Understanding CRISPR Therapeutics: Revolutionary Advances
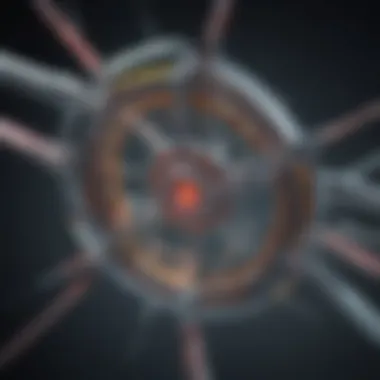
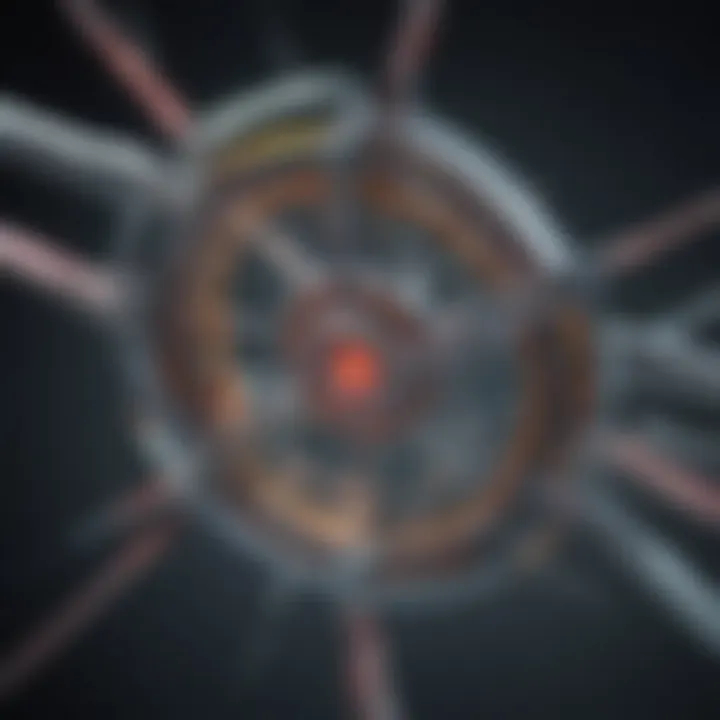
Intro
CRISPR therapeutics is emerging as a significant area of research, revolutionizing the way genetic diseases are understood and treated. Through gene editing, it promises avenues for possible cures for conditions once deemed untreatable. Individuals navigating this complex landscape need to develop a foundation in how CRISPR operates. This includes understanding the principles, mechanisms, and applications of this transformative technology.
A deep dive into CRISPR unveils numerous dimensions of its therapeutic potential. The technology has implications across various sectors, from medicine to agriculture. Despite the excitement surrounding its applications, ethical considerations present a formidable challenge. Various stakeholders must tread carefully in this evolving field as the responsibility that accompanies manipulation of the genetic code can lead to unintended consequences.
The discussion will extend beyond technical details into implications for healthcare, society, and future research directions. A nuanced understanding of the data, the methods employed, and limitations of studies will also play a critical role in comprehending CRISPR's full potential.
Methodology
Study Design
In studying CRISPR therapeutics, a mixed-methods approach is often employed. This design integrates qualitative and quantitative research methodologies to yield a rounded view of the subject matter. Literature reviews form the basis for identifying trends in current research, while case studies provide insights from actual genetic interventions. Parallel explorations of ethical frameworks are critical to draw comprehensive conclusions about CRISPR's impact.
Data Collection Techniques
Data is collected through a variety of techniques, including:
- Literature Reviews: Gathering information from scholarly articles, journals, and online databases like Wikipedia and Britannica.
- Interviews: Engaging with experts in genetics, ethics, and policy can provide deeper insights.
- Surveys: Capturing opinions from a broader audience for insights on societal perceptions of gene editing.
- Experimental Research: Observing results from clinical trials to gather empirical data on CRISPR applications.
"Understanding the methodology behind CRISPR research sheds light on both the scientific and ethical landscapes shaping the technology today."
