Trizol Plant RNA Extraction: Comprehensive Guide
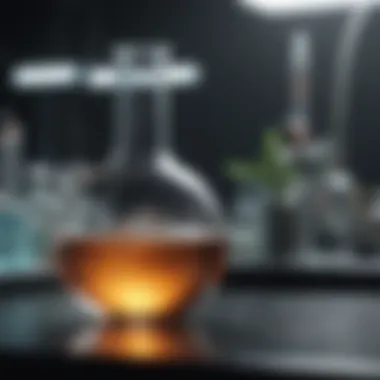
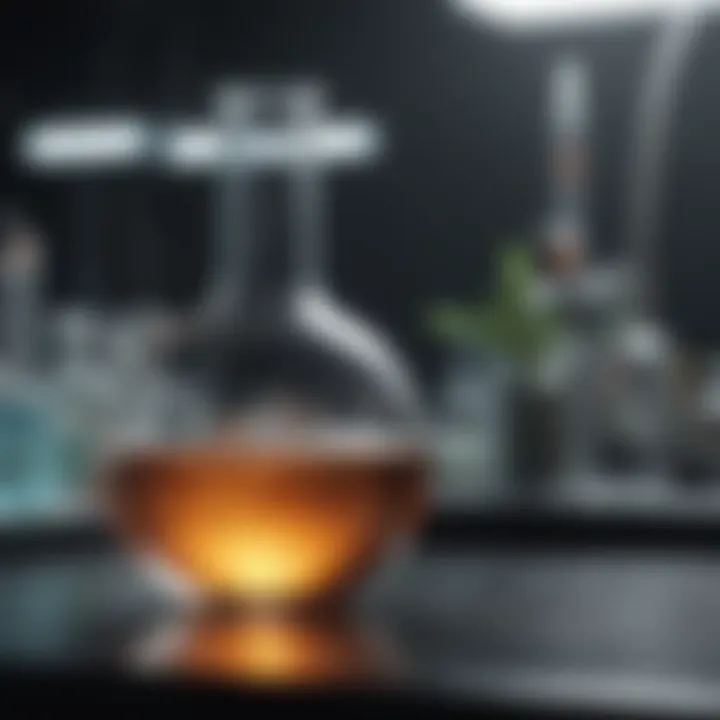
Intro
RNA extraction from plant materials can seem as formidable as navigating a labyrinth, yet it plays a crucial role in the expansive field of molecular biology.
Among the myriad of techniques available, Trizol reagent stands out for its efficiency and effectiveness. Proficient understanding of this method is key for anyone—be it students, researchers or professionals—seeking insights into plant biology and biotechnology.
In this guide, we'll navigate through the intricacies of utilizing Trizol for plant RNA extraction. From outlining the fundamental principles of RNA stability to delving into the step-by-step extraction processes and acknowledging the potential challenges, we'll aim to provide a comprehensive overview.
Let’s dive directly into the methodology that underpins this influential technique.
Methodology
Study Design
The study design for RNA extraction typically relies on a systematic laboratory setup in which raw plant material is selected and prepared. Factors such as the age and health of the plant can significantly impact the quality of RNA extracted. Careful consideration of these parameters ensures the results are not just substantial, but reproducible across experiments. It is advisable to select fresh tissue; they'll serve as a treasure trove of high-quality RNA.
Data Collection Techniques
The Trizol extraction protocol itself is relatively straightforward, consisting of the following key steps:
- Tissue Homogenization: Start by mechanically disrupting plant tissue in Trizol. This ensures thorough cell lysis, which is critical for effective RNA extraction.
- Phase Separation: Next, the homogenate is subjected to chloroform addition followed by centrifugation. This separates the mixture into distinct phases—RNA, DNA, and proteins are found in different layers.
- RNA Precipitation: The aqueous phase, containing RNA, is carefully collected and precipitated using isopropanol. Care must be taken to avoid contamination with the other cellular components.
- Washing and Resuspension: Finally, the harvested RNA is washed with ethanol to remove residual impurities and then resuspended in a buffer such as RNAse-free water for storage.
This methodology emphasizes the significance of precision, as even minor deviations can lead to subpar RNA quality.
"Quality RNA is the backbone for downstream applications, underscoring its role in the synthesis of cDNA and subsequent amplification techniques."
Potential Pitfalls
While the aforementioned steps outline a solid foundation for RNA extraction via Trizol, there are common pitfalls to be wary of. Often overlooked factors include:
- Sample Contamination: Use clean and RNase-free equipment.
- Inconsistent Tissue Processing: Ensure uniformity in tissue grinding to avoid variation in yield and quality.
