Understanding the Complex Functionality of the Retina
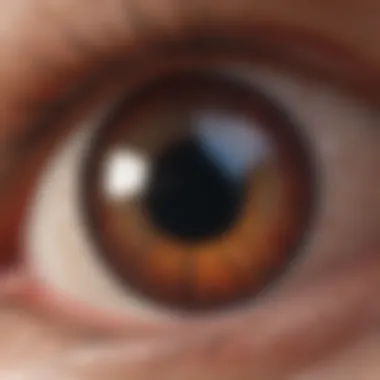
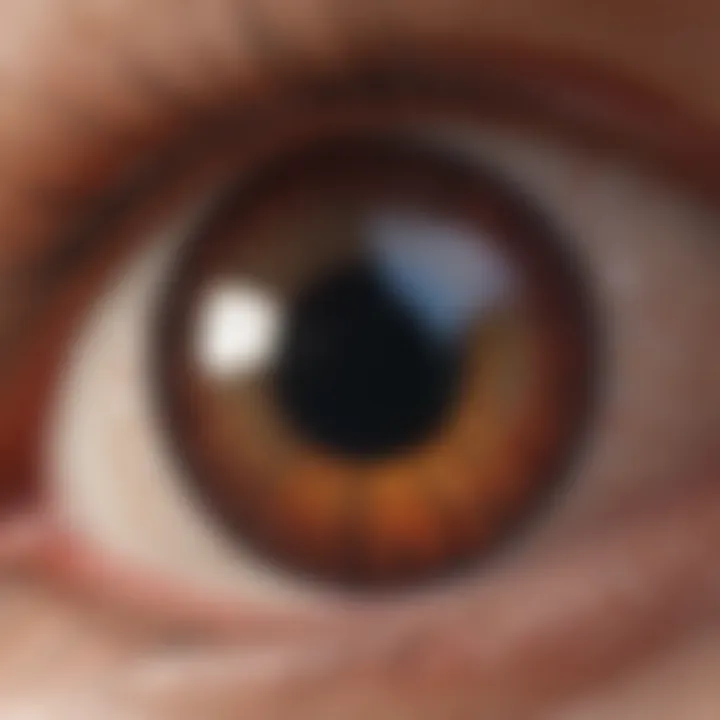
Intro
The retina is a complex structure located at the back of the eye. Its primary role is to convert light into neural signals, enabling vision. This article delves into the intricacies of the retina, including its anatomy, cell types, and the mechanisms behind visual perception. It also addresses the effects of various diseases on retinal health, emphasizing the need for ongoing research in this vital area of vision science.
Methodology
Understanding the retina's functionality requires a systematic approach.
Study Design
The exploration of retinal functionality often includes both observational studies and experimental research. Observational studies typically examine human subjects to understand how visual impairment relates to retinal health. Experimental studies may involve animal models to investigate specific cellular processes. This hybrid approach provides a comprehensive picture of retinal function and its disorders.
Data Collection Techniques
Data collection for retinal studies can employ various techniques:
- Electrophysiological Measurements: These assess the electrical responses of retinal cells to light stimulation. They provide insights into how signals are transmitted within the retina.
- Imaging Techniques: Methods like Optical Coherence Tomography (OCT) allow for in-depth imaging of the retinal layers. High-resolution imaging is crucial to visualize structural changes caused by diseases.
- Genetic Analysis: Understanding the genetic underpinnings of retinal diseases can reveal patterns and risk factors associated with specific conditions.
Data collected from these techniques can lead to a better understanding of both healthy and diseased retinas.
Discussion
Exploring the retinal functionalities sheds light on numerous important aspects:
Interpretation of Results
The findings from various studies indicate several key points. Retinal cells, including photoreceptors, bipolar cells, and ganglion cells, work in concert to process visual information. Disruption in any of these cell types can lead to visual impairments. For instance, degeneration of photoreceptors is closely linked to conditions such as retinitis pigmentosa.
Limitations of the Study
Despite the advancements in understanding retinal functionalities, some limitations exist. Variability in study design and sample sizes can affect the reliability of results. Furthermore, translating findings from animal models to humans poses challenges. Not all processes may be identical between species.
Future Research Directions
Ongoing and future research should focus on several areas. Investigating novel therapies for retinal degenerative diseases holds promise. Gene therapy, stem cell interventions, and new pharmacological approaches can help restore or enhance vision. Additionally, the relationship between retinal health and systemic diseases warrants further examination.
