Understanding Distributed Energy: A Comprehensive Overview
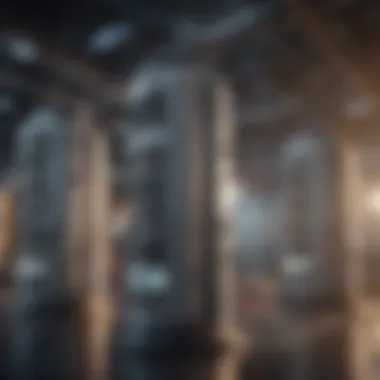
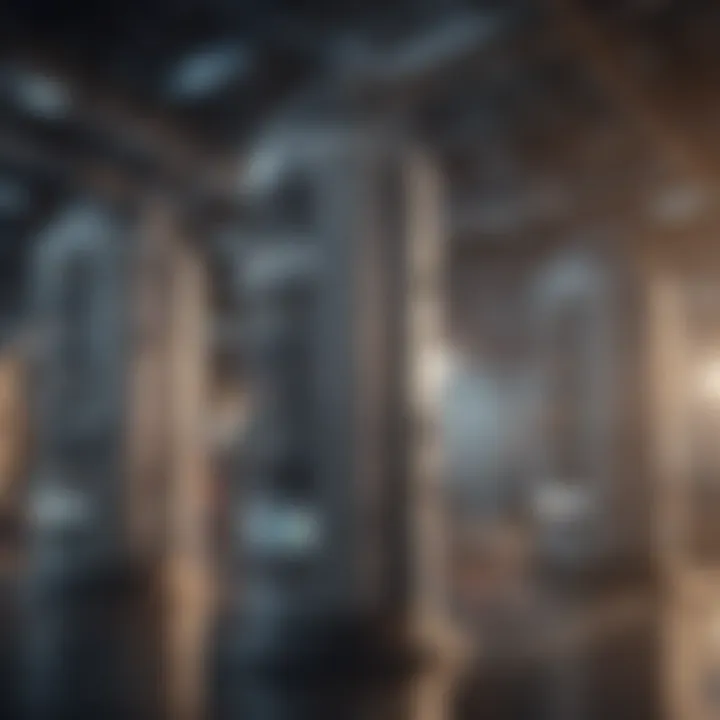
Intro
The discourse surrounding energy generation and consumption is undergoing a significant transformation. Traditional centralized systems are increasingly complemented or even supplanted by distributed energy systems. These systems enable localized energy production, often leveraging renewable sources, which aligns with broader sustainability goals. Understanding this intricate landscape entails more than merely defining terms; it requires a deep dive into the structure, advantages, challenges, and implications of distributed energy.
As energy demands evolve, so does the need for flexibility and innovation in production methods. Distributed energy not only enhances energy security but also empowers communities and individuals. By exploring its mechanisms, technologies, and future directions, we can appreciate the potential it holds for fostering a sustainable energy future.
This article will present a structured examination of distributed energy, highlighting critical definitions and system variations. Furthermore, it will address the technological advancements that drive this trend, the regulatory frameworks that govern it, and the challenges that stakeholders must navigate. A careful analysis aims to provide a well-rounded understanding of how distributed energy fits into the current energy paradigm.
Prelude to Distributed Energy
As the global energy landscape continues to evolve, understanding distributed energy becomes increasingly essential. Distributed energy refers to the small-scale generation and storage of energy close to the point of use, as opposed to centralized power sources. This shift is significant due to the growing demand for cleaner, more reliable, and efficient energy solutions.
The importance of this topic lies not only in its potential to reduce reliance on fossil fuels but also in its capacity to empower local communities. By harnessing resources like solar, wind, and biomass, distributed energy enhances energy security and promotes local economic growth. Furthermore, the developments in technology underpinning these systems, such as smart grids and energy storage solutions, open an avenue for innovation that can reshape how we think about energy consumption.
Defining Distributed Energy
Defining distributed energy entails understanding its various components and functionalities. At its core, distributed energy can be characterized by its localized production methods and its ability to operate independently or in synergy with the main power grid. Unlike traditional energy systems, which rely on large power plants to generate electricity, distributed energy systems include sources such as rooftop solar panels, community wind turbines, and biomass digesters.