Discussion
Interpretation of Results
Findings in CRISPR research highlight both advancements and challenges. The dataset reveals substantial success in clinical trials targeting genetic disorders such as sickle cell anemia and muscular dystrophy. Yet, these achievements are tempered by questions around safety, efficacy, and long-term impacts on human health.
Limitations of the Study
Despite promising results, limitations persist. The research often relies on animal models, which may not fully translate to human outcomes. Additionally, ethical dilemmas complicate the establishment of regulations and guidelines for CRISPR applications. Notably, long-term effects remain unquantified.
Future Research Directions
Future studies must focus on several key areas:
- Refining delivery systems for CRISPR components to improve specificity.
- Exploring off-target effects of gene editing to enhance safety.
- Investigating public attitudes towards gene editing to guide policy.
- Collaborating across disciplines to harmonize technological advances with ethical considerations.
The complexities of CRISPR therapeutics require ongoing dialogue and careful scrutiny from all involved disciplines. As research progresses, maintaining a balanced approach will be paramount to harnessing its full potential.
Overview of CRISPR Technology
The significance of CRISPR technology cannot be overstated. In the context of this article, it serves as a linchpin for understanding how genetic engineering is transforming medicine and biotechnology. CRISPR, or Clustered Regularly Interspaced Short Palindromic Repeats, is more than just a groundbreaking tool; it is a paradigm shift in our approach to genetic manipulation.
The essentials of CRISPR lie in its fundamental mechanisms, designed to edit specific sections of DNA. This allows for precision in targeting genes that contribute to various diseases, ranging from hereditary disorders to certain types of cancer. The implications for therapeutic applications are vast, as CRISPR can potentially correct genetic defects, enhance immunity, or even create personalized medicine solutions tailored to individual genomic profiles.
However, the use of CRISPR technology carries responsibilities. As its applications continue to expand, bioethical considerations must be at the forefront of discussions. This includes understanding the potential long-term effects of gene editing on both individuals and the larger ecosystem.
In this section, we will explore key aspects such as the fundamentals of CRISPR and its historical development to set a robust foundation for subsequent discussions on its mechanisms and applications.
Fundamentals of CRISPR
CRISPR technology consists of a simple yet powerful system that allows for targeted genome editing. Its primary components include the Cas9 protein and RNA sequences designed to guide this protein to a specific DNA sequence. The process starts with the identification of a target sequence within the genome, facilitated by the guide RNA that matches the DNA. Once the target is engaged, Cas9 acts as a molecular scissor, precisely cutting the DNA at the desired location.
This cutting mechanism opens pathways for gene modification. Following a cut, the cell's natural repair processes come into play. These can be harnessed to introduce specific changes, such as correcting harmful mutations or inserting new genetic material. This targeted approach is what distinguishes CRISPR from earlier gene-editing techniques, offering a level of specificity that was previously unattainable.
Historical Development
The journey of CRISPR technology began in the realm of microbiology, where researchers observed its presence in the immune systems of bacteria. The discovery of the CRISPR-Cas system in the 1980s laid the groundwork for its later adaptation for gene editing in higher organisms.
In 2012, Jennifer Doudna and Emmanuelle Charpentier made a pivotal breakthrough by repurposing the CRISPR-Cas9 system for genome editing. Their pioneering work demonstrated its potential as a versatile tool for various species, not just bacteria. This landmark development sparked a surge of global interest in CRISPR and accelerated research into its vast therapeutic possibilities.
Since then, CRISPR has expanded rapidly, leading to numerous publications and clinical trials. The ongoing evolution of CRISPR technology emphasizes not just its scientific merits but also the need to navigate the associated ethical complexities. Understanding the history of CRISPR is crucial, as it establishes a context for both its capabilities and responsibilities moving forward.