"Improving our understanding of retinal function will allow us to develop better interventions for vision loss."
Prelims to the Retina
The retina plays a fundamental role in the visual system. It is not just a passive layer of tissue; rather, it is an intricate structure that processes light and converts it into neural signals. Understanding the retina's multifaceted functionality is essential for grasping how we perceive our environment. This introduction sets the stage for a deeper examination of the retina’s anatomy, phototransduction, and visual processing pathways.
Definition and Overview
The retina is a thin layer of tissue located at the back of the eye. It contains light-sensitive cells that are responsible for converting incoming light into electrical signals. These cells include photoreceptors, bipolar cells, and retinal ganglion cells. When light hits the retina, it triggers a series of biochemical reactions that result in the generation of neural impulses. These impulses travel through the optic nerve to the brain, where they are processed into images.
In addition to light detection, the retina also has a complex organization. It includes various layers, such as the inner and outer layers, which contain distinct types of cells. The retinal pigment epithelium, found at the outer layer, provides crucial support to photoreceptors. It plays a key role in the recycling of photopigments used in phototransduction, demonstrating the retina's significance in maintaining visual acuity.
Historical Context of Retinal Research
Research into the retina's functionality has evolved significantly over the centuries. Early studies focused on the anatomical structure of the eye, improving our understanding of where vision begins. With the development of technologies like electron microscopy, researchers gained insights into the cellular architecture of the retina.
In the late 20th century, advances in electrophysiology allowed scientists to measure the electrical activity of retinal cells. This paved the way for discovering the mechanisms of phototransduction. Additionally, studies on retinal diseases have become increasingly important, highlighting how dysfunction can lead to conditions such as diabetic retinopathy and age-related macular degeneration. As the field progresses, ongoing research continues to reveal the intricacies of retinal cells and the processes they undergo, proving essential in the journey toward finding new treatment options.
The retina is not merely a passive receptor; it is a dynamic structure essential for the overall health of visual perception.
In summary, the complexities of the retina facilitate our visual experiences. The importance of understanding this organ cannot be overstated, as it lays the foundation for numerous advancements in vision science.
Anatomy of the Retina
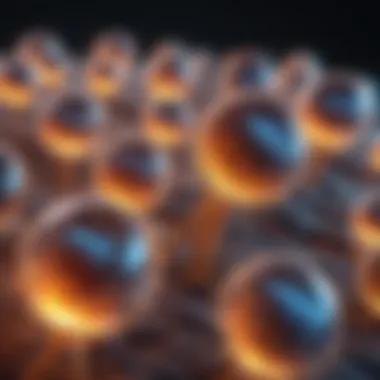
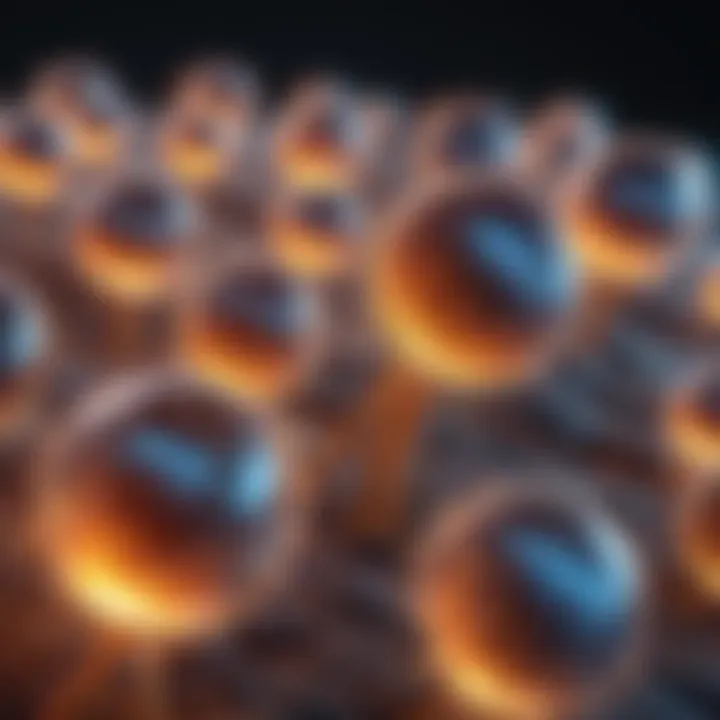
The anatomy of the retina is vital to understanding its complex functionality. The retina not only acts as the initial site for light detection but also serves as a key processing center for visual information before it is sent to the brain. A thorough knowledge of its anatomy aids in grasping how various retinal structures contribute to visual perception and overall ocular health.