In practice, distributed energy enhances resilience against power outages and disturbances within the centralized grid. This resilience is paramount in an age where natural disasters and cyber threats are on the rise. Thus, distributed energy is not just a passing trend; it represents a fundamental shift in the energy hierarchy, promoting a more balanced approach to energy generation and consumption.
Historical Context
To better understand the current state of distributed energy, one must look at its historical roots. The concept of decentralized energy production is not entirely new. Historically, people have harnessed local energy sources for centuries; from firewood to water mills, communities relied on what was nearby. However, the advent of the industrial revolution brought a significant shift towards centralized energy production, leading to the construction of large power generation facilities.
This shift resulted in several drawbacks, including long transmission lines, energy losses, and increased greenhouse gas emissions. In the late 20th century, alongside escalating environmental concerns, nostalgia for localized production began to resurface. The 1970s energy crises spurred interest in alternative sources, leading to the modern distributed energy movement. Today, technological advancements, policy support, and a commitment to sustainability help shape the renaissance of distributed energy.
"The history of energy production is a tale of centralization and decentralization, revealing our evolving relationship with resources."
Types of Distributed Energy Systems
Understanding the various types of distributed energy systems is crucial. This knowledge provides insights into how energy can be generated closer to the point of use, reducing transmission losses and improving efficiency. Each system type has its own unique characteristics, benefits, and challenges. This section will explore five key distributed energy systems: solar, wind, biomass, hydropower, and geothermal systems.
Solar Energy Systems
Solar energy systems capture sunlight and convert it into electricity, either through photovoltaic panels or concentrated solar power. They are among the most widely adopted distributed energy sources.
The benefits of solar energy systems include:
- Renewable source: Solar energy is abundant and sustainable.
- Low operational costs: Once installed, solar systems have minimal maintenance and operating costs.
- Energy independence: Users may reduce reliance on grid energy.
However, there are certain considerations:
- Intermittency: Solar generation is not constant, as it depends on weather and daylight.
- Space requirements: Solar panels require sufficient roof or land space for installation.
Wind Energy Systems
Wind energy systems utilize wind turbines to convert wind kinetic energy into electrical energy. They are efficient at various scales, from small residential units to large wind farms.
The advantages of wind energy systems include:
- Clean energy: Wind generation emits no greenhouse gases.
- Scalability: Systems can be tailored to meet diverse energy needs, whether small or large scale.
On the downside, potential challenges include:
- Intermittent availability: Wind resources can be variable.
- Noise and aesthetics: Wind turbines may be considered visually unappealing and can generate noise.
Biomass Energy Systems
Biomass energy systems convert organic materials into energy. This includes wood, agricultural residues, and waste. Biomass serves as a renewable resource, providing a consistent energy source.
Key benefits of biomass systems are:
- Waste reduction: Utilizes waste products that would otherwise contribute to landfills.
- Versatile applications: Biomass can be turned into heat, electricity, or biofuels.
However, some considerations are:
- Land use: Growing biomass resources may compete with food production.
- Emissions: Burning biomass can release pollutants if not managed properly.
