dynamics and consumer behavior. Moreover, the intersection of policies and economics can significantly impact the overall perception of electric cars.
Government Incentives and Policies
Government initiatives are vital in fostering the growth of the electric vehicle industry. Various incentives exist to encourage consumers to purchase electric cars. Some of these include tax credits, rebates, and grants which enable consumers to reduce the total cost of ownership.
Additionally, some regions have set ambitious targets for reducing greenhouse gas emissions. This often involves mandates for automakers to increase the number of electric vehicles in their fleets. Policies such as zero-emission vehicle (ZEV) mandates ensure that manufacturers produce a certain percentage of electric models, thus promoting the transition to cleaner technologies.
In certain countries, charging infrastructure investments are subsidized, making it easier for businesses and local governments to deploy charging stations. Enhanced charging access is essential for the practicality of electric vehicles, and incentives in this area foster widespread adoption.
Market Trends and Consumer Adaptation
The market for electric vehicles is rapidly evolving, driven by both consumer preferences and advancements in technology. An increasing number of consumers are becoming environmentally conscious, which impacts their buying decisions. Data shows that many individuals are willing to invest in electric vehicles if they perceive them as viable alternatives to traditional cars.
Consumers are also influenced by social factors, such as the presence of charging stations and the visibility of electric vehicles in their communities. When electric vehicles become a regular sight on the streets, they contribute to normalizing use and acceptance. Furthermore, the performance and technological advancements have made electric models more appealing.
In terms of market trends, the competition among manufacturers is intensifying. Major automotive brands like Tesla, Nissan, and Chevrolet, among others, are innovating to capture market share. Many offer different price points and features, appealing to a broader audience.
This competitive landscape is also forcing traditional car manufacturers to adapt. Many are investing heavily in electric technology to respond to changing consumer demands and regulatory pressures. As the market matures, collaboration between governmental bodies and automotive manufacturers will enhance infrastructure development and consumer education efforts.
"The regulatory landscape is not just a hurdle; it can be a catalyst for innovation and adaptation in the electric vehicle market."
In summary, understanding regulatory and economic factors is essential for comprehending the rapid evolution of electric vehicles. By following government incentives and market trends, stakeholders can make informed decisions that align with industry advancements.
Challenges Facing Electric Vehicles
The expansion of electric vehicles (EVs) in the automotive market is aligned with goals of sustainability and reduction of fossil fuel dependency. However, the rise of electric cars is not without its obstacles. Understanding the challenges that electric vehicles face is crucial for addressing barriers to adoption and ensuring their long-term success.
Infrastructure Limitations
Infrastructure for electric vehicles plays a pivotal role in supporting their growth. A well-developed network of charging stations is essential, yet current availability is often lacking. This limitation manifests in several ways:
- Charging Station Distribution: Many areas, especially rural regions, have insufficient charging stations. This discrepancy creates "charging deserts," where potential EV owners hesitate to commit to electric cars due to fears about charging accessibility.
- Variety of Charging Types: Different EVs require specific types of chargers, such as Level 1, Level 2, and DC fast chargers. A lack of universal charging solutions leads to compatibility issues and complicates the charging process for consumers.
- Time for Recharging: Charging an electric car typically takes longer than filling a tank with gasoline. If charging stations are few and far between, this can lead to significant waiting times. The inconvenience might deter consumers from switching to electric vehicles.
These infrastructure limitations underscore the need for investment in charging facilities and innovations in charging technology. Overcoming these barriers will facilitate a smoother transition for consumers and promote the widespread adoption of electric vehicles.