Mechanism of Action
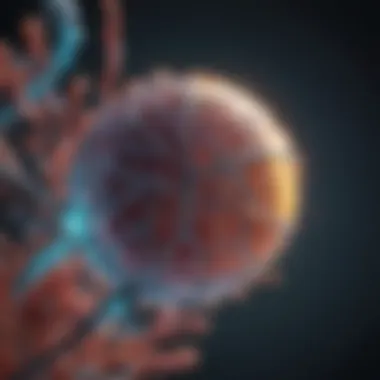
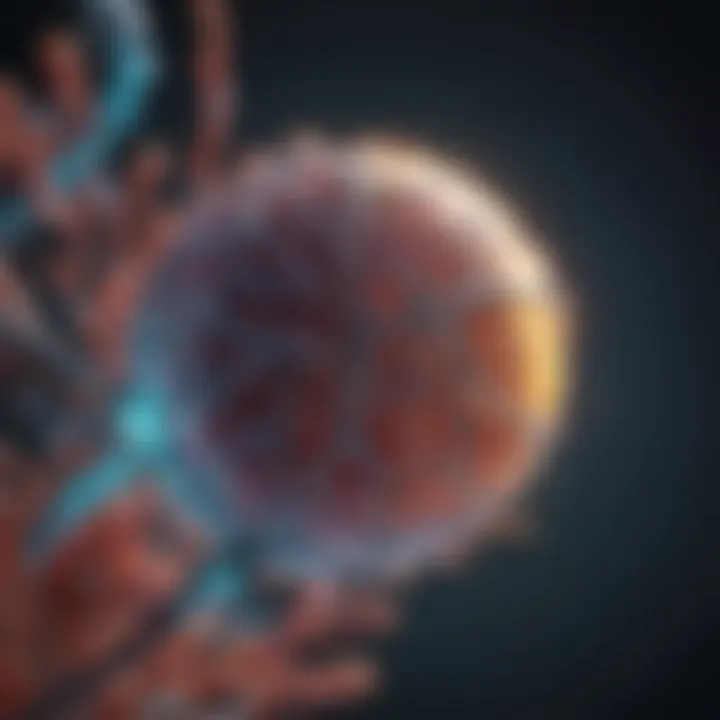
The mechanisms underlying CRISPR technology are fundamental to its role in genetic editing. Understanding these mechanisms is critical for grasping how CRISPR can be effectively utilized in therapeutic contexts. It delineates the processes that allow scientists to target, cut, and modify DNA sequences precisely, which can lead to significant medical advancements. Each stage of this mechanism comes with its own benefits and considerations that impact its application in therapeutic settings.
Components of CRISPR-Cas9
The CRISPR-Cas9 system consists of two primary components: the guide RNA (gRNA) and the Cas9 enzyme.
The gRNA is crucial because it directs the Cas9 enzyme to a specific location on the DNA that requires editing. The precision of this targeting is what makes CRISPR-Cas9 a powerful tool in gene editing. Cas9 functions as a molecular scissors that creates a staggered cut in the DNA at the site specified by the gRNA.
This combination of gRNA and Cas9 is especially effective because it enables researchers to tailor their approach for various applications, ranging from correcting genetic mutations to investigating gene functions.
Gene Editing Process
The gene editing process can be broken down into three essential steps: targeting DNA, cutting mechanism, and repair mechanisms.
Targeting DNA
Targeting DNA is the first critical step in the gene editing process. This phase involves the design of the gRNA to match a specific DNA sequence. The gRNA attaches to its complementary DNA strand, and this pairing is highly specific. This specificity is a key characteristic of targeting DNA and contributes to the overall efficiency of CRISPR technology.
Because of this high precision, targeting DNA minimizes risks of off-target effects, where unintended sections of DNA might be altered. However, designing an effective gRNA can be challenging due to the complexity of genomic sequences.
Cutting Mechanism
The cutting mechanism involves the action of the Cas9 enzyme. Once the targeting is successful, Cas9 binds to the DNA at the site specified by the gRNA and makes a double-strand break in the DNA. This feature of CRISPR-Cas9 is one of its most beneficial aspects as it allows for the precise editing of genomes at designated locations. The reliability of this cutting process is a strength of CRISPR, but it also brings about concerns related to potential off-target cuts, which can lead to unintended consequences.
Repair Mechanisms
After the DNA has been cut, the cell's natural repair mechanisms come into play. Repair mechanisms can either lead to non-homologous end joining (NHEJ) or homology-directed repair (HDR). NHEJ is the more common pathway, often resulting in insertions or deletions at the cut site. This process can disrupt gene function, which may be desirable in some contexts, such as knocking out unwanted genes. Conversely, HDR can be used for precise edits, allowing researchers to introduce specific changes by providing a template DNA sequence.