- Temperature Control: RNA is sensitive to degradation. Keeping samples on ice throughout the protocol can markedly enhance stability.
In tackling these challenges, researchers can greatly enhance the reliability and reproducibility of their results.
Summary
The methodology of RNA extraction using Trizol is vital for those who wish to understand and apply biotechnology in research settings. It blends precision with simplicity, yet demands careful attention to detail. Each step can provide insight into not just the RNA itself, but the biological intricacies of the plant being studied.
Having laid a solid groundwork, it’s equally pertinent to interpret and discuss the implications of the results, keeping in view the future trajectory of research in this domain.
Intro to RNA Extraction
RNA extraction is a fundamental technique in molecular biology that provides insight into the genetic expression and functionality of organisms, particularly in plants. Understanding the process of extracting RNA is crucial for researchers aiming to study gene expression, developmental biology, and plant stress responses. In essence, RNA serves as a bridge between DNA and proteins—it's the messenger that conveys genetic instructions. This makes its extraction not just a lab procedure but a vital step for myriad applications in biotechnology and research.
Importance of RNA in Plant Biology
The significance of RNA in plant biology cannot be overstated. RNA molecules are involved in various essential cellular processes, including protein synthesis, gene regulation, and metabolic pathways. Among the various types of RNA, messenger RNA (mRNA) plays a pivotal role, as it transmits genetic information from DNA to ribosomes, the cellular machinery responsible for protein production. In addition to this, RNA silencing mechanisms such as microRNAs engage in regulatory functions that crucially impact plant development and stress responses. Therefore, studying RNA offers profound insights into how plants adapt to environmental stimuli, how they develop, and how they can be improved for agricultural purposes.
"The DNA of plants dictates their potential, but RNA is the storyteller that weaves that potential into reality."
Moreover, considering that plants often respond to external stressors—like drought or pest infestations—by altering their gene expression, the ability to extract and analyze RNA can be indispensable for establishing plant resilience strategies. What emerges is a complex interplay between RNA molecules that guide physiological changes, making RNA extraction a key player in plant research and development.
Overview of RNA Extraction Techniques
When it comes to extracting RNA from plant tissues, various techniques are employed, each with its unique methodologies and advantages. Some common methods include:
- Phenol-Chloroform Extraction: A time-honored approach involving the use of organic solvents to separate RNA from proteins and other impurities. This method, while effective, can be hazardous if not managed properly.
- Column-based Kits: These commercial kits offer a user-friendly format for RNA extraction, utilizing silica membranes to capture RNA. They significantly reduce the risk of contamination and often provide consistent yields.
- Magnetic Bead-based Methods: This emerging method uses magnetic beads to isolate RNA quickly. The process can be automated, making it suitable for high-throughput applications.
- CTAB Extraction: Particularly favored for plants with high polysaccharide content, this method utilizes cetyltrimethylammonium bromide to yield cleaner RNA.
Each technique has its pros and cons, and the choice often depends on the type of plant material, the scale of extraction, and the intended downstream applications.
For many applications in research, utilizing Trizol reagent has become increasingly popular due to its efficiency and reliability, as we will explore in greater detail in subsequent sections.
Understanding Trizol Reagent
In the world of plant RNA extraction, Trizol reagent holds a pivotal position. Its popularity stems from its efficiency in isolating high-quality RNA from diverse plant tissues, making it a go-to choice for many researchers. This section is crucial as it lays the foundation for grasping how Trizol operates, making the subsequent processes of RNA extraction comprehensible. When one understands the nuances of how Trizol works, it enhances the overall extraction process, giving researchers better outcomes.
