Understanding EEG Cap Electrodes: A Detailed Overview
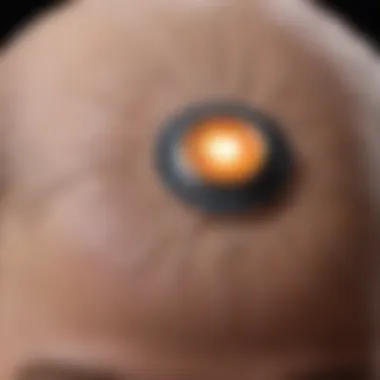
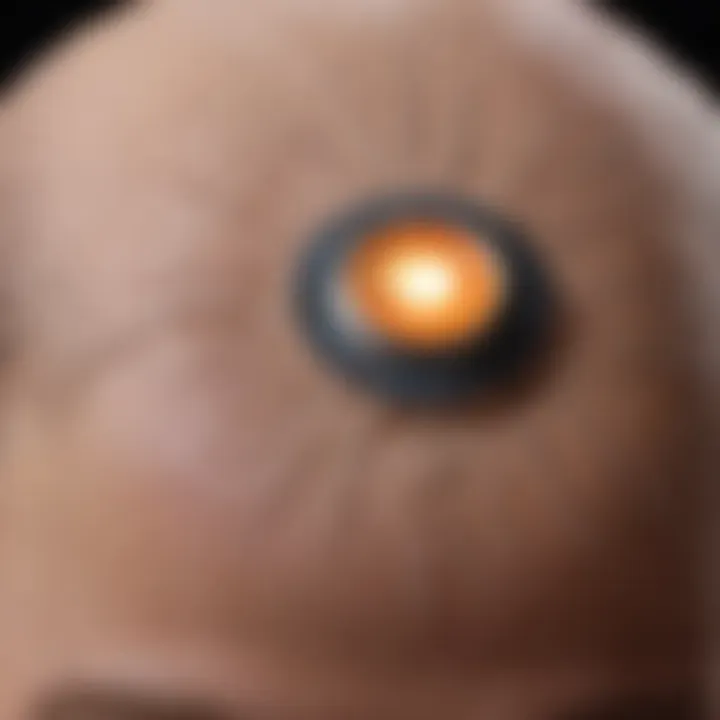
Intro
Electroencephalography (EEG) is a powerful tool used for studying brain function and diagnosing neurological disorders. At the heart of this technology are EEG cap electrodes, which play a crucial role in capturing the electrical activity of the brain. Understanding these electrodes is essential for anyone interested in neurophysiology, whether in research or clinical settings.
EEG cap electrodes are designed for various purposes, from clinical assessments to research in cognitive sciences. Their effectiveness hinges on both their design and placement. In this article, we will cover the intricacies of these electrodes, their functionality, and their broader implications in neurophysiological studies.
We will also tackle specific challenges faced during electrode placement and the interpretation of the resultant data. Finally, we will explore the future of EEG technology and how it can shape the understanding of neural processes.
Methodology
Study Design
To effectively highlight the significance of EEG cap electrodes, a comprehensive study design is essential. It involves examining various aspects such as the configuration of electrodes, material used, and how these features influence data quality. This study should also consider the varying applications, both in research and clinical practices, to give a complete picture of how EEG cap electrodes function in different scenarios.
Data Collection Techniques
Collecting data on the performance and efficacy of EEG cap electrodes requires rigorous methodology. Common techniques include:
- Experimental setups: Utilizing standard EEG configurations to assess performance under comparable conditions.
- Participant studies: Involving different demographics to understand how age, gender, or neurological condition might affect data collection.
- Software analysis: Using advanced algorithms to analyze signal integrity and noise levels.
The use of multiple data collection techniques ensures a robust understanding of EEG cap electrodes and their implications in various fields.
Discussion
Interpretation of Results
Once data is collected, it is imperative to interpret the results effectively. The electrodes' ability to capture relevant signals can vary significantly based on placement, skin conductivity, and design features. A thorough understanding of these variables is critical in drawing accurate conclusions from the data.
Limitations of the Study
While this exploration of EEG cap electrodes aims to be comprehensive, several limitations must be acknowledged. For instance, differences in study designs can lead to varied results. Additionally, there may be external factors that influence data collection, such as environmental noise or participant factors like anxiety levels during testing.
Future Research Directions
Looking ahead, the advancements in EEG technology are promising. Future research could focus on:
- Improved electrode materials that enhance conductivity and reduce noise.