While HDR is a powerful tool, it is less efficient than NHEJ and can be harder to control in certain cell types.
"Understanding the mechanisms of CRISPR-Cas9 is essential for harnessing its potential in gene therapy and biotechnology."
In summary, the mechanism of action of CRISPR is characterized by a precise targeting system enabled by the gRNA, a reliable cutting process through Cas9, and subsequent repair mechanisms that can either disrupt or reform genes. Each component plays a pivotal role in the overall efficiency and accuracy of CRISPR, making it a revolutionary tool in genetic technology.
Applications in Medicine
The applications of CRISPR technology in medicine stand as a testament to its transformative potential in addressing various diseases. As CRISPR advances through continued research, its relevance in treating genetic disorders, cancer, and in regenerative medicine becomes increasingly clear. The versatility and precision of CRISPR make it a topic of significant interest in medical research and clinical applications, catering to a wide range of conditions. This section outlines these pivotal applications, providing insight into their impact on healthcare.
Genetic Disorders
Monogenic Disorders
Monogenic disorders arise from mutations in a single gene, making them a straightforward target for gene editing. Diseases such as cystic fibrosis and sickle cell anemia exemplify this category. The nature of monogenic disorders allows researchers to utilize CRISPR to correct or replace the faulty genes effectively. This specificity trends towards a lower risk of off-target effects, which is a significant advantage of using CRISPR in these cases.
In addition, the simplicity of targeting single genes often results in faster clinical trials. However, it is essential to consider that not all monogenic disorders have equally effective responses to CRISPR. The diversity within these conditions requires careful consideration of how gene editing can be best applied.
Polygenic Conditions
Polygenic conditions, on the other hand, are influenced by multiple genes. This complexity presents significant challenges when employing CRISPR for treatment. Conditions such as diabetes and heart disease involve numerous genetic and environmental factors which complicate straightforward editing. Despite these challenges, CRISPR holds promise in researching these conditions, helping to identify potential targets for intervention.
A key feature of polygenic conditions is their multifactorial nature, which makes CRISPR’s role less about direct correction and more about understanding the underlying genetic interactions. Exploring these interactions can lead to insights that benefit preventative strategies and individualized treatments. However, the outcomes may not be as predictable or controlled as those seen with monogenic disorders.
Cancer Therapeutics
Targeting Specific Mutations
CRISPR’s ability to target specific mutations in cancer cells demonstrates its potential to drastically change cancer therapeutics. Mutations in oncogenes and tumor suppressor genes drive cancer progression, presenting an opportunity for targeted gene editing. By focusing on these mutations, researchers aim to halt or reverse tumor growth, offering hope for more effective therapies.
A critical characteristic of targeting mutations is its precision. The ability to edit genes directly related to cancer provides a targeted approach that could lead to personalized treatments. However, the complexity of cancer genomics must be acknowledged. Cancer cells can exhibit heterogeneous mutations, leading to difficulties in ensuring all cancerous cells are adequately targeted by CRISPR.
Enhancing Immunotherapy
In recent years, CRISPR technology has been used to enhance immunotherapy approaches. By modifying immune cells, such as T-cells, CRISPR can increase their ability to recognize and destroy cancer cells. This enhancement can lead to more potent therapies, potentially transforming how certain cancers are treated.
The role of CRISPR in immunotherapy underscores its versatility and adaptability. However, challenges remain in understanding the implications of modifying immune responses, which could lead to unintended consequences, such as autoimmunity. Striking a balance between therapeutic benefits and safety is critical in this application.
Regenerative Medicine
Tissue Engineering
Tissue engineering harnesses CRISPR to create biological substitutes that improve or restore tissue function. This application has the potential to revolutionize treatment strategies for injuries or degenerative diseases. By using CRISPR to edit stem cells or other cell types, personalized tissues can be produced. The idea of creating tissues tailored to individual needs exemplifies the power of CRISPR in regenerative medicine.
A significant characteristic of tissue engineering is its capacity to combine biological and synthetic materials for improved healing. CRISPR's role in enhancing these advances is vital. However, sustainable practices and long-term results must be evaluated continuously to ensure safety and effectiveness in clinical applications.