Chemical Composition of Trizol
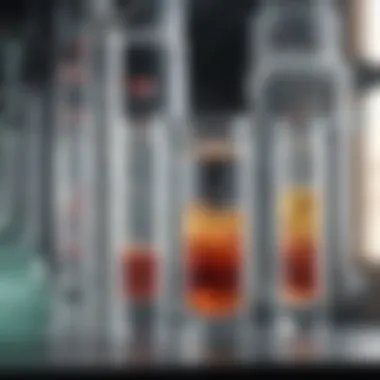
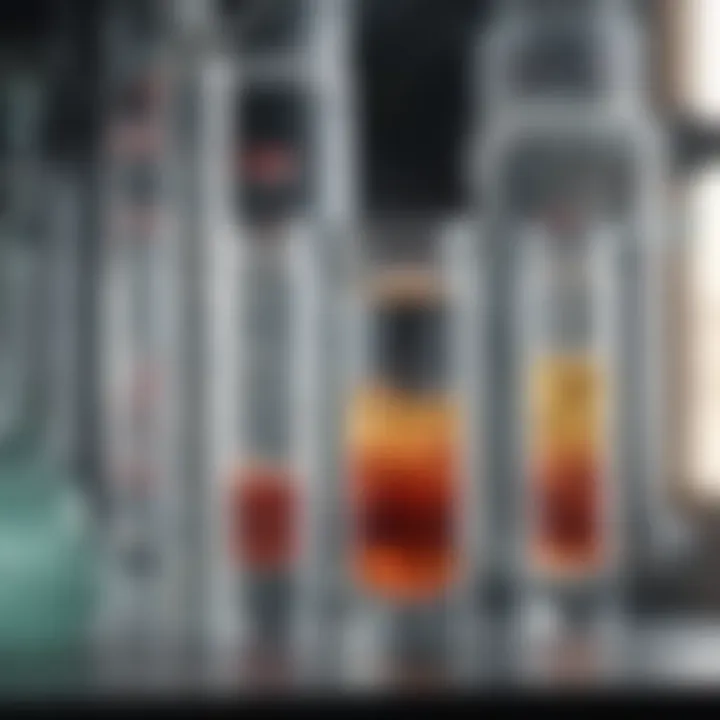
Trizol is a powerful reagent primarily made up of three components: guanidine thiocyanate, phenol, and isopropanol.
- Guanidine thiocyanate: This is a chaotropic agent, which plays an essential role in disrupting the structure of nucleic acids and proteins. This agent allows for the efficient lysis of cells, paving the way for further processes.
- Phenol: It’s a solvent that not only provides separation between RNA, DNA, and proteins but also aids in the purification of nucleic acids. Using phenol allows researchers to segregate RNA cleanly from other cellular components.
- Isopropanol: This component is vital in the precipitation of RNA, ensuring that it is concentrated enough for analysis.
The combination of these elements leads to a robust protocol that many have adopted. Though one should keep in mind that proper handling is necessary because these reagents can be hazardous.
Mechanism of Action
The working of Trizol reagent can be summarized through a sequence of efficient biochemical reactions. When plant samples are ground and mixed with Trizol, the following occurs:
- Cell Lysis: The reagent facilitates the breakdown of cell membranes, allowing the release of RNA, DNA, and proteins from cells. This is where the chaotropic properties of guanidine thiocyanate come into play.
- Phase Separation: Upon adding chloroform to the Trizol/plant mixture and centrifuging, the solution separates into three distinct phases: an aqueous phase contains RNA, an interphase contains DNA, and a phenol phase contains proteins. Understanding this step is crucial for successful RNA isolation.
- Precipitation of RNA: The aqueous phase is carefully collected, and isopropanol is added. This causes RNA to precipitate out of solution, making it visible as a white pellet upon subsequent centrifugation.
- Washing and Resuspension: After the RNA pellet is washed with ethanol, it can be resuspended in an appropriate buffer, preparing it for downstream applications.
Trizol provides a streamlined method for extracting RNA, allowing researchers to focus more on analyzing their samples rather than troubleshooting inefficient extraction processes. With its three-step separation, Trizol ensures a high degree of purity and yield in RNA extraction.
"Understanding the chemical makeup and mechanism of Trizol is central to mastering RNA extraction processes effectively."
Given its efficiency, understanding Trizol reagent is not just about its components; it’s about recognizing its role as a facilitator in the broader landscape of molecular biology and plant research.
Preparation for RNA Extraction
Preparing for RNA extraction is not just a preliminary step; it's an essential process that greatly influences the yield and integrity of the extracted RNA. Effective preparation allows researchers to minimize errors during the extraction protocol, ensuring that quality RNA is obtained for downstream applications. By focusing on specific elements within this phase, one can set a solid foundation for successful RNA work.
Selecting Plant Material
The choice of plant material is crucial in RNA extraction, as it directly impacts the quality and quantity of RNA that can be extracted. Different species, tissues, and developmental stages contribute to varying RNA yields and integrity. When selecting plant material, consider the following:
- Health of the Plant: Ensure that the plant is healthy, as stress or disease can affect RNA quality.
- Plant Tissue Type: Different plant parts (leaves, roots, seeds, etc.) have distinct RNA profiles. Fresh, young leaves often yield high-quality RNA, while woody tissues may present challenges due to their dense cell structure.
- Environmental Factors: Environmental conditions such as temperature and humidity can influence RNA stability. Harvesting during optimal conditions can lead to better RNA integrity.
- Storage Considerations: If immediate extraction isn't possible, proper storage is vital. Freezing samples in liquid nitrogen or storing at -80°C minimizes RNA degradation until extraction can occur.
The strategic selection of plant material can streamline the extraction process, ensuring that you get the best possible RNA for your research needs.