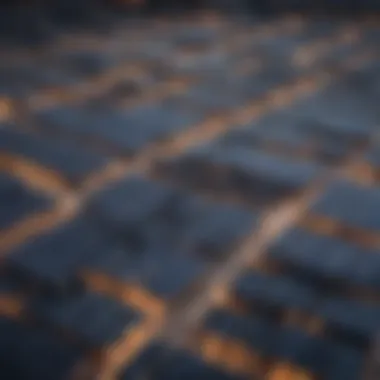
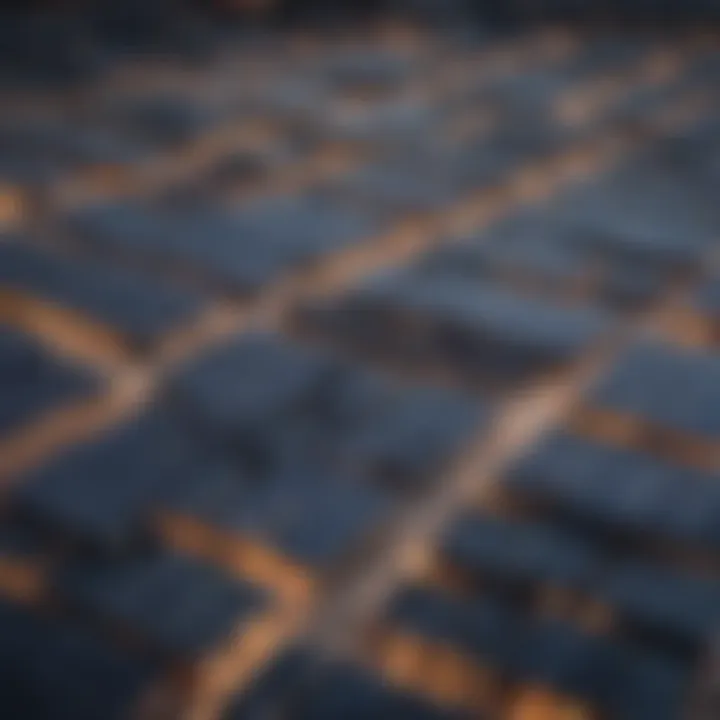
Hydropower Systems
Hydropower systems harness water flow to generate electricity. While large-scale dams are common, small-scale run-of-the-river projects represent a form of distributed energy.
The benefits of hydropower include:
- Consistency: Hydropower can provide reliable baseload electricity.
- Storage capability: Large reservoirs can store water and manage supply during peak demand.
Challenges include:
- Environmental impact: Dam construction can disrupt local ecosystems.
- Geographic limitations: Not all regions have suitable water resources.
Geothermal Systems
Geothermal systems use heat from the Earth's interior to produce energy. They can power entire communities or serve individual buildings.
The advantages of geothermal systems include:
- Sustainability: Offers a consistent energy source, virtually free from seasonal fluctuations.
- Low emissions: Emits little to no greenhouse gases compared to fossil fuels.
However, some key points to consider are:
- Location dependency: Access to geothermal resources is limited to certain geographical areas.
- Initial costs: Drilling and installation can be expensive.
In summary, understanding various types of distributed energy systems enables stakeholders to make informed decisions about energy sourcing and implementation. Each system holds unique benefits and challenges that must be navigated to optimize energy production and sustainability.
"Distributed energy systems can play a pivotal role in achieving energy independence and sustainability. Each type contributes differently, and understanding these distinctions is essential."
Technological Innovations in Distributed Energy
Technological innovations are at the heart of the evolution of distributed energy systems. They enhance the efficiency and reliability of energy generation and consumption while creating opportunities for new energy management practices. As the global demand for clean energy solutions grows, the implementation of these innovations becomes essential for transitioning to more sustainable energy frameworks. This section examines three pivotal advancements: smart grids, energy storage solutions, and microgrids. Each of these elements contributes significantly to the modernization of energy systems.
Smart Grids and Energy Management
Smart grids represent a transformative advancement in energy distribution. They utilize digital communications technology to monitor and manage the transport of electricity from all generation sources to meet the varying electricity demands of end users. The integration of smart meters and sensors helps utility companies gain real-time data regarding usage patterns, enabling them to adjust supplies accordingly. This technology fosters a more resilient and efficient energy system.
Benefits of smart grids include:
- Improved Reliability: Smart grids reduce the likelihood of power outages, as they can detect issues quickly and reroute power flows.
- Integrated Renewable Sources: They facilitate the incorporation of renewable energy sources, allowing for increased dependence on clean energy.
- Consumer Participation: Consumers can track their energy usage more closely and engage with demand response programs. This encourages energy conservation.
Overall, smart grids enhance operational efficiency and can significantly reduce the carbon footprint of energy systems.
Energy Storage Solutions
Energy storage technologies are critical for managing the intermittent nature of renewable energy sources like solar and wind. They allow excess energy generated during peak production times to be stored and used during periods of high demand or low generation. This not only aids in optimizing energy supply but also stabilizes the grid.