Battery Disposal and Recycling Issues
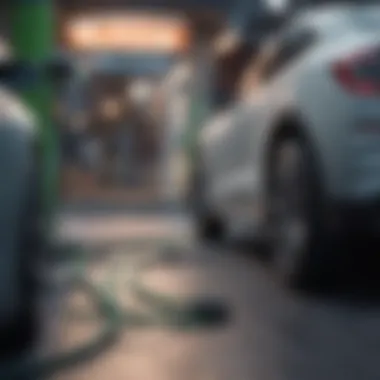
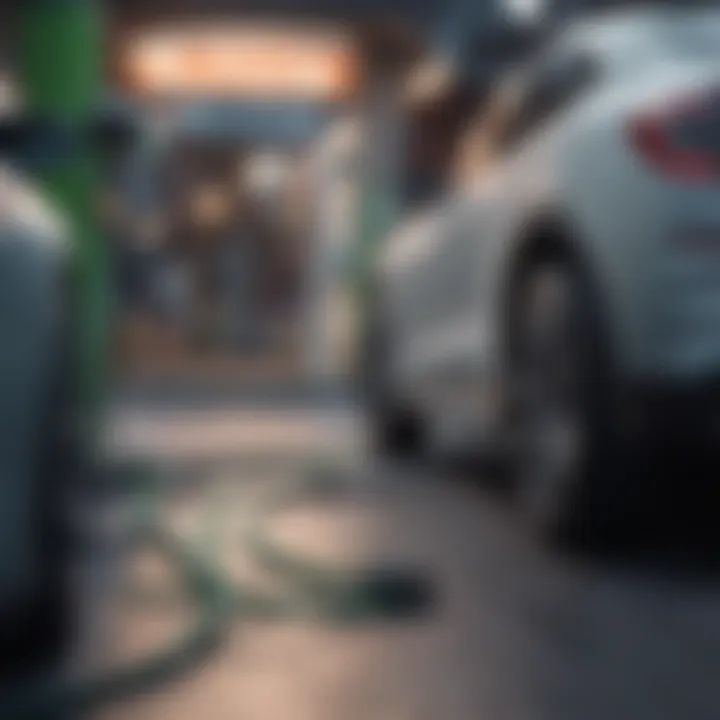
As the market for electric vehicles grows, so does the concern surrounding battery disposal and recycling. The batteries in electric vehicles, primarily lithium-ion, present environmental and logistical challenges that must be addressed. Some key points to consider include:
- Toxic Materials: Lithium-ion batteries contain hazardous materials that can pose risks to ecosystems if not disposed of properly. The potential for water and soil contamination is a serious concern that requires effective management strategies.
- Recycling Processes: The current recycling processes for EV batteries are not yet efficient or widely implemented. Many batteries end up in landfills, which is not environmentally sustainable. Advancements in battery recycling technologies are necessary to reclaim valuable materials.
- Regulatory Framework: The lack of standardized regulations regarding battery disposal creates confusion and inconsistency. This can hinder efforts to promote safe and effective recycling practices across the industry.
Emphasizing proper disposal and recycling is essential for the long-term sustainability of electric vehicles. Educational initiatives aimed at informing consumers about disposal options are also crucial.
The transition to electric vehicles is integral to achieving broader environmental goals, but infrastructure and recycling must be urgently addressed to ensure their viability in the future.
Understanding these challenges provides insight into the dynamics of electric vehicle adoption and opens discussions on how to effectively tackle these pressing issues.
Case Studies of Electric Vehicle Implementations
Case studies provide a pivotal lens through which the real-world application of electric vehicles can be examined. They illustrate not only how these vehicles operate in various environments but also highlight the myriad benefits and considerations associated with their integration into different sectors. By exploring case studies, readers can grasp the practical implications of electric vehicle technology, including aspects such as efficiency, user satisfaction, and infrastructural challenges. In this context, two specific areas are important: urban adoption and commercial fleet utilization.
Successful Adoption in Urban Areas
Electric vehicles have gained traction in urban settings for several reasons. Cities often face severe air quality issues and traffic congestion, making the transition to cleaner transportation solutions pressing. Case studies from cities like San Francisco and Amsterdam exemplify successful electric vehicle integration into public transportation systems. These cities have enacted policies that favor electric buses and cars, resulting in reduced emissions and enhanced public health.
- Challenges Overcome: Urban areas typically struggle with space constraints. Electric vehicles, being more compact and efficient, minimize the requirements for parking and fuel infrastructure.
- Public Transport Systems: Cities have initiated programs designed to electrify their buses, significantly lowering greenhouse gas emissions compared to diesel alternatives. For instance, the deployment of electric tram networks in various European cities has proven to be beneficial for urban mobility.
- Government Incentives: Many city governments are also adopting financial incentives for electric vehicle purchases, encouraging residents to shift from traditional combustion engines.