Equipment and Reagents Needed
Having the right equipment and reagents on hand is another crucial step in preparing for RNA extraction using Trizol. Below is a comprehensive list of what you might need:
Equipment
- Mortar and Pestle: For homogenizing plant material, ensuring that cells are broken down properly to release RNA.
- Centrifuge: A refrigerated centrifuge can help in the phase separation process.
- Pipettes and Tips: Accurate pipetting is crucial to measure out reagents precisely; using sterile pipette tips is also important to avoid contamination.
- Vortex Mixer: This ensures that the Trizol is mixed thoroughly with the plant sample for uniform extraction.
Reagents
- Trizol Reagent: The primary agent used for RNA extraction, enabling phase separation crucial for isolating RNA.
- Chloroform: Often used for the phase separation process to further purify the RNA.
- Ethanol or Isopropanol: Required for the precipitation of RNA from the aqueous phase.
- 75% Ethanol: For washing the RNA pellet to remove impurities.
"Proper preparation is half the battle won in RNA extraction. Pay attention to the details, and the results will affirm your efforts."
Before beginning the extraction, ensure that all equipment is free of RNases, as these enzymes can degrade RNA during the process. Idealy, working in an RNase-free environment will safeguard your extracted material, enhancing the reliability of your subsequent analyses.
In summary, thorough preparation is indispensable for successful RNA extraction. By judiciously selecting your plant material and gathering the right equipment and reagents beforehand, you set the stage for a smooth extraction process that maximizes RNA yield and integrity.
Comprehensive Protocol for RNA Extraction
The extraction of RNA from plant tissues is a task often fraught with challenges. However, a comprehensive protocol can significantly demystify this process, ensuring quality and reliability in results. This section will illuminate the intricacies involved in each phase of RNA extraction, detailing the actions required for successful outcomes. Implementing a structured approach not only facilitates the extraction but also maximizes the purity and yield of the RNA obtained. A well-crafted protocol ultimately serves as a foundation for downstream applications, emphasizing the importance of each step in the extraction process.
Sample Homogenization
One of the first and crucial steps in RNA extraction is sample homogenization. This often overlooked yet critical process ensures that the plant material is adequately broken down to facilitate effective extraction.
When homogenizing, the goal is to disrupt the cellular structures, thus releasing RNA into the extraction buffer. Various methods can be employed here, such as using a mortar and pestle, bead mills, or homogenizers. Each method has its nuances, and the choice depends on the tissue type and the desired yield.
- Mortal and pestle: A traditional method for softer plant tissues. One must be cautious to work in chilled conditions to prevent RNA degradation.
- Bead mills or homogenizers: Very efficient for tougher tissues. This equipment uses small beads to mechanically break down the sample, which can yield higher amounts of RNA.
The key consideration here is to ensure that the sample remains on ice during the entirety of the homogenization process. RNA is highly sensitive to degradation, particularly by RNases, and maintaining a low temperature is paramount.
Phase Separation
Following sample homogenization, the next step is to achieve phase separation. This step involves the use of Trizol, which, upon mixing with the homogenized plant sample, facilitates the partitioning of RNA, DNA, and proteins into distinct phases.
The process generally requires:
- Addition of Trizol: This reagent interacts with the cellular material, creating conditions conducive for separation.
- Incubation: Allowing the mixture to sit at room temperature for a short period assists in the complete lysis of the sample, enhancing the separation efficiency.
- Centrifugation: This is where the magic happens. Centrifuging the mixture causes the formation of three layers—the aqueous phase that contains RNA, an interphase with DNA, and a lower organic phase.