Common energy storage solutions include:
- Batteries: Lithium-ion batteries are commonly used for both residential and industrial applications. They are efficient and have relatively low maintenance costs.
- Pump hydro storage: This method uses gravitational potential energy, involving pumping water to a higher elevation during low demand and releasing it through turbines for power generation when needed.
- Flywheels: These devices store energy through rotational motion and are suitable for short-duration energy needs.
Energy storage systems can enhance the flexibility and reliability of distributed energy setups. They play a pivotal role in addressing the challenge of supply and demand imbalances.
Microgrids Explained
Microgrids are localized grids that can operate independently or in conjunction with the traditional grid. They enhance energy resilience by allowing local areas to generate, store, and consume power seamlessly. Microgrids utilize various energy sources, including renewables and conventional fuels, on a smaller scale.
The benefits of microgrids include:
- Resilience: They can function autonomously during grid failures, ensuring continuous power supply to critical facilities such as hospitals.
- Localized Energy Production: This reduces energy transmission losses and reliance on long-distance electricity transport.
- Sustainability: Microgrids promote the use of renewable energy resources, reducing greenhouse gas emissions.
"Microgrids offer a vision for a more resilient and sustainable energy future, enabling communities to take control of their energy needs."
Regulatory and Policy Frameworks
The regulatory and policy frameworks surrounding distributed energy are essential for fostering an environment where such systems can thrive. These frameworks shape how distributed energy systems are implemented, what technologies are adopted, and the extent to which these systems can contribute to broader energy goals. Effective regulations help balance the needs of various stakeholders, including consumers, utility companies, and government entities. As the energy landscape becomes increasingly decentralized, the importance of clear policies and incentives cannot be overstated.
Government Incentives for Distributed Energy
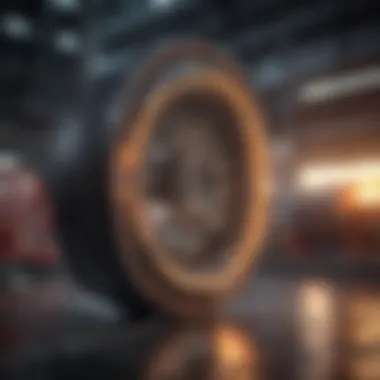
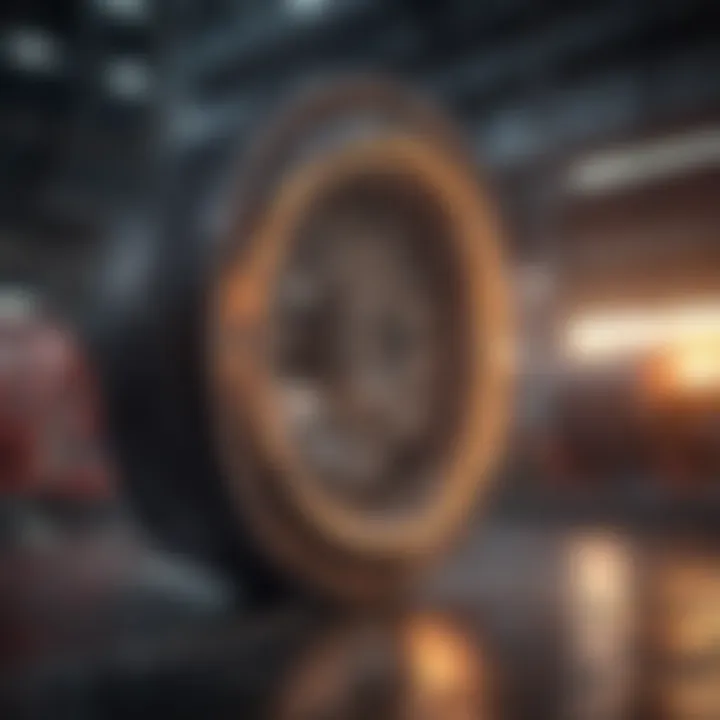
Government incentives are crucial for the growth of distributed energy. These can take various forms, such as tax credits, grants, and rebates designed to lower the initial cost of installing systems like solar panels or wind turbines. They also provide a supportive structure that encourages individuals and businesses to invest in renewable energy technologies. Some notable examples of government incentives include:
- Investment Tax Credit (ITC): This allows taxpayers to deduct a significant percentage of the energy system installation costs from their federal taxes.