Layers of the Retina
Inner Layer
The inner layer of the retina consists of different types of neurons, mainly bipolar cells and ganglion cells, which play crucial roles in relaying visual information. One key characteristic of the inner layer is its organization of these cells in distinct strata that facilitate effective signal transmission. This structure aids in the rapid processing of visual input, a beneficial aspect for this article's focus on real-time visual perception. A unique feature of this layer is its integration of signals from photoreceptor cells before transmission to the brain.
However, its functionality can be compromised in various retinal disorders, such as glaucoma, where ganglion cells degenerate, leading to vision loss.
Outer Layer
The outer layer of the retina primarily includes photoreceptor cells, known as rods and cones. This layer is essential for light detection, making it a significant aspect of the article. Its main characteristic is the ability to convert photon signals into electrical impulses due to the presence of specialized pigments. The photoreceptors’ unique feature lies in their distribution across the retina, with rods being more prevalent in the peripheral regions and cones concentrated in the fovea.
This arrangement allows for both low-light and color vision under different circumstances. Nonetheless, this layer is susceptible to damage from excessive light exposure or aging, bringing about issues such as macular degeneration.
Pigment Epithelium
The pigment epithelium, located beneath the photoreceptors, plays an integral role in retinal health and functionality. The main characteristic of this layer is its ability to absorb excess light to prevent scattering, which enhances visual acuity. This quality supports the article’s emphasis on clear vision. A unique feature is its role in the regeneration of visual pigments, which are essential for phototransduction.
However, dysfunction in this layer can lead to numerous retinal diseases, highlighting the importance of research in understanding how to protect and restore its health.
Retinal Cells
Photoreceptors
Photoreceptors, consisting of rods and cones, are the first neural elements that interact with light. The main aspect of these cells is their transformation of light into chemical signals, a crucial step in visual processing. The key characteristic is that rods are highly sensitive to light, while cones allow for color vision. Their unique feature is their placement within the retina, enabling diverse light conditions to be interpreted effectively.
Yet, degeneration of photoreceptors is common in retinal diseases, making their health a significant concern.
Bipolar Cells
Bipolar cells act as intermediaries between photoreceptors and ganglion cells. Their central role in relaying information makes them essential for visual signal transmission. The characteristic of bipolar cells is that they can integrate signals from multiple photoreceptors, thus contributing to the retina’s spatial and temporal resolution. Their unique ability to respond to a range of light intensities makes them pivotal in dim lighting conditions.
However, their functionality can be impaired under pathological conditions, impacting overall vision.
Retinal Ganglion Cells
Retinal ganglion cells are the final output neurons of the retina, transmitting processed visual information to the brain. Their main aspect is their capacity to carry signals via the optic nerve, providing a direct link to the visual cortex. A key characteristic is their diverse types, each responsible for different visual pathways. Their unique feature involves processing spatial and temporal aspects of vision, critical for motion detection and depth perception.
Despite their importance, ganglion cells are prone to damage from various retinal disorders, which can lead to irreversible vision loss.
Phototransduction Process
The phototransduction process is fundamental for vision. It is the mechanism by which light is converted into electric signals in the retina. This process occurs in specialized cells called photoreceptors, and it is crucial for the perception of light and vision. Understanding phototransduction allows researchers to better appreciate how visual information is processed and how disorders can affect this critical function.
Mechanism of Light Detection
Light detection begins when photons hit the photoreceptor cells, which come in two main types: rods and cones. Rods are sensitive to low light levels and are responsible for night vision, while cones detect color and are active in brighter conditions. The phototransduction cascade is initiated when light interacts with photopigments located within the photoreceptor cells.
When light strikes the retinal, a chromophore within the photopigments, it changes in structure. This triggers a series of biochemical reactions involving various proteins such as rhodopsin in rods and photopsins in cones. The change in the retinal structure activates a G-protein called transducin. Activated transducin then stimulates phosphodiesterase, which reduces levels of cyclic guanosine monophosphate (cGMP). As cGMP decreases, sodium channels close, leading to hyperpolarization of the photoreceptor cell and a decrease in the release of neurotransmitters to bipolar cells.