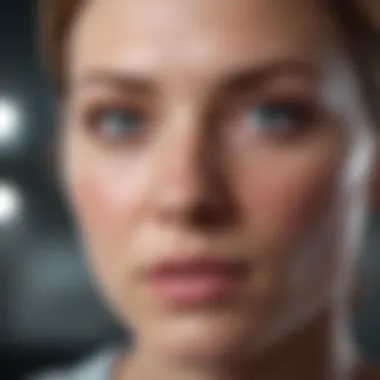
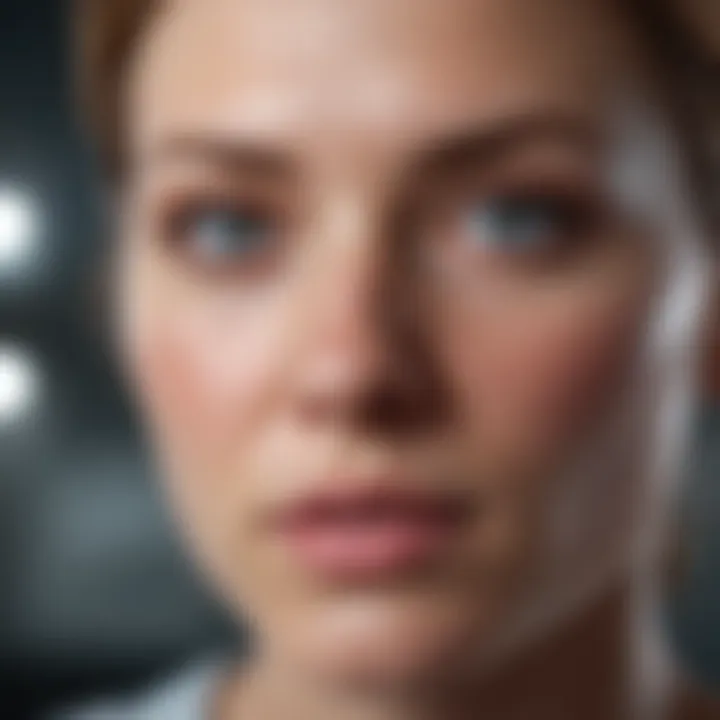
Stem Cell Modification
The modification of stem cells using CRISPR presents numerous avenues for regenerative medicine. By targeting specific genes in stem cells, researchers can direct their differentiation into desired cell types. This application has implications for treating conditions such as spinal cord injuries or heart disease. The ability to impact stem cells fundamentally marks a critical development in treating complex diseases.
One unique aspect of stem cell modification using CRISPR is its capability to improve cell survival and function after transplantation. Despite the positives, ethical implications about manipulating stem cells must remain at the forefront of discussion. As the technology evolves, ethical considerations must be balanced with scientific advancements to maintain public trust.
The applications of CRISPR in medicine represent not just a scientific breakthrough but the dawn of a new era in healthcare.
Current Research Status
The field of CRISPR therapeutics is evolving rapidly, with ongoing research significantly impacting its potential applications. The current research status encompasses clinical trials and emerging technologies. Both elements are crucial for assessing the therapeutic capabilities of CRISPR. As scientists explore various aspects, understanding the progress in this area fosters a clearer picture of CRISPR's role in medicine.
Clinical Trials Overview
Successful Trials
Successful trials in CRISPR research demonstrate the therapeutic efficacy and safety of gene editing in clinical settings. Recent trials, like those addressing sickle cell disease and beta-thalassemia, showcase the promise of CRISPR in treating genetic disorders. These trials often involve altering patients' blood cells to create a healthy state. The key characteristic of these trials is the potential to significantly improve patients' quality of life.
The unique feature of such successful trials is their ability to offer long-lasting results, often after a single treatment. This advantage is compelling, providing clear support for further advancements in CRISPR applications. Successful trials also help in obtaining regulatory approvals more swiftly, leading to quicker access to innovative therapies.