- Production Tax Credit (PTC): This offers financial benefits based on the amount of renewable energy produced, making it a direct economic incentive for generators.
- State-specific incentives: Many states enact their own programs to support distributed energy, which can include renewable portfolio standards (RPS), feed-in tariffs, and net metering policies.
These programs promote innovation and sustainability within local economies, increasing opportunities for job creation in the energy sector. They also help reduce greenhouse gas emissions and make renewable energy more accessible to a larger segment of the population.
Challenges in Regulation
Despite the advantages of distributed energy, several challenges hinder effective regulation. Some of the prominent issues include:
- Complexity of Existing Regulations: Navigating the existing regulations often proves difficult for new entrants in the distributed energy market. This complexity can discourage investment and innovation.
- Compatibility with Traditional Utility Structures: Many regulatory frameworks are designed around centralized energy systems. Transitioning to a model that supports distributed energy requires significant amendments to existing policies.
- Managing the Integration of Diverse Technologies: The rapid emergence of new technologies leads to a patchwork of regulations that often does not account for the interoperability of systems. Each new tech can face delays in receiving regulatory approval, which stifles progress.
Governments and policymakers must recognize these challenges to develop more streamlined and coherent frameworks that effectively support distributed energy adoption while maintaining grid reliability and ensuring consumer protections. As the momentum toward renewable energy continues to grow, adapting regulatory frameworks will play a critical role in shaping the future of energy production and consumption.
"Regulatory clarity and supportive policies are fundamental to the successful integration of distributed energy systems into our energy landscape."
Sustainability and Environmental Impact
Sustainability and environmental impact are critical themes in the context of distributed energy systems. As the world faces pressing environmental challenges, including climate change and resource depletion, understanding the environmental implications of energy production is more important than ever. Distributed energy systems—such as solar panels, wind turbines, and biomass generators—offer potential solutions that align with sustainability goals. Their localized nature allows for greater energy independence and reduced reliance on fossil fuels, which is crucial in mitigating the climate crisis.
From a sustainability perspective, these systems contribute to reduced greenhouse gas emissions and promote the use of renewable resources. As more individuals and businesses implement distributed energy solutions, the collective impact can lead to significant reductions in a region's overall carbon footprint. Moreover, many distributed energy technologies can be deployed quickly and with lower upfront costs compared to large-scale conventional power plants. This flexibility in implementation not only supports energy resilience but also enhances economic viability.
Reducing Carbon Footprint
Reducing the carbon footprint is a paramount benefit of distributed energy systems. The carbon footprint refers to the total amount of greenhouse gases emitted directly or indirectly by human activities. Traditional fossil fuel-based energy generation is a major contributor to these emissions. In contrast, distributed energy systems provide cleaner alternatives.
For example, solar energy systems capture sunlight to generate electricity without producing emissions during operation. Similarly, wind energy systems harness the wind to power turbines, creating clean energy. When people utilize these renewable resources, they significantly lower their carbon emissions.
Implementing distributed energy technologies on a large scale speaks directly to the goals of international climate agreements, like the Paris Accord. By transitioning to these cleaner forms of energy, countries can create tangible pathways towards their emissions reduction targets.
- Key Benefits of Reducing Carbon Footprint:
- Lower greenhouse gas emissions
- Compliance with environmental policies
- Enhanced public health due to cleaner air
"The shift towards localized energy generation has the potential to drastically alter our environmental landscape, making a positive impact on global carbon levels."
Effects on Local Ecosystems
The deployment of distributed energy systems can markedly affect local ecosystems. While these systems offer substantial environmental benefits, their installation and operation must be carefully considered to minimize negative impacts.
For instance, solar farms can disrupt local habitats if not correctly placed. However, thoughtful planning and site selection can mitigate these effects. In many cases, solar panels are now placed on rooftops or degraded lands, thereby preserving natural habitats. Moreover, wind turbines can also pose risks to local wildlife, particularly birds and bats. The placement of turbines in migratory paths requires careful assessment and planning to minimize harm.