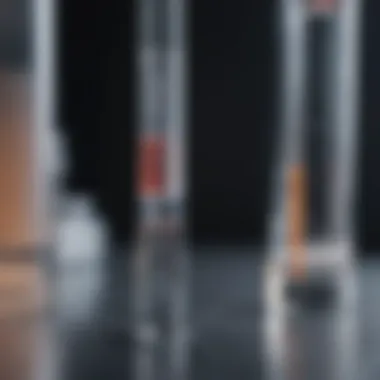
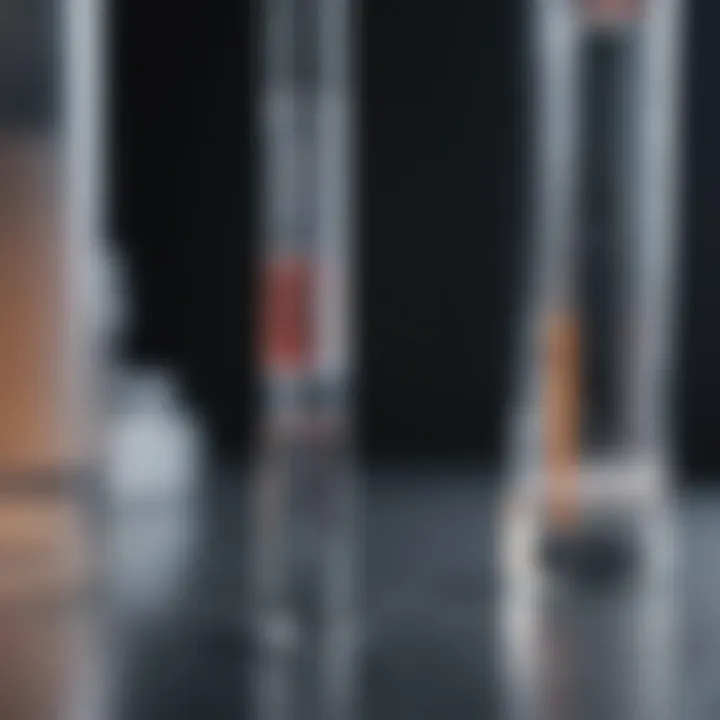
This clear demarcation allows for the careful extraction of the aqueous layer, though it’s imperative to avoid contamination with the other phases. This phase separation is crucial for obtaining high-quality RNA.
Precipitation of RNA
The third step, precipitation of RNA, is where the actual isolation occurs. After carefully extracting the aqueous phase, isopropanol or ethanol is added to facilitate the precipitation of RNA.
Here’s how it typically goes:
- Adding isopropanol: This step requires careful mixing to ensure the RNA precipitates out of solution. Gently inverting the tube allows for solubilized RNA to coalesce into a visible pellet.
- Incubation: Letting the mixture sit at low temperatures stimulates the formation of the RNA pellet, with a usual incubation time of 30 minutes at -20°C.
- Centrifugation: The final step to collect the RNA, after which a pellet should be visible at the bottom of the tube.
This is a delicate process since handling the sample gently is crucial to prevent RNA breakage. After precipitation, the next stage requires washing the pellet to eliminate remaining impurities.
Washing and Resuspension
This final step, washing and resuspension, is vital for ensuring the integrity of the extracted RNA. After the RNA pellet has been collected, it undergoes washing with alcohol to remove any contaminants.
Here are the typical procedures followed:
- Washing with Ethanol: Once the pellet has formed, it should be washed with cold ethanol. This helps to clean the RNA of salt and other residuals that may interfere with later applications.
- Centrifugation: The washed pellet is then centrifuged again, and the supernatant is carefully removed to avoid disrupting the pellet.
- Resuspension: Finally, the pellet should be resuspended in RNase-free water or a suitable buffer. This step is crucial as it determines the final concentration and solubility of the RNA.
By resuspending the RNA properly, researchers can ensure its usability for various downstream applications, such as gene expression analysis and cloning.
In summary, navigating through the comprehensive protocol of RNA extraction using Trizol requires careful execution of each phase. From proper sample homogenization to effective washing and resuspension, understanding these steps can greatly aid researchers in obtaining high-quality RNA for their studies.
Quality Control of Extracted RNA
In the complex world of molecular biology, ensuring the quality of extracted RNA is tantamount to success in subsequent experiments. It’s not just about getting the RNA out of plant material; it’s about making sure that what you’re working with is pure, intact, and suitable for rigorous analysis. This section will explore the significance of RNA quality control, the specific methods for assessing RNA integrity, and the techniques for quantifying extracted RNA.
Assessing RNA Integrity
One of the first steps in quality control is checking the integrity of the extracted RNA. This is crucial, as fragmented RNA can lead to unreliable results in downstream applications, such as RT-PCR or sequencing. There are a handful of widely accepted methods to evaluate RNA integrity.
- Bioanalyzer or TapeStation: These instruments provide a visual representation of RNA quality via electrophoretic separation. They can give you an RNA Integrity Number (RIN), which ranges from 1 (completely degraded) to 10 (intact). A typical standard for quality RNA used in many applications is a RIN of 7 or higher.
- Agarose Gel Electrophoresis: An older, yet effective method, agarose gel analysis allows visualization of RNA bands. Ideal RNA exhibits sharp bands, especially the ribosomal RNA (rRNA) bands. If the bands are smeared or faint, this can be indicative of degradation.
- Spectrophotometry: With this method, you can assess the purity of RNA by measuring the absorbance at 260 nm and 280 nm (A260/A280 ratio). A ratio between 1.8 and 2.0 signifies good RNA purity.