This complex sequence efficiently converts light into an electrical signal that can be transmitted to the brain. The ability to detect light and transform it into a neural signal is one of the retina's essential roles in the visual process.
Role of Photopigments
Photopigments are crucial for initiating the process of phototransduction. They are light-sensitive molecules that undergo structural changes when they absorb photons. The main photopigments include rhodopsin, found in rods, and different types of photopsins found in cones, each responding to specific wavelengths of light.
The functioning of these photopigments can be summarized as follows:
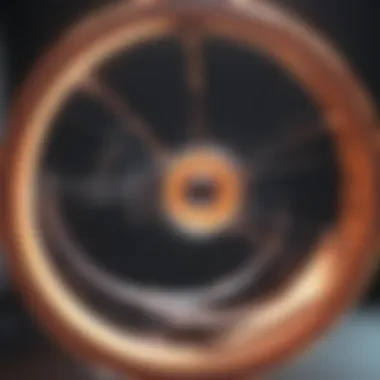
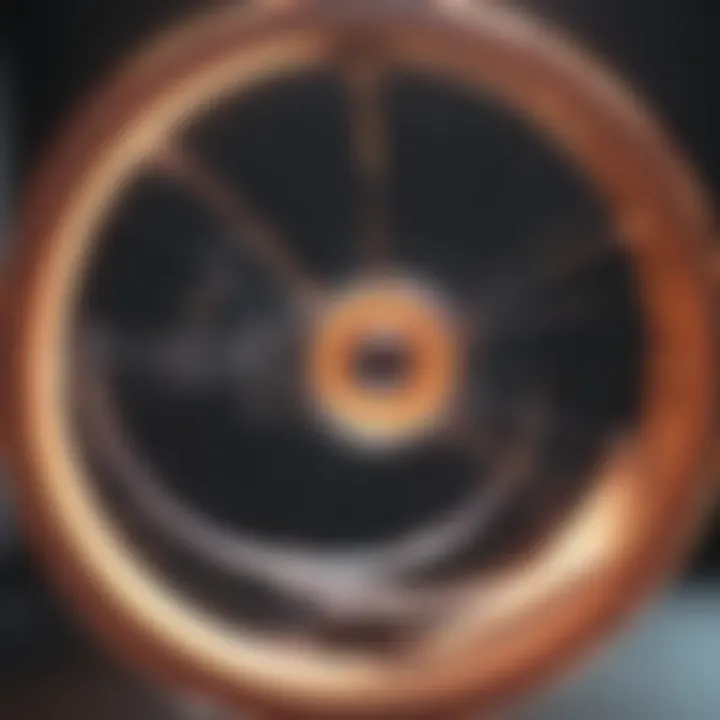
- Rhodopsin: Highly sensitive to dim light, crucial for vision in low illumination.
- Photopsins: There are three types corresponding to short (blue), medium (green), and long (red) wavelengths. Each cone cell contains one type of photopsin, allowing for color discrimination.
The regeneration of photopigments is a vital process. After phototransduction, the retinal must be converted back to its original form to be reused. This regeneration occurs in the retinal pigment epithelium through a series of enzymatic reactions.
Understanding photopigments is essential as their dysfunction can result in serious visual impairments, highlighting the importance of ongoing retinal research.
Visual Processing Pathways
Visual processing pathways are essential for understanding how the retina transmits visual information to the brain. The retina is not just a passive receiver of light; it actively processes images before relaying them through complex neural circuits. This phenomenon is crucial in elucidating how we perceive the world around us, and it is a central theme in this article.
Understanding visual processing pathways involves exploring several key elements. First, the interaction between different types of retinal cells plays a significant role. Photoreceptors, bipolar cells, and ganglion cells each contribute unique functions that help in encoding visual information. The precise communication among these cells ensures that the brain receives accurate representations of visual stimuli.
An important consideration in visual processing is the concept of parallel processing. In simpler terms, this allows different aspects of a visual scene to be processed simultaneously. For instance, the brain can detect edges, colors, and movements all at once. This multi-faceted processing enhances our ability to react swiftly to visual changes in our environment, which is vital for survival.