On the positive side, distributed energy systems can also promote biodiversity. For example, agrophotovoltaics, where solar farms are combined with agricultural activities, can enhance ecosystem services by supporting local flora and fauna. This dual use of land maximizes efficiency and helps maintain the ecological balance.
- Considerations for Effects on Local Ecosystems:
- Careful site assessment and planning
- Implementation of wildlife protection measures
- Integration of ecosystems into renewable energy designs
As we look towards a future increasingly reliant on renewable energy, the environmental impact of these technologies must remain at the forefront of energy discussions. Understanding both the benefits and challenges associated with distributed energy solutions will be essential for effective policy and sustainability strategies.
Economic Considerations
The economic aspects of distributed energy systems play a crucial role in understanding their viability and impact on the energy sector. Economic considerations encompass a variety of elements, including the financial implications of investment, operational costs, and potential savings for consumers and utilities. As we explore these elements, it becomes clear that distributed energy is not just about environmental sustainability; it is also about creating a robust economic landscape that benefits individuals and communities.
Cost-Benefit Analysis
A thorough cost-benefit analysis is essential to evaluate the economic practicality of distributed energy systems. This analysis compares the upfront investments required for installation, maintenance, and operation against the long-term benefits such as reduced utility bills, government incentives, and enhanced energy independence.
- Initial Costs: Many distributed energy systems, like solar panels or wind turbines, require significant capital for setup. This includes not only the equipment but also installation and grid connection costs.
- Operational Savings: Once established, these systems often produce energy at lower costs compared to traditional power sources. Reduced electricity bills are a significant incentive for homeowners and businesses.
- Incentives and Subsidies: Many governments offer financial incentives for installing renewable energy sources. Tax credits, rebates, and grants can substantially lower the initial investment, making distributed energy more accessible.
- Long-Term Value: Increased energy security and price stability can lead to a more economically resilient energy landscape.
In summary, a comprehensive cost-benefit analysis demonstrates that despite high initial costs, the long-term savings and benefits often outweigh these expenses, making distributed energy systems an attractive option for many.
Job Creation in the Distributed Energy Sector
The distributed energy sector not only addresses energy generation but also significantly contributes to job creation. As communities shift towards decentralized energy resources, numerous employment opportunities arise across various levels.
- Installation and Maintenance: Jobs in installation, maintenance, and repair of renewable energy systems such as solar panels and wind turbines are rapidly increasing. Skilled labor is needed to ensure these systems function effectively.
- Research and Development: With continuous innovations, there is a constant demand for professionals in R&D. Universities and private firms focus on improving efficiency and reducing costs associated with distributed energy.
- Policy and Regulation: As distributed energy becomes more mainstream, roles in regulatory compliance and energy policy are emerging. Professionals are needed to navigate the evolving landscape of energy laws and incentives.
- Community Outreach and Education: Increased interest in distributed energy systems has led to a need for educational programs and community initiatives. Job opportunities exist for educators and outreach coordinators who inform the public about the benefits and workings of these systems.