Regular assessment of RNA integrity will save you from falling into the pit of unreliable data later on. The mantra here is simple: assess and address before you dig deeper into your experiments.
Quantification Techniques
Once you’ve established that your RNA is intact, the next logical step is quantification. The measurement of RNA concentration is essential not just to ensure that you have enough material for your experiments but also to maintain batch-to-batch consistency. Several quantification techniques stand out:
- Nanodrop Spectrophotometer: This is one of the most popular methods for quantifying RNA. It requires only a small volume of sample and provides a quick readout of RNA concentration. However, it can be sensitive to contaminants, so use it in conjunction with other methods for the most reliable results.
- Fluorometric Methods: Using DNA-binding dyes such as RiboGreen or Qubit assays, these methods offer high sensitivity and specificity for RNA. They are an excellent choice when working with low concentrations of RNA or when precise quantification is needed.
- PCR-based Approaches: Though less traditional, some researchers have begun to turn to quantitative RT-PCR as a means of quantifying RNA. This method is particularly useful when looking to quantify a target RNA transcript directly.
The right choice of quantification technique can enhance the reliability of your results and ensure that subsequent experiments are as robust as possible.
"Quality over quantity is the mantra of scientific integrity."
In summary, quality control in RNA extraction is a cornerstone of successful molecular biology research. It prohibits wasted time and resources by ensuring that only high-quality, intact, and accurately quantified RNA is used for downstream analyses. When you pay attention to these details, the fidelity of your experimental output increases exponentially.
Common Issues in RNA Extraction
RNA extraction, while a vital process for understanding plant biology, presents various challenges that can significantly affect the quality of the resulting RNA. Identifying and addressing these common issues is crucial for researchers aiming to obtain high-quality RNA for downstream applications. Here, we explore two major problems: RNA degradation and inhibition of downstream applications, outlining their implications and offering strategies to mitigate them.
RNA Degradation
One of the biggest concerns during RNA extraction is degradation. RNA is inherently unstable and can easily break down due to ribonucleases, also known as RNases, which are often ubiquitous in the environment. These enzymes can degrade RNA relatively quickly, often within minutes if proper precautions are not taken.
To combat RNA degradation, researchers should prioritize several key practices:
- Use RNase-free equipment: Tools and reagents should be designated for RNA work and treated to eliminate any potential RNases.
- Incorporate inhibitors: Utilizing RNase inhibitors can be a game-changer, providing an extra layer of protection while handling plant tissues.
- Keep samples cold: Storing and processing samples on ice limits enzyme activity significantly. The colder, the better is a good rule of thumb.
Awareness and vigilance in your extraction environment can make a world of difference. If RNA is compromised during extraction, downstream analyses such as RT-PCR or sequencing may yield unreliable data, spurring the need for repeat experiments and wasted resources.
Inhibition of Downstream Applications
Once extracted, the applicability of RNA hinges on its quality. Various components and contaminants can inhibit the effectiveness of downstream applications, leading to erroneous results. Substances such as phenol, ethanol, or even residual Trizol can cripple the performance of assays designed to analyze RNA.
To sidestep these pitfalls, researchers should consider the following measures:
- Thorough washing: Ensuring that RNA is adequately washed during the extraction process reduces the possibility of retaining leftover reagents.
- Purification protocols: Following up the extraction with a purification step, for example, using columns designed for RNA cleanup, can remove contaminants.
- Quality assessment: Regularly checking RNA concentration and integrity via methods such as gel electrophoresis or spectrophotometry helps assess the potential inhibition issues before moving on to experiments.