Trials Underway
Trials currently underway are equally significant in advancing CRISPR research. These trials focus on diverse areas, including cancer treatment and genetic disorders that have not seen effective therapies previously. The key characteristic of ongoing trials is their exploration of different delivery methods for CRISPR components, which is essential for maximizing therapeutic efficacy.
A unique feature of these trials is their inclusion of adaptive designs, which allow researchers to modify protocols based on real-time results. This flexibility offers advantages in optimizing treatment plans. However, it may lead to ethical concerns about informed consent and patient safety, factors that demand careful consideration as research proceeds.
Emerging Technologies
CRISPR Variants
CRISPR variants represent an exciting development in gene editing techniques. These include newer systems, such as CRISPR-Cas12 and CRISPR-Cas13, which offer distinct advantages over the traditional Cas9. Their key characteristic lies in improved specificity and reduced off-target effects, making them a promising choice for therapeutic applications.
The unique feature of CRISPR variants is their potential to target RNA, not just DNA. This capability expands the therapeutic horizon for conditions like viral infections and genetic disorders caused by RNA. These variations provide both advantages and disadvantages. For instance, they may enhance the precision of treatments, but researchers must conduct further studies to ascertain long-term effects and safety.
Delivery Systems
The development of effective delivery systems is a fundamental aspect of CRISPR research. These systems ensure that CRISPR components are efficiently introduced into cells. The key characteristic of advanced delivery systems is their ability to target specific tissues or cells, thereby enhancing therapeutic outcomes.
A unique feature of these systems includes the use of nanoparticles, which can encapsulate CRISPR components for safer and more effective delivery. While this approach offers significant advantages, challenges remain in terms of scalability and ensuring consistent delivery efficiency. Ongoing research aims to navigate these hurdles for practical applications in clinical settings.
"The ongoing progress in both clinical trials and emerging technologies is pivotal in determining the future landscape of CRISPR therapeutics."
Ethical Considerations
The discussion surrounding CRISPR therapeutics cannot be complete without addressing ethical considerations. As this technology moves from the laboratory to clinical applications, various ethical dilemmas arise. These dilemmas are critical to ensuring safe, equitable, and responsible use of CRISPR technology in healthcare and beyond.
Biosafety and Biosecurity
Biosafety focuses on preventing unintentional release of genetically modified organisms, while biosecurity aims to prevent their misuse in a potential bioweapon context. The risks associated with CRISPR, such as the possibility of off-target effects, mean that thorough evaluation of safety protocols is necessary. Misapplications could lead to unintended consequences in human health or the environment. It is essential for researchers and institutions to establish clear guidelines to manage risks associated with gene editing. Regulatory bodies must also impose stringent safety checks before any CRISPR-derived therapy can be approved for human use.
Equity in Access to Treatment
Equity in access to CRISPR treatments is paramount. As therapies are developed, they may initially only be available to individuals who can afford them. This opens a wider gap in healthcare disparities. Addressing these concerns involves not only creating affordable treatment options but also ensuring that marginalized populations have access to these innovations. Prioritizing inclusivity in research design, funding, and healthcare infrastructure can help mitigate potential inequalities. Policymakers must foster a framework that promotes fair access while considering the ethical implications of social justice in healthcare.
Genetic Privacy
Maintaining genetic privacy is a significant ethical issue tied to CRISPR therapeutics. Genetic modification often involves sensitive personal data sharing, which, if mismanaged, can lead to privacy violations. The possibility of genetic information being used without consent raises concerns about who controls this data and how it is utilized. Guidelines must be established to protect individuals’ privacy rights, ensuring that genetic information is handled with sensitivity and respect. Regulations should promote transparency regarding data use, empowering patients to make informed decisions about their genetic information.
"As we advance into an era of genetic editing, it is our collective responsibility to navigate the moral landscape with awareness and caution."
The ethical considerations surrounding CRISPR technology require ongoing dialogue. As the technology continues to evolve, it is vital to keep these discussions at the forefront of its development and implementation. Ensuring accountability and ethical integrity will ultimately shape the future of CRISPR in medicine.
Challenges and Limitations
Understanding the challenges and limitations of CRISPR therapeutics is essential in evaluating its potential applications in medicine. As groundbreaking as CRISPR technology is, it faces several significant hurdles that could affect its efficacy and acceptance. The focus on these challenges is not merely academic; it informs future research directions and ethical considerations, ultimately shaping the impact of CRISPR on healthcare.