- Innovative designs that provide better comfort and usability for longer monitoring periods.
- Integration with artificial intelligence to improve data interpretation and identification of patterns in brain activity.
Understanding these areas will foster developments in both clinical practices and research methodologies in neurophysiology.
"The future of EEG technology holds vast potential for enhancing our understanding of brain function and improving patient outcomes in clinical settings.”
As we move forward, the role of EEG cap electrodes remains pivotal in bridging the gap between technical proficiency and therapeutic applications in neuroscience. This field continues to evolve, and staying updated is crucial for anyone involved.
Prelude to EEG Cap Electrodes
The study of electroencephalography (EEG) is central to understanding brain activity. Throughout this article, the focus will be on EEG cap electrodes, essential tools used in both clinical and research settings. These electrodes play a significant role in capturing electrical signals generated by the brain's neurons.
EEG cap electrodes provide a non-invasive means to analyze brain functions. They are designed to be placed on the scalp, allowing technicians to record electrical signals with minimal discomfort to the subject. Their importance extends beyond simple measurement; they enable researchers to investigate various neurological conditions, track cognitive developments, and study the basic mechanisms of brain function.
Moreover, EEG caps have evolved over time, leading to advances in materials and configurations. Understanding the development and function of these components allows us to appreciate their contribution to neuroscience. Recognizing the standards for configuration and placement also ensures that data collected is reliable, facilitating better interpretation and application.
Definition and Purpose
Electroencephalography caps, equipped with multiple electrodes, serve a straightforward yet critical purpose: to monitor and analyze the brain's electrical activity. These electrodes act as sensitive receivers, capturing signals that emanate from the brain during various states, such as sleep, concentration, or even relaxation.
The primary goal of EEG cap electrodes is to provide real-time data that can aid both diagnosis and research. In clinical settings, they help healthcare providers detect abnormal brain activity and assist in diagnosing conditions like epilepsy and sleep disorders. In research, they empower scientists to explore cognitive processes, brain-computer interfaces, and neurofeedback methodologies.
The flexibility of EEG caps allows them to adapt to various scenarios. Design differences may cater to different research requirements or patient needs, showcasing their versatility in the field of neuroscience.
Historical Development
The journey of EEG caps and their electrodes began in the early 20th century with the pioneering work of Hans Berger. His invention of the first EEG recording techniques set the stage for the development of modern electroencephalography.
Originally, EEG devices used single electrodes to measure brain activity, limiting the spatial resolution of recorded data. Over time, as the understanding of the brain's structure and function improved, the need for more sophisticated measurements became evident. This led to the development and implementation of multiple electrode systems, resulting in the EEG cap as we know it today.
In the 1950s, the introduction of the International 10-20 system standardized electrode placement, greatly improving data consistency and comparability across studies. This continuous evolution reflects a growing appreciation for the complexity of brain activity and the necessity of accurate measurement tools in both clinical and scientific investigations.
In summary, EEG cap electrodes represent a vital advancement in neurophysiology. They bridge the gap between theoretical research and practical applications, facilitating critical insights into how our brain processes information and responds to external stimuli.
Components of EEG Caps
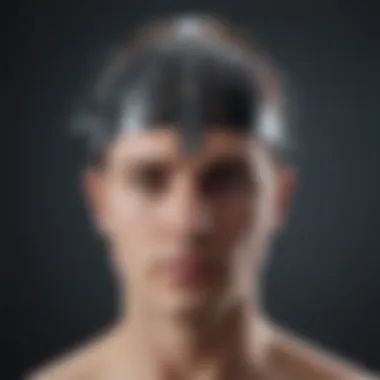
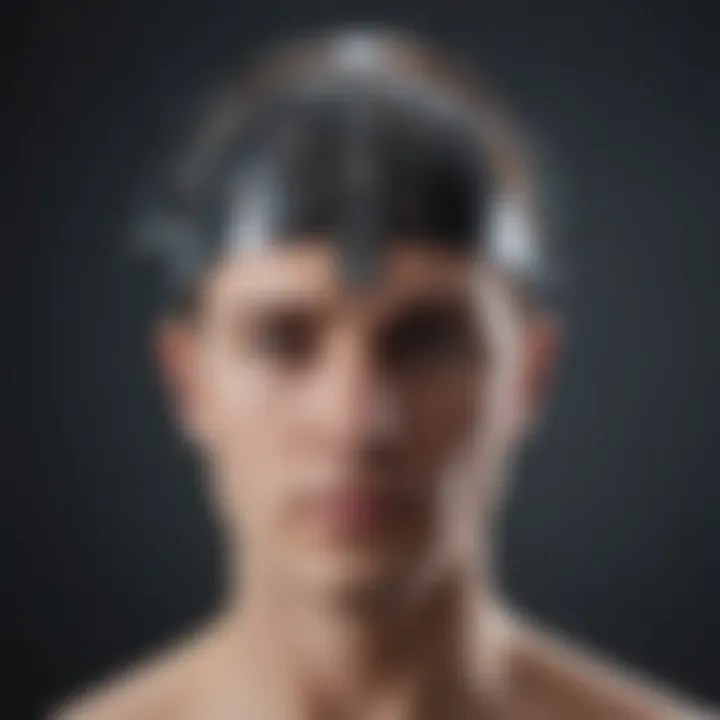
Understanding the components of EEG caps is crucial for appreciating their role in both research and clinical settings. These components must work harmoniously to ensure accurate data capture and reliable interpretation of brain activity. The effectiveness of an EEG cap lies in two main elements: the materials used for electrodes and the design and configuration of the cap itself.