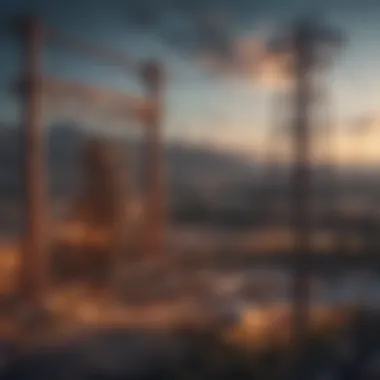
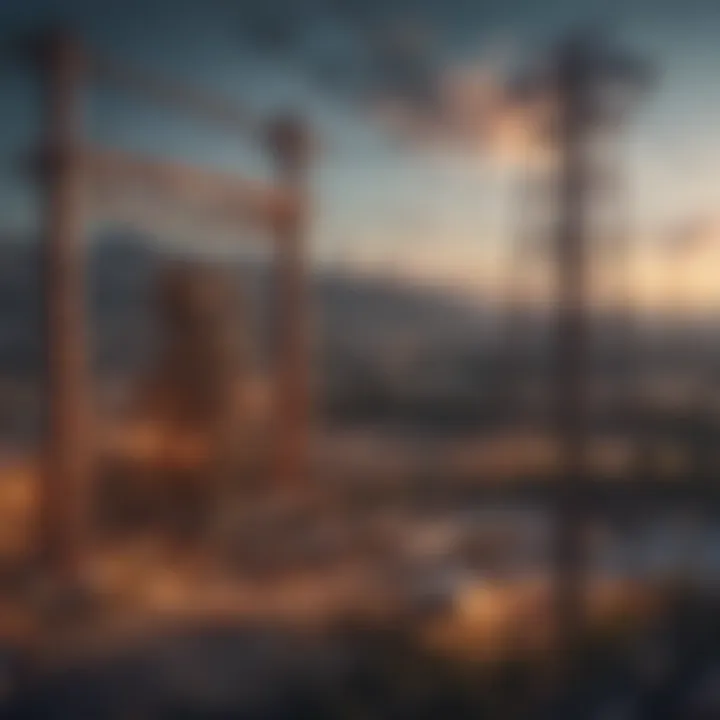
Challenges Facing Distributed Energy Implementation
Understanding the challenges that impede the implementation of distributed energy systems is crucial in today’s energy landscape. These challenges affect not just the feasibility of these systems but also their efficacy in contributing to energy sustainability. As distributed energy becomes increasingly vital in shifting away from traditional energy paradigms, several key elements arise that hinder widespread adoption.
Interconnection Issues
Interconnection refers to the ability of distributed energy resources to connect and operate efficiently with the main power grid. Achieving successful interconnection can face numerous technical and regulatory hurdles.
For example, the standards for grid connection may vary widely from one region to another. Utilities often impose stringent requirements on distributed energy systems, such as those related to safety, power quality, and reliability. This divergence can lead to delays and increase costs for developers and energy producers trying to implement new projects. Furthermore, many grid infrastructures exist that have not been upgraded for compatibility with distributed technologies, thus limiting their efficiency.
- Lack of Standardization: Without standard interconnection protocols, it becomes a daunting task to synchronize various distributed systems within the grid.
- Costs of Infrastructure Upgrades: Upgrades can be financially burdensome, especially for smaller renewable energy projects, thus creating a barrier to entry.
"Successful deployment of distributed systems is significantly intertwined with the grid's ability to integrate these resources seamlessly."
Technological Barriers
Various technological challenges present significant obstacles to the broad adoption of distributed energy systems. The rapid advancement of technology often outpaces the existing infrastructure and regulatory frameworks, leading to inefficiencies.
- Compatibility: Many distributed energy systems, such as solar panels or wind turbines, use inverter technology that can be incompatible with older grid infrastructures.
- Energy Storage Limitations: Efficient energy storage remains an unresolved issue. Technological advancements in batteries are essential to ensure that surplus energy can be stored and utilized effectively during high demand.
- Data Security: Increased connectivity introduces vulnerabilities. Securing these systems against cyber threats is paramount for preventing disruptions.
The integration of new energy technologies often requires a transitional phase where old systems must be modified or replaced. This adds both time and cost to the overall implementation process.
Resistance from Traditional Utilities
Traditional utilities play a critical role in the energy system but often are resistant to the rise of distributed energy models. Their business models typically rely on centralized generation sources, meaning that decentralized production can threaten their established profitability. Consequently, utilities may view distributed energy as a competitive threat rather than a complementary resource.
Points of contention often include:
- Revenue Models: With more consumers generating their own energy, utility revenues can decline, leading to higher rates for non-participating customers.
- Infrastructure Investment: Utilities are generally hesitant to invest in the necessary infrastructure to support distributed systems due to concerns about their economic viability.
- Regulatory Alignments: There can be significant dissonance between utility companies and regulatory bodies, complicating efforts to promote distributed energy solutions.
These factors contribute to an environment where innovation can be slow, and progress may be stifled. Encouraging collaboration between traditional utilities and distributed energy advocates is important for overcoming these challenges effectively.
The challenges facing distributed energy implementation are multifaceted. Both technical and regulatory obstacles create a complex landscape for developers and policymakers. Understanding these issues is essential for formulating strategies that can pave the way for a more integrated and sustainable energy future.