Moreover, disruptions within these pathways can lead to various visual impairments. Understanding the underlying mechanisms is beneficial for advancing therapeutic strategies targeting retinal disorders. Regaining or preserving visual function starts with comprehending these pathways and their complexities.
Signal Transmission to the Brain
Signal transmission is a critical step in the visual processing pathways. Once light is absorbed by the photoreceptors, it triggers a cascade of biochemical reactions known as phototransduction. This process converts light into electrical signals.
These electrical signals travel from the photoreceptors to bipolar cells, which act as a bridge, relaying the signals to retinal ganglion cells. Each type of retinal ganglion cell is tuned to specific aspects of the visual landscape, like detecting motion or changes in brightness. The axons of these ganglion cells form the optic nerve, which then carries the processed information to the brain. The transmission speed, and the fidelity of this information, is essential for accurate perception.
It is important to note that any disturbance in signal transmission can affect visual acuity. Understanding these effects helps researchers develop interventions to improve retinal function.
Visual Cortex Integration
Once the signals reach the brain, they are routed to the primary visual cortex located in the occipital lobe. Here, a higher level of processing takes place, integrating the raw data from the retina into coherent visual representations.
Integration involves combining information from multiple retinal ganglion cells, leading to perception of colors, shapes, and depth. The brain's ability to synthesize this information allows for complex interpretations, such as recognizing faces or navigating environments.
This integration is not static; it is dynamic and affected by various factors, including experience and attention. As a result, our perception can be influenced by context and familiarity with visual elements. For researchers, understanding these processes aids in grasping how learning and memory interact with vision. Overall, studying visual cortex integration unlocks insights into both normal and abnormal visual perception.
Role of the Retina in Visual Perception
The understanding of how the retina functions in visual perception is crucial for comprehending the entirety of the visual system. The retina acts as the initial stage in processing visual information. It accomplishes this by converting light into neural signals that the brain interprets as images. The health and integrity of the retina significantly affect one's ability to perceive and interpret visual stimuli.
A fundamental element of retinal function is its role in contrast and color vision. This involves a combination of photoreceptor activity and pathway signaling that conveys essential visual information to the brain. Moreover, the retina is not merely a passive receiver of visual stimuli. It engages in complex processing that enhances contrast and enables color differentiation in our environment.
Contrast and Color Vision
Contrast perception allows an individual to distinguish objects from their background. This process is essential for visual clarity. The retina features two types of photoreceptor cells: rods and cones. Rods are sensitive to light intensity and provide vision in low-light conditions. Cones, on the other hand, are responsible for color vision and function best in well-lit environments. The latter contains three types that each respond to different wavelengths of light – short, medium, and long. This trichromatic system facilitates the perception of a broad range of colors.
The interplay between these two cell types significantly influences how we perceive contrasts. When contrasting colors are present, the rods help enhance the perception of edges and shapes. The brain receives signals from both rods and cones through bipolar and ganglion cells, which further refine these signals for clarity.
The retina’s ability to provide contrast is not merely based on light and dark areas but also involves the color contrasts that inform our understanding of surrounding objects.
Motion Detection
Motion detection is another vital function of the retina, allowing organisms to react to dynamic visual stimuli swiftly. This capability is energetically important for survival, as it enables the identification of predators and prey. In this context, retinal ganglion cells play an important role. Certain ganglion cells respond preferentially to the direction and speed of motion. Their activation occurs when the photoreceptors detect a change in light intensity over time, translating this change into motion signals.
The specialized cells contribute to understanding the trajectory and speed of moving objects, offering insight into potential interactions with the environment. This motion detection capability has implications not only for daily life but also for the evolution of species. Precise and rapid motion assessment supports more complex behaviors and interactions with the environment.
In summary, the retina's processing abilities encompass intricate functionalities like contrast enhancement and motion detection. These elements underpin the vast visual experiences we encounter daily, displaying the complexity embedded within this relatively small yet powerful structure.
Retinal Health and Disorders
Understanding retinal health and disorders is essential within this article as it underscores the significance of maintaining the retina's integrity for optimal vision. This section aims to discuss prevalent retinal diseases, their characteristics, and how they impact visual perception. Recognizing these disorders can also highlight the need for early intervention and ongoing research.