Electrode Materials
Electrode materials play a significant role in the performance of EEG caps. The choice of materials affects conductivity, sensitivity, and overall signal quality. Common electrode materials include silver/silver chloride, which is widely respected for its low noise and high reliability. Gold and conductive polymers are also becoming popular due to their beneficial properties.
Each material offers distinct advantages:
- Silver/Silver Chloride: Highly conductive, stable, and cost-effective.
- Gold: Offers enhanced biocompatibility and corrosion resistance.
- Conductive Polymers: Provide flexibility, reducing discomfort during long-use periods.
The deterioration of electrodes can lead to poor signal quality. Thus, researchers and clinicians must ensure proper calibration and maintenance of electrode materials. In summary, selecting appropriate materials is essential to enhance the performance and longevity of EEG caps.
Cap Design and Configuration
The design and configuration of EEG caps are vital for ensuring optimal electrode placement on the scalp. The cap must fit snugly yet comfortably to prevent motion artifacts that can distort data. This aspect is particularly important for studies requiring prolonged wear or during subject movement.
EEG caps are usually constructed from flexible, breathable fabrics that allow for easy adjustments. Proper design facilitates a wide adaptability, allowing a single cap to fit various head shapes and sizes. The configuration often follows established systems like the International 10-20 system, which ensures standardized spacing between electrodes, enhancing the comparability of results across different studies.
When configuring the cap, the considerations include:
- Electrode Density: More electrodes can capture finer details of brain activity, but they also complicate the setup and analysis.
- Placement Guidelines: Adhering to the 10-20 system ensures consistent data collection methods.
- Comfort and Usability: Tailoring designs that accommodate both users and subjects fosters better compliance during data collection.
Types of EEG Electrodes
Electroencephalography (EEG) plays a critical role in brain activity measurement. The electrodes used in EEG caps are essential for accurate and reliable data capture. Different types of EEG electrodes provide various benefits and applications, which is crucial for both clinical and research settings.
Wet Electrodes
Wet electrodes use conductive gel or paste to facilitate better electrical contact with the scalp. This type enhances the quality of the signal collected from the brain due to lower impedance.
- Benefits: Wet electrodes are often favored in clinical settings because they produce high-quality recordings. The conductive medium significantly reduces noise, resulting in a better representation of cerebral electrical activity.
- Considerations: However, they require preparation time and can be messy, which might not be suitable for all environments. Proper skin preparation is necessary before application to achieve optimal performance.
In applications like sleep studies, their effectiveness is noticeable. The detailed signal acquisition is often indispensable for diagnosing conditions like epilepsy.
Dry Electrodes
Dry electrodes are becoming increasingly popular, especially for wearable EEG technology. They employ materials that do not need conductive gel, thus making them very user-friendly.
- Benefits: These electrodes significantly enhance convenience and ease of use. Users do not have to go through messy procedures, which can encourage wider use outside of traditional lab settings. They are also more adaptable, suitable for various environments and demographics.
- Considerations: Signal quality may not match that of wet electrodes, particularly when high fidelity recording is necessary. This can limit applications in critical clinical settings where precision is essential.
The development of dry electrode technology signifies a notable shift towards portable EEG monitoring, allowing research and diagnostics in everyday settings.
Flexible and Miniaturized Electrodes
Flexible and miniaturized electrodes represent the latest trends in EEG technology. These electrodes are designed to conform to the contours of the scalp. Their design aims to minimize discomfort during longer monitoring sessions.