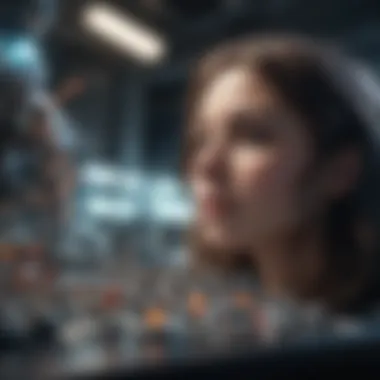
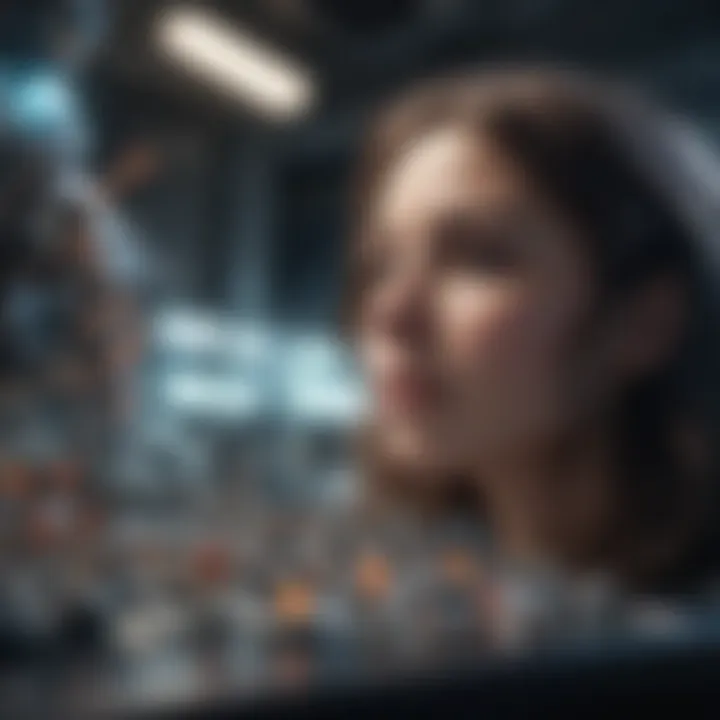
Off-Target Effects
Off-target effects are unintended edits in the genome that occur when the CRISPR-Cas9 system cuts DNA at sites other than the targeting sequence. These effects can lead to unpredictable genetic alterations, raising serious safety issues. Recent studies have shown that while CRISPR is robust in its precision, it is not infallible. Depending on the guide RNA's design, there can be mismatches with unintended sequences.
Minimizing off-target effects is vital for enhancing the safety profile of CRISPR-based therapies. Methods like high-throughput sequencing assist researchers in assessing potential off-target sites.
Technical Constraints
Technical constraints of CRISPR include limitations related to its efficiency in different cell types and tissues. Not all cells take up the CRISPR components equally well. This variation can affect the consistency of outcomes in therapeutic applications. Moreover, the current delivery methods, such as viral vectors or lipid nanoparticles, have their own sets of challenges, including immune responses and short-lived expressions of transfected genes.
Furthermore, the scope of gene edits permissible is still limited. Larger genetic modifications or simultaneous edits to multiple genes are still areas needing refinement.
Regulatory Hurdles
Regulatory approval is another significant limitation for CRISPR technologies. Given the nature of gene editing, the ethical and legal implications are complex. Regulatory bodies such as the FDA in the United States and EMA in Europe are still developing frameworks for CRISPR applications. The ambiguity surrounding regulations can lead to delayed innovations and slow the translation of research into clinical therapies. Moreover, ensuring patient safety while navigating these regulations introduces additional layers of complexity.
"The ethical implications of gene editing are profound, requiring in-depth discussions among scientists, ethicists, and policymakers."
Future Directions
The exploration of future directions in CRISPR therapeutics is essential. It highlights the significant advancements anticipated in the realm of genetic engineering. As research in gene editing progresses, the potential of CRISPR expands beyond current applications. Several areas deserve close attention, including improvements in delivery mechanisms, new applications in various fields, and the integration of CRISPR with other technologies.