Future Trends in Distributed Energy
The evolution of distributed energy systems reflects a significant shift in how energy is produced, consumed, and managed. As society continues to prioritize sustainable practices, understanding future trends in distributed energy is crucial. This knowledge helps stakeholders anticipate changes and adapt strategies effectively. The increasing importance of local energy generation and grid integration provides multiple benefits. These benefits include improved energy security, reduced transmission losses, and enhanced grid resilience amidst environmental uncertainties. Furthermore, ongoing advancements in technology and regulation will continue shaping the future landscape of distributed energy.
Decentralization of Energy Production
Decentralization signifies a move away from traditional, centralized energy models. Instead of relying on large power plants and extensive transmission networks, energy production is localized. This shift offers multiple advantages. Local systems can enhance energy independence and facilitate rapid adaptation to local needs. By generating energy closer to where it is consumed, communities can reduce reliance on failing grids and external energy sources.
The rise of decentralized systems also leads to increased participation among consumers, sometimes referred to as prosumers. These individuals not only consume energy but can also generate and sell excess energy back to the grid.
Some key points about decentralization include:
- Enhanced Energy Resilience: Localized systems reduce the risk of large-scale outages.
- Increased Accessibility: Decentralized energy allows remote and underserved communities to access reliable energy.
- Reduced Transmission Costs: Producing energy near end-users decreases costs related to long-distance transmission.
Thus, the future of energy production likely favors a decentralized approach that empowers local communities to take control of their own energy needs.
Integration of Artificial Intelligence
Artificial intelligence (AI) is poised to play an essential role in the future of distributed energy systems. AI technologies have the potential to optimize energy generation, distribution, and consumption processes. Automation can lead to increased efficiency and enhanced decision-making capabilities in energy management.
Key areas where AI can impact distributed energy include:
- Smart Grid Management: AI can analyze data in real-time, allowing for better management of energy flows and demand response mechanisms.
- Predictive Maintenance: AI can help anticipate maintenance needs for localized energy systems, thus reducing downtime and extending equipment life.
- Consumer Behavior Analysis: Understanding consumption patterns can help tailor energy prices and incentives, enhancing operational efficiency.
As AI technologies advance, they will refine the performance of distributed energy systems. The capability to adapt dynamically to changing environments will be indispensable in achieving a sustainable energy future.
Embracing future trends like decentralization and AI integration offers significant potential for resilience and sustainability in distributed energy systems.
Closure
The conclusion of this article is crucial in synthesizing the key elements discussed regarding distributed energy systems. A nuanced understanding of distributed energy is key today as the energy landscape undergoes significant transformation. It emphasizes the importance of localized energy generation and the growing shift towards decentralized energy production that can operate both independently and with the conventional grid.
One main benefit of distributed energy systems is their ability to enhance energy security. By diversifying energy sources and enabling localized production, these systems can mitigate the risks associated with reliance on large, centralized power plants. Furthermore, they can improve resilience against energy shortages and disruptions, often exacerbated by natural disasters or infrastructural failures.
In addition to energy security, sustainability must also be highlighted. The shift towards renewable energy sources through distributed systems contributes to lowering carbon emissions and promoting environmental stewardship. The integration of technologies like smart grids and energy storage solutions is set to bolster efficiency and reliability, crucial components in addressing climate change challenges.
Moreover, the economic implications are significant. With investments in distributed energy systems, job creation becomes a tangible benefit, stimulating local economies and fostering innovation. This is particularly relevant in a world where energy jobs are evolving and new skills are consistently required.
However, challenges remain. Regulatory frameworks may need restructuring to facilitate growth in this sector. Traditional utilities may resist the change due to self-interest, and overcoming these barriers is a critical aspect of future developments in distributed energy. Remote areas might need further support to adopt these technologies fully.
In summary, the conclusion serves to tie together these essential threads and reinforce that distributed energy is not merely a trend but a fundamental transition towards more sustainable, resilient, and economically beneficial energy systems. It is vital for educators, researchers, students, and professionals to understand this shift as they navigate the rapidly evolving energy landscape.