- Benefits: The major advantage of this electrode type is comfort and adaptability. They can also be applied to more challenging areas of the scalp that standard electrodes cannot effectively register, thus capturing a wider range of brain activity.
- Considerations: The technology is still evolving; as such, consistent data integrity can vary. Additionally, they might struggle with attachment in environments where movement is involved.
Flexible and miniaturized electrodes open new pathways for continuous brain monitoring in various applications, including cognitive studies and brain-computer interfaces, making them a focal point in current research.
"The choice of EEG electrode type can significantly affect signal quality and data integrity, impacting overall research and clinical outcomes."
EEG Cap Configuration Standards
EEG cap configuration standards are critical in ensuring the consistent and accurate placement of electrodes during electroencephalography procedures. The arrangement and positioning of these electrodes directly influence the quality of the data collected. By adhering to established standards, researchers and clinicians can minimize variabilities that may affect signal interpretation. Accurate placement is essential for reliable readings, particularly when diagnosing conditions such as epilepsy or conducting cognitive studies.
One of the most recognized frameworks in EEG cap configuration is the International 10-20 System, which serves as a guideline for electrode placement based on head measurements. This standardized approach improves reproducibility across different studies and clinical settings, fostering comparison of results among various research and clinical endeavors.
International 10-20 System
The International 10-20 System is a tried-and-true method for electrode placement in EEG studies, providing a simple yet effective reference system. This method divides the head into a standardized grid that is based on specific distances between anatomical landmarks. The term '10-20' refers to the fact that electrodes are placed at either 10% or 20% intervals of the total distance between those landmarks.
Utilizing the 10-20 system ensures that electrodes cover the entire scalp uniformly, allowing for optimal coverage of brain regions. This comprehensive mapping is essential for capturing brain activity across various areas, leading to a more robust interpretation of data. The methodology allows for the precise correlation of brain activity with specific regions of interest, aiding in both clinical diagnosis and research applications.
Variations in Placement Techniques
Although the 10-20 system is widely accepted, variations in electrode placement techniques do exist. These differences may arise from specific clinical needs or regional variations in protocol. For instance, some researchers might employ the 10-10 or 10-5 systems, which provide denser electrode placement by reducing the interval between electrodes. This can enhance spatial resolution, particularly beneficial in epilepsy monitoring.
Another notable approach involves using individualized placement based on a subject's anatomical features. This technique may enhance data quality for studies focusing on particular brain regions. However, such methods also require careful consideration and skilled practitioners to accurately adjust placements.
As EEG technology advances, integration of image data from MRI or CT scans is becoming more common. This allows for better alignment of electrode positions on the individual’s specific brain anatomy, increasing the precision of signal acquisition. While variations offer benefits, they can also complicate the standardization necessary for baseline comparisons.
Overall, EEG cap configuration standards play an essential role in electroencephalography practices. They enable a structured approach to electrode placement, fundamentally influencing the reliability and validity of resulting data. Understanding these standards equips users with the knowledge necessary to optimize EEG systems for both clinical and research applications.
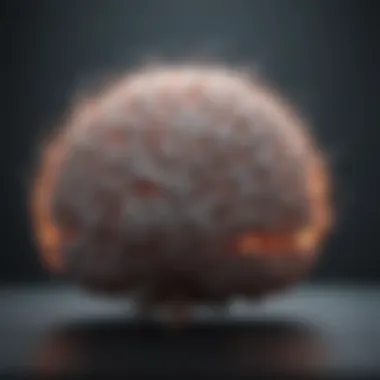
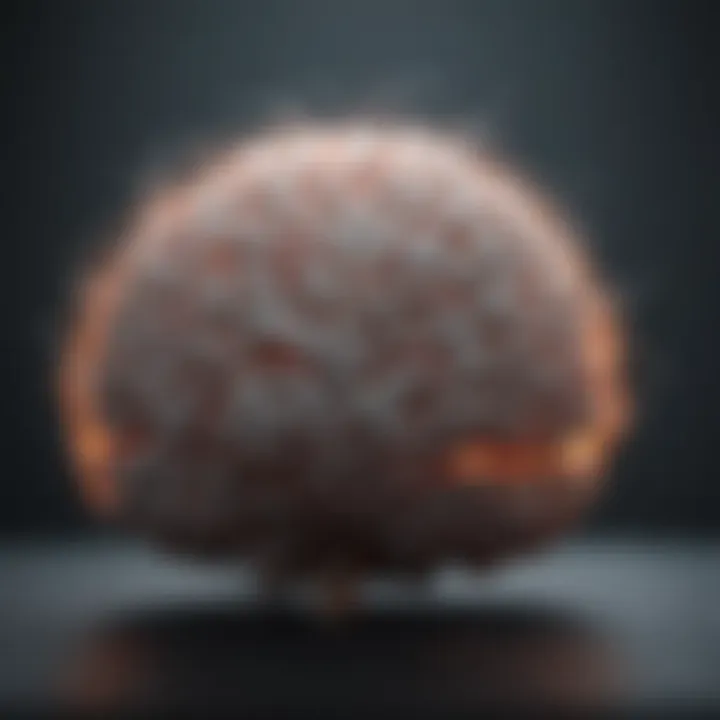
By recognizing the importance of consistent EEG cap configuration, researchers and clinicians can significantly improve the quality of data collected, enhancing the ability to access complex brain functions.