Advancements in Delivery Mechanisms
Delivering CRISPR components accurately to target cells remains a key challenge. Innovations in delivery systems can enhance precision, making treatments more effective. Methods like lipid nanoparticles, viral vectors, and electroporation are being examined. These advancements can significantly improve the therapeutic outcome. By refining these delivery methods, researchers can ensure that CRISPR reaches specific tissues or organs. This targeted approach minimizes side effects and increases the efficiency of gene editing.
Potential New Applications
Crop Improvement
Crop improvement through CRISPR can transform agricultural practices. Modifying crops to resist pests or tolerate harsh conditions is critical for food security. This approach enables the development of crops with desired traits more rapidly and accurately than traditional breeding techniques. The efficiency and speed of CRISPR make it a valuable tool in biotechnology. However, concerns arise regarding ecological impacts and public acceptance of genetically modified organisms.
Virus Resistance
The potential of CRISPR in developing virus resistance is noteworthy. This includes designing plants that can withstand viral infections or creating therapies for viral diseases in humans. Enhancing resistance to common viral diseases will improve agricultural yield and food supply. Additionally, in human medicine, gene therapies can target viral genome integration, offering new treatment avenues. Despite these benefits, challenges include ensuring specificity and managing possible off-target effects.
Integration with Other Technologies
AI and Machine Learning
Integrating AI and machine learning with CRISPR can revolutionize design and efficiency. These technologies can predict off-target effects and optimize guide RNA sequences. Utilizing algorithms could streamline the process, enabling swift adjustments based on experimental data. The fusion of AI with genetic engineering is a forward-looking approach that enhances precision and reduces trial and error. However, reliance on technology could pose risks if not properly regulated.
Nanotechnology
Nanotechnology offers promising solutions for CRISPR delivery challenges. The use of nanoparticles can provide a means to deliver CRISPR components effectively, protecting them during transit in biological environments. This integration can lead to more targeted and sustained gene editing. Nonetheless, there are safety and regulatory concerns regarding nanomaterials that must be addressed to foster public trust in these technologies.
The future of CRISPR therapeutics is ripe with possibilities, but it requires careful navigation of the associated challenges to realize its full potential.
End
The conclusion offers a vital summation of the discussions around CRISPR therapeutics. This article highlights the revolutionary impact CRISPR has on genetic medicine. It covers the importance of understanding mechanisms, applications, and ethical considerations. Key insights from the research serve to emphasize CRISPR's transformative potential.
In summarizing the key points:
- Mechanisms articulate the specific processes that allow targeted gene editing.
- Applications in medicine provide insight into how CRISPR can treat genetic disorders and improve cancer therapies.
- Ethical considerations raise awareness about the implications of manipulating genetic material.
By integrating these elements, the conclusion encapsulates the scope of CRISPR and urges further exploration into its possibilities and consequences.
Summary of Key Insights
Understanding CRISPR is essential in modern biosciences. Key insights include:
- CRISPR's precision allows for specific targeting of genes, offering a method to correct genetic disorders.
- Ongoing clinical trials demonstrate real-world applications. These trials signify a shift in how disorders are treated, moving away from traditional methods.
- Ethical discussions inform how society should approach gene editing. Issues like genetic privacy and equity in access to treatments are critical to consider.
As research progresses, the importance of dissemination and comprehension of these insights increases. Education on CRISPR not only builds awareness but also fosters informed discussions on its use.
The Path Forward
The future of CRISPR therapeutics is promising yet complex. Several paths may unfold:
- Advancements in delivery mechanisms can enhance the efficiency of gene editing while reducing off-target effects.
- Potential new applications outside of medical treatments, such as enhancing crop resilience, showcase the broad influence of CRISPR.
- Integration with technologies like AI and machine learning can streamline CRISPR processes, improving our ability to predict outcomes and refine editing techniques.
As researchers address current limitations, the discipline may need to embrace collaborations across fields. Continued investment in research and infrastructure will be necessary for ethical implementation and maximizing benefits from CRISPR technology. Each step forward will deepen our understanding of genetics and biology.