The results from these initiatives have shown promising trends. User satisfaction tends to be higher, partly due to reduced operational costs and quieter, smoother rides. Furthermore, studies show a correlation between electric vehicle adoption and increased investment in local charging infrastructure, ensuring that urban electric vehicle ecosystems grow effectively.
Electric Fleet Vehicles in Commercial Usage
The utilization of electric vehicles in commercial fleets marks another successful case study area, showcasing significant advantages in terms of cost-efficiency and sustainability. Companies such as Amazon, UPS, and DHL have invested heavily in electric delivery vans.
- Cost Savings: Multiple studies indicate that firms that transition to electric fleet vehicles can significantly reduce fuel and maintenance costs. For example, electric vans have lower operational costs over their lifetime compared to their gasoline counterparts.
- Greener Logistics: Electric fleet initiatives enhance corporate sustainability goals, reducing the overall carbon footprint. Alongside operational benefits, these measures have also been linked to better public perception and brand loyalty among consumers who value eco-friendly practices.
- Challenges to Implementation: While the transition is beneficial, challenges remain. Charging infrastructure must be developed, and companies need to address range anxiety when planning logistics and deliveries.
"The shift towards electric fleet vehicles is not only a strategic business decision but also a necessary step toward reducing our environmental impact."
In summary, the exploration of successful electric vehicle implementations in urban areas and their use in commercial fleet operations provides valuable insights into the mechanisms that facilitate the transition to electric mobility. These case studies underscore the complexity and potential of electric vehicles as they become an integral part of sustainable transportation solutions.
The Role of Research and Innovation
Research and innovation are central to the evolution of electric vehicles. As the automotive industry shifts towards more sustainable practices, understanding this role is crucial. Innovative research underpins advancements in battery technology, motor efficiency, and energy management systems. By fostering this research, we can address current challenges and improve the overall performance and affordability of electric cars.
One significant benefit of research in this field is its ability to enhance battery life and efficiency. Ongoing studies focus on developing lithium-sulfur and solid-state batteries. These alternatives promise increased energy density, reduced charging times, and improved safety. Therefore, effective innovation can significantly expand the practical range and usability of electric vehicles.
Additionally, research initiatives facilitate breakthroughs in charging infrastructure. With increased investment, researchers explore wireless charging systems and fast-charging technologies that radically improve user convenience. Ensuring quick and accessible charging options can heavily influence consumer adoption.
"The continuous evolution of battery and motor technology defines the potential of electric vehicles in real-world applications."
Reducing costs is another primary benefit derived from research and innovation. Enhanced production techniques and material sciences can lower the expense of components, ensuring that electric vehicles become more competitive with traditional combustion engines.
Ongoing Research Initiatives
Ongoing research initiatives focus on numerous aspects of electric vehicle technology. Universities, research institutions, and private companies worldwide are collaborating to accelerate innovations. Notable areas of exploration include:
- Battery technology advancement: Research aims to create batteries that last longer and recharge faster. This includes optimizing materials and chemical compositions that enhance energy density.
- Electric powertrain optimizations: Efforts are being made to refine electric motors, focusing on efficiency and overall vehicle performance to meet diverse driving conditions.
- Sustainability in production: Many projects aim to reduce the carbon footprint associated with battery manufacturing processes, addressing the environmental concerns tied to resource extraction.
These initiatives are critical for creating a robust electric vehicle landscape that meets consumer and market demands.
Collaborative Efforts in Industry and Academia
Collaboration between the automotive industry and academic institutions is vital for the advancement of electric car technology. This synergy encourages knowledge sharing, resource pooling, and coordination of projects that can lead to significant breakthroughs. Key aspects of this collaboration include:
- Joint Research Projects: Collaborative studies often tackle specific challenges, such as enhancing battery efficiencies or refining manufacturing methods.
- Internships and Practical Experience: Partnering with universities allows students to gain real-world experience, offering fresh perspectives and potential new solutions to existing problems.
- Funding and Resource Allocation: Industry partnerships can channel funds into important research areas, speeding up the timeline for technological advancements.
By engaging in active collaboration, both academia and industry can effectively drive the future of electric vehicles forward, ensuring that innovations translate into practical applications that benefit society as a whole.