Signal Acquisition and Processing
Signal acquisition and processing forms a critical part of the functionality of EEG cap electrodes. This section outlines the importance of accurately capturing electrical signals generated by the brain, the methods utilized in processing these signals, and the implications for interpretation and analysis. Accurate signal acquisition directly impacts the quality of data obtained, and understanding this aspect helps elucidate the complexities involved in neurophysiological research.
Electrical Properties of the Brain
The brain's electrical activity is primarily based on the biophysics of neuronal communication. Neurons communicate via action potentials and local field potentials, generating small electrical signals that can be measured on the surface of the scalp. Understanding these electrical properties is essential for EEG technology, as the resulting measurements are reliant on the impedance associated with these signals. Factors such as skin, skull, and cerebrospinal fluid resistance all contribute to the characteristics of EEG signals.
- Action Potentials: When a neuron fires, there is a rapid change in ion concentrations across the membrane, resulting in a spike potential that can propagate along the neuron.
- Local Field Potentials: These reflect the summed electrical activity of a group of neurons within close proximity, offering insight into brain function on a larger scale.
The brain's intrinsic electrical properties must be considered in EEG cap design and electrode placement to ensure successful signal acquisition. Each electrode's configuration must account for these elements, as miscalculations can lead to poor signal quality that hinders analysis.
Amplification and Filtering
Once the electrical signals are acquired, amplification and filtering are crucial to enhance their quality for further analysis. EEG signals are weak, typically ranging from microvolts to millivolts, necessitating amplification to make them usable. The process involves several key aspects:
- Amplification: The initial step involves increasing the amplitude of the signal to a level where it can be effectively analyzed. High-quality operational amplifiers are employed to ensure minimal noise and distortion.
- Filtering: Signals are often contaminated with noise and artifacts. This necessitates the use of filters to isolate the frequency ranges of interest. Low-pass filters are used to remove high-frequency noise, while high-pass filters eliminate low-frequency drift.
Including good filtering techniques prevents misinterpretation of neural signals, thus enhancing data integrity. The selection of appropriate filter settings ensures that the resulting output accurately represents brain activity, facilitating better insights into neural mechanisms.
"Without proper amplification and filtering, the integrity of the EEG data would be compromised, leading to potential misinterpretations in clinical or research settings."
In summary, both signal acquisition and processing are foundational in EEG electrode functionality. Understanding the brain's electrical properties allows researchers to develop systems that maximize data quality, while effective amplification and filtering techniques ensure that the meaningful aspects of neural signals are preserved and utilized.
Applications of EEG Cap Electrodes
The applications of EEG cap electrodes stand as a testament to their significance in both clinical settings and research environments. As the understanding of brain activity evolves, the demand for effective and precise methods of monitoring those activities has grown. EEG cap electrodes are at the forefront of this demand, providing invaluable data that can lead to critical insights in various fields. Their versatility allows for a wide range of uses, thus playing an essential role in enhancing our understanding of neurological conditions and cognitive processes.
Clinical Uses
Epilepsy Monitoring
Epilepsy monitoring represents a core application of EEG cap electrodes. This practice involves tracking brain activity to identify and analyze seizures. The electrodes capture electrical signals in real-time, making insights into seizure patterns possible. The main reason for its popularity lies in its effectiveness and the ability to provide immediate feedback on patient condition.
One unique feature of epilepsy monitoring is its capability for long-term evaluation. This allows clinicians to assess the frequency and severity of seizures over days or weeks. The main advantadge is that it supports tailored treatment strategies based on individual patient needs. However, it requires a controlled setting, which sometimes adds complexity to the patient’s experience.