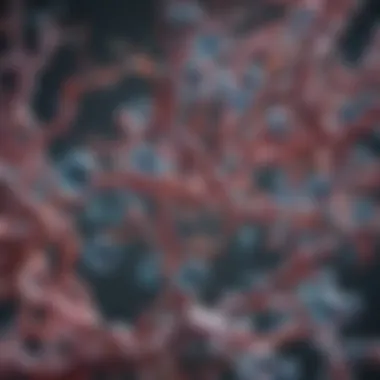
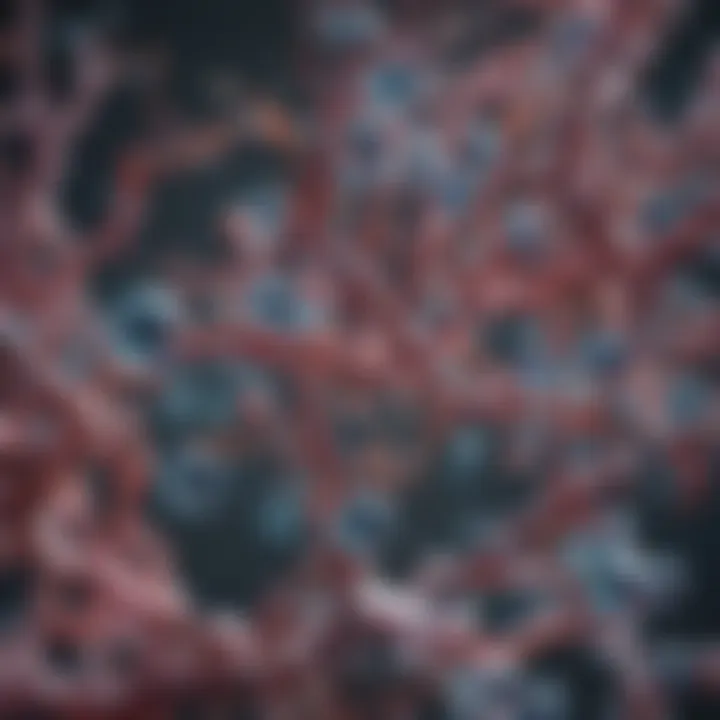
"The success of your extraction lies not only in the protocol but equally in the attention to detail throughout the process."
Applications of Extracted RNA
Extracted RNA plays a crucial role in advancing our understanding of plant biology and facilitating various research undertakings. The utility of this biomolecule stretches far and wide, encompassing numerous applications, each with significant implications in the field of molecular biology, biotechnology, and agricultural sciences. Its versatility is especially relevant when considering gene expression patterns, molecular diagnostics, and genomic studies, establishing it as a cornerstone in modern scientific inquiry.
Gene Expression Studies
One of the primary applications of extracted RNA is in the realm of gene expression studies. By analyzing RNA, researchers can observe which genes are actively transcribed within cells, shedding light on physiological responses to external stimuli or developmental stages. This understanding can enhance our knowledge of fundamental plant processes, like growth, response to stress, and interaction with pathogens.
Researchers often utilize quantitative reverse transcription PCR (qRT-PCR) to measure variations in gene expression levels. This technique provides a powerful approach to identify expressed genes under specific conditions or treatments. However, for accurate interpretation, high-quality RNA must be obtained, free from contaminants that may hinder downstream applications. Moreover, considerations such as the choice of reference genes and biological replicates play a pivotal role in ensuring the reliability of results.
RT-PCR Techniques
Real-Time Polymerase Chain Reaction (RT-PCR) techniques represent another vital application for extracted RNA. These methodologies allow for the amplification and quantification of specific RNA sequences with remarkable precision. Used extensively for both diagnostic and research purposes, RT-PCR serves as a tool to understand disease mechanisms, notably in identifying pathogen presence or quantifying plant responses during stress events.
The process is relatively straightforward but requires meticulous attention to detail. Each step from sample preparation to the reaction setup must be executed with accuracy. The real-time aspect of this technique also opens up the door to analyzing dynamic changes in gene expression over time, offering insights into how plants adapt to their environment. However, the potential for inhibitors in the extracted RNA can affect the efficiency of amplification, which highlights the necessity of using high-quality samples that are meticulously purified to get reliable results.
Next-Generation Sequencing
Another critical application of extracted RNA is in Next-Generation Sequencing (NGS). This approach allows scientists to obtain massive amounts of data about the plant transcriptome. By sequencing the RNA from various conditions, researchers can uncover new insights into gene functions, discover novel genes, and analyze complex regulatory networks.
NGS technology pairs well with RNA extraction protocols, particularly those involving high-quality RNA such as that obtained via the Trizol method. The biggest advantage of NGS lies in its capacity to surpass limitations imposed by traditional sequencing methods; it can analyze the whole transcriptome concurrently, highlighting not only coding RNA but also non-coding RNAs that have been increasingly recognized for their roles in gene regulation.
The depth of information gleaned from Next-Generation Sequencing significantly broadens our understanding of plant functional genomics and contributes to the development of improved agricultural traits.
In sum, the applications of extracted RNA, ranging from gene expression analysis to advanced sequencing techniques, provide invaluable insights that are shaping the future of plant research. Researchers must prioritize the quality of their RNA extractions to ensure accuracy and validity in their findings, as this will ultimately drive progress in understanding plant biology and advancing agri-biotechnology.
Future Perspectives in RNA Extraction
As the realm of molecular biology evolves, the extraction of RNA, particularly from plant materials using techniques like Trizol, becomes increasingly crucial. The future of RNA extraction holds monumental potential for enhancing the efficacy and precision of biological research. From improving extraction methodologies to integrating advanced molecular techniques, the surrounding landscape looks promising. This section delves into the nuances of advancements in extraction methods and the substantive integration with other molecular techniques, both of which aim to bolster research outcomes.