Common Retinal Diseases
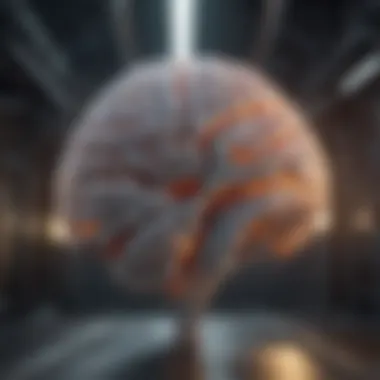
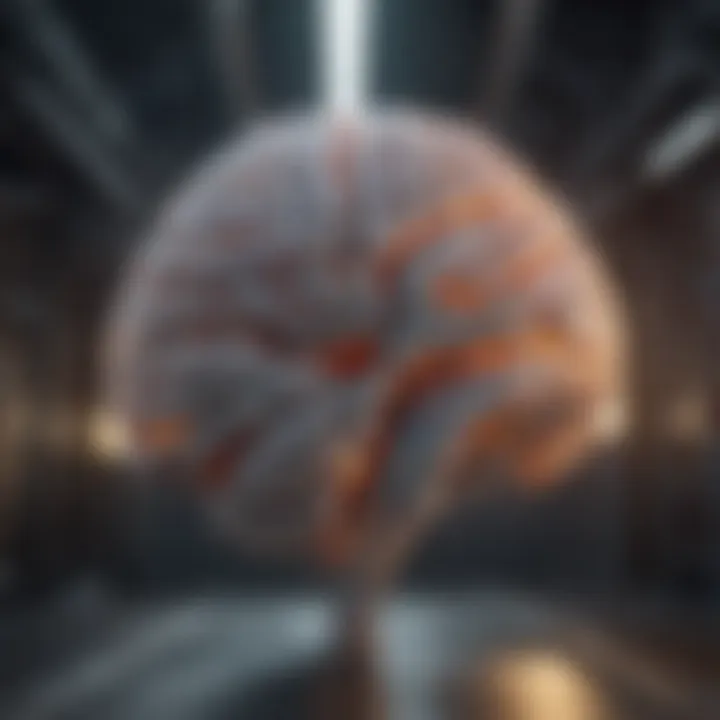
Diabetic Retinopathy
Diabetic retinopathy is a major complication associated with diabetes. It affects the blood vessels in the retina, causing them to swell or leak fluid. One key characteristic of diabetic retinopathy is its potential to lead to vision loss if not addressed. It is a beneficial topic for this article because it reflects the broader implications of chronic diseases on retinal health. The unique feature of diabetic retinopathy lies in its stages, ranging from mild nonproliferative forms to severe proliferative disease, which can result in blindness. Understanding this spectrum is crucial as it reveals the advantages of regular eye examinations for those with diabetes.
Age-related Macular Degeneration
Age-related macular degeneration (AMD) is a common retinal disorder primarily seen in older adults. It is characterized by the deterioration of the macula, an area of the retina crucial for central vision. AMD is a popular inclusion in this article due to its substantial impact on quality of life by impairing detailed vision. A unique aspect of this condition is the distinction between the dry and wet forms, where the wet type involves abnormal blood vessel growth that leads to more significant vision loss. Discussing AMD brings attention to the importance of dietary and lifestyle choices in its prevention.
Retinal Detachment
Retinal detachment occurs when the retina separates from the back of the eye. This can lead to irreversible vision loss if not treated promptly. One key characteristic of retinal detachment is that it is often painless, making it a deceptive condition for many patients. Its inclusion in this article is significant because it emphasizes the urgent nature of certain retinal conditions. A unique feature of retinal detachment is its potential causes, which can include trauma or underlying retinal diseases. This adds to the conversation about the need for awareness and proactive measures for maintaining retinal health.
Impact of Retinal Disorders on Vision
Retinal disorders significantly affect vision quality and overall health outcomes. Common symptoms may include blurred vision, color changes, or even loss of central or peripheral vision. These conditions typically disrupt how visual information is processed. By recognizing these impacts, it becomes clear how important retinal health is in preserving one's quality of life. Advances in treatments and management strategies continue to evolve, reflecting the importance of ongoing research in this area of vision science.
"Early detection of retinal disorders can greatly prevent irreversible vision loss."