Sleep Studies
Sleep studies utilize EEG cap electrodes to investigate sleep disorders. This is crucial in understanding the stages of sleep and diagnosing conditions like sleep apnea or insomnia. The electrodes facilitate detailed assessments of brainwaves, which helps in constructing a complete picture of the subject's sleep cycle. Such studies have become increasingly crucial in clinical environments.
A key characteristic of sleep studies is their ability to monitor brain activity in a non-invasive manner. This makes them a beneficial choice for practitioners. Additionally, the data from these studies can guide treatment methods effectively. On the downside, participants may find themselves in uncomfortable settings, which could impact their natural sleep behavior.
Neurofeedback
Neurofeedback, another significant application of EEG cap electrodes, involves training individuals to alter their brain activity. This technique is gaining traction due to its non-invasive nature and potential mental health benefits. It allows users to gain awareness and control over their mental states and has applications in conditions like anxiety or ADHD.
The key characteristics of neurofeedback revolve around its personalized approach. Each session can be adjusted to suit individual needs, which addresses specific mental health challenges effectively. Its interactive nature adds to its appeal; however, outcomes can vary widely among individuals, which may limit its consistent effectiveness.
Research Applications
Cognitive Studies
Cognitive studies leverage EEG cap electrodes to explore brain activity related to various cognitive functions. These studies are crucial in unlocking the complexities of processes such as attention, memory, and decision-making. EEG technology is particularly favored in these investigations due to its high temporal resolution.
One of the main benefits is the real-time observation capability, allowing for a deeper understanding of cognitive processes as they occur. Researchers can analyze how different stimuli influence brain activity. A challenge lies in interpreting results, as data can be affected by numerous variables.
Brain-Computer Interfaces
Brain-computer interfaces (BCIs) represent an innovative field relying on EEG cap electrodes for movement control. Through these interfaces, users can control devices using their thoughts, significantly benefiting individuals with disabilities. The potential implications for mobility and independence are profound, making BCIs a popular area of research.
The unique feature of BCIs is their promising application in assistive technologies. This gives them a real-world impact that goes beyond theoretical exploration. However, the technology still faces limitations in terms of signal clarity, making real-time control challenging in certain scenarios.
Neuroscience Investigations
Neuroscience investigations benefit extensively from EEG cap electrodes as they expand knowledge regarding brain function in health and disease. Through these investigations, researchers can study everything from neural connectivity to the impact of external stimuli on brain circuitry. The capacity to analyze brain function in a detailed manner is invaluable in bridging the gaps in current understanding.
A significant advantage of neuroscience investigations is the ability to track changes over time. This longitudinal analysis provides insights into neuroplasticity and development. Nevertheless, with complex data comes challenges in analysis and interpretation, requiring advanced tools and expertise.
Overall, EEG cap electrodes present a versatile tool for a range of applications, from clinical monitoring to research investigations, enhancing our understanding of brain function and opening new avenues for advancements in neuroscience.
Challenges in EEG Cap Use
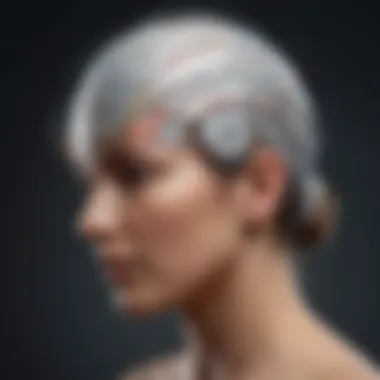
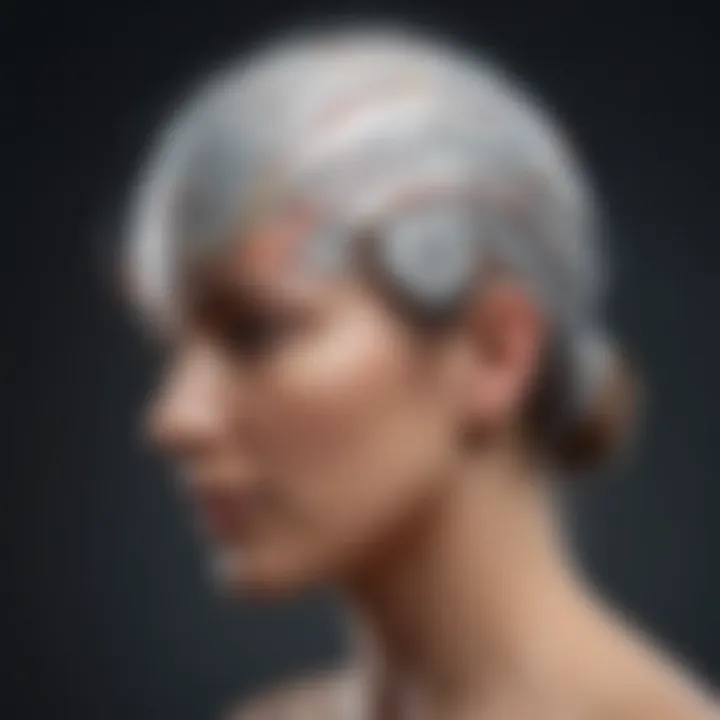
EEG cap electrodes have advanced significantly in design and functionality. However, challenges still arise in their usage. These challenges profoundly affect the quality of data collected during neurophysiological studies. Accurate electrode placement and artifact management are vital elements in ensuring the efficacy of EEG recordings. Failure to address these concerns can lead to misleading results, impacting both research and clinical outcomes.