Public Perception and Awareness
Public perception and awareness play vital roles in shaping the electric vehicle market. Understanding how people view electric cars influences their adoption rates and market dynamics. In this section, we will explore the significant aspects of public perception, as well as the benefits and considerations surrounding awareness initiatives.
Attitudes Towards Electric Vehicles
Attitudes towards electric vehicles vary significantly among different demographics. Many individuals recognize the environmental benefits associated with electric cars, such as reduced emissions and decreased reliance on fossil fuels. However, others harbor skepticism regarding the practicality and reliability of electric vehicles. Some common concerns include charging infrastructure, battery lifespan, and overall performance compared to traditional combustion engines.
Factors influencing these attitudes often stem from misinformation or lack of firsthand experience with electric vehicles. As awareness improves, attitudes tend to shift positively. Electric vehicles are also viewed as technologically advanced and innovative, attracting consumers who value modernity.
"Consumer perceptions can dictate market success. Addressing misconceptions is crucial for advancing electric vehicle adoption."
Education and Outreach Efforts
Education and outreach programs are essential to improving public perception and fostering positive attitudes towards electric vehicles. These efforts can take various forms, including workshops, information sessions, and community events. Engaging the public through educational initiatives demystifies electric cars and highlights their benefits.
Effective outreach includes partnerships with local governments, schools, and environmental organizations. Such collaborations create comprehensive programs that encourage electric vehicle usage and provide clear information about their advantages and operating mechanisms.
Additionally, leveraging social media platforms like Facebook and engaging communities on forums such as Reddit can amplify outreach effects. As people share experiences and information, the collective understanding of electric vehicles can deepen, leading to increased acceptance and interest in this sustainable transport option.
Key elements of successful education and outreach efforts include:
- Demonstrations of Electric Vehicle Features: Allow potential users to experience electric cars firsthand.
- Informative Campaigns: Disseminate factual information to counter stereotypes and false beliefs.
- Community Engagement: Involve local communities in discussions surrounding electric vehicles and their impact.
In summary, public perception and awareness are critical in driving electric vehicle adoption. Addressing misconceptions and enhancing understanding can lead to more favorable attitudes, thereby supporting the growing market for these vehicles.
End
The conclusion of this article encapsulates the essence of understanding the mechanisms behind electric car functionality. It is essential to dissect the multilayered components and processes that drive electric vehicles. Recognizing how electric motors, batteries, and control systems interact offers profound insights into their operational reliability. Moreover, grasping these mechanisms highlights not only the technological advancements achieved but also the long-term sustainability potential that electric cars represent.
Summary of Key Insights
Several pivotal insights emerge from this exploration. Electric vehicles stand out for their efficiency and minimal environmental footprint compared to traditional combustion engines. Understanding the chemistry behind batteries illuminates how energy storage is optimally managed. Furthermore, recognizing the role of power electronics clarifies how electric energy is ultimately converted into reliable motion.
Key points include:
- Electric Motors: Different types and their efficiencies contribute significantly to vehicle performance and energy consumption.
- Battery Technology: Ongoing research in lithium-ion and solid-state batteries reveals pathways for improving energy density and lifecycle.
- Charging Infrastructure: The development of fast-charging solutions and extensive charging networks is crucial for widespread adoption.
- Environmental Impact: Despite manufacturing challenges, electric vehicles offer a pathway to reducing carbon emissions.
Future Outlook
Looking ahead, the future of electric car technology holds great promise. Innovations in battery technology, such as improved recycling processes and alternative chemistries, are on the horizon. These advancements aim to enhance battery life and reduce costs, making electric cars more accessible to consumers.
Additionally, the integration of autonomous systems could reshape electric vehicle functionality. Self-driving technology may synergize with electric vehicles' efficiency for optimized travel experiences.
The transition toward electric vehicles is not merely a technical endeavor. It encompasses broader economic, social, and environmental considerations. As regulatory frameworks support sustainability initiatives, the electric vehicle market will likely continue to flourish, presenting both opportunities and challenges.
"Understanding the mechanisms of electric vehicles is crucial for grasping the future of transportation and energy consumption."