Advancements in Extraction Methods
In recent years, there have been innovative strides in RNA extraction methodologies that aim to optimize yield and purity. Scientists are now looking at various modifications to existing techniques as well as completely novel approaches.
- Automation: Higher throughput capabilities have been introduced, minimizing the manual steps involved, and reducing the possibility of contamination. Methods utilizing robotics can assist in achieving a more consistent RNA yield, which is vital in reproducible research.
- Alternative Solvents: While Trizol remains a standard, there is ongoing exploration into alternative reagents that might be less hazardous. For instance, researchers are testing out phenol-free solutions that could retain RNA integrity without posing significant health risks.
- Microfluidics: This technology enables rapid and precise manipulation of small volumes of fluids. Adapting microfluidic systems for RNA extraction can enhance both speed and efficiency, allowing for real-time monitoring of the extraction process.
Such advancements not only reduce time but also enhance the overall quality of the RNA extracted, minimizing degradation and maximizing overall yield.
Integration with Molecular Techniques
Working with RNA has become intertwined with various molecular techniques, thus expanding the capability to analyze genetic material more effectively. The integration of RNA extraction processes with molecular tools brings forth numerous advantages:
- RT-qPCR: Real-Time Quantitative Polymerase Chain Reaction is a powerful tool for studying gene expression. When coupled with refined RNA extraction techniques, RT-qPCR offers sensitive and accurate quantification, supporting researchers in understanding gene dynamics under different conditions.
- RNA-Seq: Next-Generation Sequencing provides insights into the transcriptome, helping to identify novel genes, alternative splicing events, and gene regulation pathways. High-quality RNA is critical for reliable RNA-Seq results, hence the importance of good extraction methods cannot be stressed enough.
- CRISPR Technology: The burgeoning field of CRISPR gene editing depends largely on effective RNA extraction and synthesis. Utilizing well-extracted RNA can enhance the efficiency and specificity of guide RNAs utilized in this transformative gene-editing technology.
The successful integration of RNA extraction processes with advanced molecular techniques is not just about streamlining workflows; it fundamentally revolutionizes the capacity of researchers to unravel biological complexity.
The future of RNA extraction is not merely about improving existing protocols but is also about embracing a holistic approach that intertwines with various biological disciplines. As these advancements reshape how we approach experiments and analysis, the implication for research could lead to groundbreaking discoveries.
By understanding these nuances, students, researchers, educators, and professionals can better appreciate the significance of robust RNA extraction as they delve deeper into the realm of plant biology.
Culmination
The conclusion of this guide brings the intricate world of RNA extraction into clearer focus. It's not just about extracting RNA; it’s about understanding its significance in plant biology and the various protocols available to researchers. This last section encapsulates the essence of RNA extraction using Trizol, emphasizing critical elements that underscore its value in modern plant research.
Recap of Key Points
To summarize, the importance of RNA extraction cannot be overstated. Here are some essential aspects:
- Trizol Reagent: A robust agent vital in isolating high-quality RNA, ensuring its integrity for downstream applications.
- Methodological Rigor: The significance of following a meticulous protocol to avert common pitfalls that could compromise results.
- Quality Control: The necessity of assessing RNA integrity and concentration to ensure reliable data for subsequent experiments.
- Applications in Research: RNA's role extends to diverse fields like gene expression studies, RT-PCR, and sequencing, highlighting its versatility and significance.
By remembering these key points, researchers can approach their RNA extraction tasks with enhanced clarity and confidence.
Final Thoughts on Research Implications
Looking ahead, the implications of quality RNA extraction are profound. Researchers must recognize that extracting RNA isn't a mere procedural task; it’s foundational to scientific inquiry. Accurate extraction opens numerous doors, from delving into gene expression patterns to unraveling the genetics behind plant resilience against climate change. As our comprehension of plant biology advances, effective RNA methodologies will become even more crucial in our ecological toolbox.
Furthermore, recent advancements in extraction technologies hint at a future where RNA extraction becomes more efficient and user-friendly, mitigating many of the current challenges. In an age where data-driven research thrives, having robust RNA extraction protocols will significantly enhance the reliability and reproducibility of biomolecular investigations.
Thus, as this article draws to a close, it is paramount to take these insights and apply them. The potential for future discoveries hinges on the groundwork laid by quality RNA extraction methods.
"In the realm of plant science, where every nucleotide counts, the ability to extract pristine RNA is an art that fuels discovery."
Leverage this guide as a stepping stone in your research, and remember that excellence in RNA extraction could very well lead to the next groundbreaking discovery.