Electrode Placement Accuracy
Electrode placement accuracy is crucial for obtaining reliable EEG data. The International 10-20 system provides a standardized framework for placing electrodes on the scalp. However, variations in individual head anatomy can complicate this. Factors such as hair type, head shape, and even the movement of subjects can influence electrode positioning. The precision of placement directly affects the signal quality, determining the effectiveness of the EEG in detecting brain activity.
Multiple techniques and tools, such as anatomical landmarks and imaging, can help improve accuracy. In clinical settings, trained personnel often perform electrode placement to ensure consistency and minimize errors. In research, automated systems and advanced imaging techniques are being developed to assist in achieving better precision.
Artifact Management
Artifacts in EEG recordings can arise from various sources, including muscle activity, eye movements, and external electrical noise. This interference can obscure the true signals that represent brain activity. Proper artifact management is essential to obtain clear and interpretable data.
To mitigate these effects, researchers and clinicians can employ several strategies:
- Pre-processing techniques: Software tools can identify and filter out specific types of artifacts before analysis.
- Controlled environments: Recording in settings that minimize external noise helps reduce interference.
- Participant instruction: Educating subjects on minimizing movements during recordings can reduce physiological artifacts.
“Addressing challenges in electrode placement and artifact management is essential for the integrity of EEG data.”
Technological Advances in EEG Electrodes
The world of EEG technology continuously evolves, adapting to new scientific discoveries and societal needs. Advancements in the design and functionality of EEG electrodes have changed how researchers and clinicians approach neurophysiological studies. This section looks deeply into the significance of these technological strides, focusing on specific innovations and their impact.
Wearable EEG Technology
Wearable EEG technology represents a transformative shift in brain activity monitoring. Traditional EEG setups often require extensive equipment and laboratory settings, which can limit accessibility and convenience. Wearable devices offer a solution by allowing users to gather EEG data in real-life environments. These compact and often less cumbersome systems empower participants to engage in daily activities while recording brain activity and other neurological data.
Key advantages of wearable EEG technology include:
- Enhanced Mobility: Users can move freely, facilitating studies in naturalistic settings.
- Remote Monitoring: Healthcare providers can track patient health from a distance, improving treatment response time.
- User Engagement: Participants are more likely to remain engaged in studies when they do not have bulky equipment.
However, developing accurate and reliable wearable EEG devices comes with challenges. These include ensuring data quality, reducing environmental noise, and maintaining user-friendliness. Innovators must balance these tasks while keeping the technology affordable and accessible.
Integration with Other Modalities
Another significant advancement in EEG technology lies in its integration with other modalities, such as functional magnetic resonance imaging (fMRI) or eye-tracking systems. By combining these methods, researchers can achieve a multi-dimensional view of brain activity. This integration allows for a comprehensive mapping of neural circuits and offers insights that standalone EEG cannot provide.
For instance, fMRI can identify where brain activity occurs, while EEG reveals when it happens. When used together, they provide valuable temporal and spatial information. Additional benefits include:
- Broader Data Acquisition: Combining modalities captures more comprehensive datasets, allowing for deeper analysis of complex brain functions.
- Cross-Validation: Results from one modality can validate findings in another, enhancing the credibility of collected data.
- New Research Horizons: This fusion opens new avenues for exploring cognitive processes, potentially leading to breakthroughs in understanding brain disorders.