Advancements in Retinal Research
Advancements in retinal research have spurred significant progress in understanding and addressing various retinal diseases. The retina's complexity necessitates ongoing studies that delve into its structure and function. Innovations in this field promise not only to unveil the fascinations of human vision but also to offer solutions for conditions that lead to vision loss. This section discusses two main focal points of advancement: innovative imaging techniques and gene therapy approaches, each representing the frontier of research in retinal health.
Innovative Imaging Techniques
Innovative imaging techniques have dramatically transformed the landscape of retinal research. Methods such as optical coherence tomography (OCT) allow researchers and clinicians to visualize the retinal structure in real time, providing high-resolution images of the retina's layers and pathology. This technique is pivotal in diagnosing and monitoring diseases such as diabetic retinopathy and macular degeneration.
Another advancement involves the use of fundus photography, which works hand-in-hand with OCT. This method captures detailed images of the retina's surface, enabling a clearer view of any abnormalities that may arise. Furthermore, adaptive optics imaging permits researchers to assess the photoreceptor cells with precision, enhancing our understanding of their role in visual function.
The integration of artificial intelligence (AI) in retinal imaging is also noteworthy. AI algorithms analyze images, detecting subtle changes that may escape the human eye. This capability not only aids in quicker diagnoses but also enhances the accuracy of retinal assessments. The enhanced imaging modalities enable earlier interventions and more personalized treatment plans, promising improved outcomes for patients.
Gene Therapy Approaches
Gene therapy approaches represent a groundbreaking shift in how we address retinal disorders. By targeting the genetic origins of diseases like retinitis pigmentosa and Leber congenital amaurosis, gene therapy aims to correct or replace defective genes within the retinal cells. This method is still in the experimental stages, but the initial results have been promising, holding the potential to restore vision in patients with previously untreatable conditions.
Recent trials have demonstrated the ability to administer the therapeutic genes through techniques such as subretinal injections. This targeted approach installs the corrected gene directly within the retinal environment, maximizing the chances of effectiveness.
The implications of gene therapy go beyond individual conditions. They open a pathway for understanding genetic diseases' complexities at a broader level. As research continues, it paves the way for future strategies, including combination therapies where gene therapy is used alongside drugs and other interventions.
"Advancements in retinal research not only enhance our understanding of the visual system but also bring new hope to those suffering from vision impairment."
The End: The Future of Retinal Studies
The exploration of retinal studies is crucial in understanding vision science and its complexities. The retina is not just a passive receptor of light; it is an intricate structure that performs multifaceted functions to ensure visual perception. Recognizing this complexity opens up new avenues for research and practical application in medicine and technology.
Implications for Vision Science
Research into the retina holds immense implications for vision science. Advances may lead to breakthroughs in treating and managing diseases such as diabetic retinopathy and age-related macular degeneration. By understanding the underlying mechanisms of these conditions, scientists stand to enhance diagnostic methods and develop more effective treatments.
Additionally, the study of the retina can shed light on visual processing and perception. Insights gained could inform the development of assistive devices for individuals with visual impairments. Such devices could be tailored to compensate for specific retinal dysfunctions, thereby improving the quality of life for many.
Ongoing research continues to reveal the retina's role in higher visual functions, such as attention and cognition. This knowledge may reshape our understanding of how visual information is integrated and utilized by the brain.
The Role of Ongoing Research
The significance of continual research in retinal studies cannot be overstated. It propels us closer to understanding various retinal disorders, genetic mutations, and environmental factors impacting eye health. Innovative imaging techniques and gene therapy methods are paving the way for promising results in patient care.
Here are some notable areas where ongoing research is essential:
- New Therapeutic Approaches: Development of gene therapies that may provide a cure or effective management strategies for retinal disorders.
- Advanced Diagnostic Tools: Innovations in imaging technologies can lead to early detection of retinal diseases, improving outcomes.
- Understanding Disease Mechanisms: Research into the cellular and molecular basis of retinal diseases enhances our ability to create targeted interventions.
Ongoing studies also foster collaboration between multidisciplinary teams. This partnership between biologists, clinicians, and technologists ensures a holistic approach to problem-solving in retinal health.
"The future of retinal research is not merely about understanding a layer of cells, but about unlocking the full potential of the visual system and its impact on human experience."
In closing, the future of retinal studies promises significant advancements in health care and technology. As we continue to invest in this vital area of vision science, we pave the path toward revolutionary discoveries that can improve countless lives.