Future Perspectives on EEG Cap Electrodes
The evolution of EEG cap electrodes is poised to continue as advancements in technology and methodology progress. Understanding future perspectives in this area is critical because it informs both researchers and clinicians about new possibilities for brain activity monitoring. The influence of EEG technology extends beyond traditional clinical uses, reaching into diverse fields such as cognitive neuroscience and mental health interventions. This section explores emerging research areas and the potential impacts that new developments in EEG cap electrodes may have on neuroscience.
Emerging Research Areas
The landscape of EEG cap electrodes is rapidly evolving due to innovative research initiatives. Several significant areas are being explored:
- Real-Time Data Processing: Advances in computational power enable more efficient real-time processing of EEG data. This could improve the accuracy of interpreting brain signals instantly, allowing for immediate feedback in clinical settings.
- Machine Learning Integration: Machine learning algorithms are becoming more sophisticated in EEG signal analysis. By leveraging big data, researchers can uncover patterns in brain activity that were previously unnoticed, facilitating breakthroughs in understanding neurological conditions.
- Wireless Technology: The shift towards wireless EEG systems allows for more significant mobility. This innovation will enable researchers to conduct studies in more diverse environments and contexts, enhancing the ecological validity of their findings.
- Personalized EEG Cap Design: Future designs may include personalized EEG caps that conform to individual head shapes, resulting in better electrode placement and data accuracy.
The relevance of these research areas cannot be understated. Each innovation presents new pathways for enhancing our understanding of brain function and addressing specific neurological challenges.
Potential Impacts on Neuroscience
The projected advancements in EEG cap electrodes could lead to numerous transformative impacts in neuroscience:
- Improved Diagnostic Tools: As the accuracy of EEG data interpretation increases, clinicians may be able to diagnose conditions like epilepsy and sleep disorders more precisely. This can lead to tailored therapies that improve patient outcomes.
- Innovative Treatment Modalities: The intersection of EEG technology with neurofeedback and brain-computer interfaces can produce novel treatment approaches. These methods allow patients to gain greater control over their brain activity, potentially aiding in rehabilitation for conditions like stroke or serious mental health issues.
- Enhanced Research Capabilities: The future of EEG cap technology will create new opportunities for basic neuroscience research. With improved data quality, researchers will have the ability to investigate complex cognitive processes underlying behaviors and mental functions.
- Interdisciplinary Applications: The integration of EEG technology with fields like artificial intelligence or wearable health technology may foster innovative applications beyond conventional neuroscience. This includes assessing cognitive load during tasks or tracking mental states in everyday activities.
"The future of EEG technology promises to redefine how we approach brain research and patient care, unlocking invaluable insights into neural mechanisms."
Overall, the future perspectives on EEG cap electrodes highlight the significance of ongoing innovation within this field. The potential to impact clinical practices and research methodologies makes it a crucial area for continued exploration. As these technologies develop, they will pave the way for a deeper understanding of the complex workings of the human brain.
Ending
In the realm of neurophysiology, EEG cap electrodes play a vital role. This article highlights their design, functionality, and relevance in both clinical and research settings. Understanding the intricacies of EEG cap electrodes is essential for students, researchers, and professionals in neuroscience. With the information discussed, readers can appreciate how these components capture brain activity effectively.
Addressing the complexities around EEG practices helps to improve the accuracy of brain signal interpretation. Furthermore, being aware of current challenges, such as electrode placement accuracy and artifact management, can enhance the overall effectiveness of EEG studies. Therefore, the continual development and refinement of EEG technology are crucial.
Summary of Key Points
- EEG cap electrodes are integral to capturing brain activity.
- The design and materials of the electrodes influence data quality.
- Various types, including wet and dry electrodes, offer unique benefits.
- The 10-20 system standardizes electrode placement, ensuring consistency.
- Technological advancements shape future EEG applications in research and clinical practice.
Final Thoughts
The importance of EEG cap electrodes cannot be overstated. As neuroscience evolves, these electrodes will continue to adapt and improve. Future research may lead to innovative designs that enhance signal acquisition and processing capabilities. Understanding the existing technology will prepare future generations to further this work in meaningful ways. Continuous exploration in this field remains necessary for unlocking the complexities of the brain.
"The intersection of technology and neuroscience holds immense potential for breakthroughs that were once deemed unimaginable."
Further study can lead to deeper insights into cognitive functions and neural disorders, bringing potential hope for new treatment methods. The future of EEG technology appears promising, and it's an area well worth monitoring.