In-Depth Analysis of PCR Protocol and Taq Polymerase
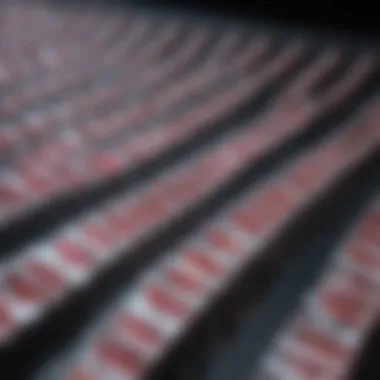
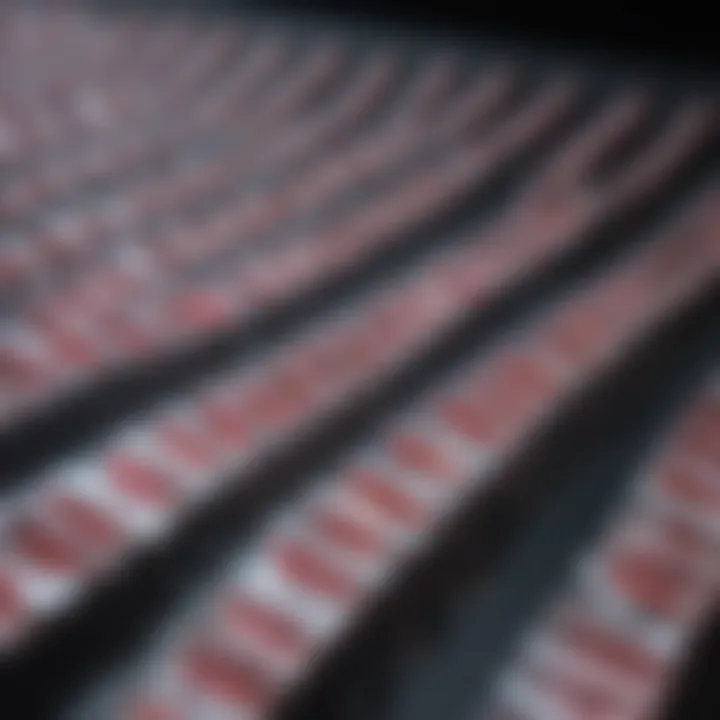
Intro
Polymerase Chain Reaction (PCR) has transformed the field of molecular biology. Its ability to amplify specific DNA sequences has made it an essential technique in various applications, from diagnostics to cloning. Taq polymerase, a thermostable enzyme derived from the bacterium Thermus aquaticus, plays a critical role in the PCR process. Understanding how this enzyme operates and the protocol’s intricacies is vital for researchers and educators alike. This article aims to dissect these components, offering detailed insights into the methodology, challenges, and best practices associated with PCR and Taq polymerase.
Methodology
Study Design
The study of the PCR protocol and Taq polymerase entails a comprehensive review of existing literature and experimental methodologies. This analysis utilizes both qualitative and quantitative data to highlight best practices and common pitfalls in PCR procedures. By examining multiple studies, including those published in peer-reviewed journals, this article aims to provide a clear and cohesive understanding of PCR’s fundamental principles.
Data Collection Techniques
Data for this analysis is collected through various means, including:
- Literature Review: Surveying scientific journals focused on molecular biology and biochemistry.
- Experimental Protocols: Analyzing standard operating procedures (SOPs) for PCR applications.
- Interviews with Practitioners: Gathering insights from active researchers in the field to understand real-world applications and challenges.
By synthesizing these sources, the article encompasses a well-rounded examination of PCR and Taq polymerase, ensuring relevance and depth.
Discussion
Interpretation of Results
The results from the study indicate that Taq polymerase's efficiency can significantly affect the PCR output. Factors such as reaction conditions, primer design, and template quality all play roles in the efficacy of the amplification process. Ensuring optimal conditions can lead to more reliable and reproducible results, which is crucial for scientific rigor.
It is essential for researchers to consider enzyme fidelity and amplification efficiency when designing PCR experiments. Whether for clinical diagnostics or research applications, these factors determine the quality of the results obtained.
Limitations of the Study
While this analysis offers comprehensive insights, it is not without limitations. Variability in experimental conditions, such as differences in laboratory practices and reagent quality, can skew results. Additionally, the focus on Taq polymerase means that other polymerases, which may be more suited for specific applications, are not covered in detail. Future research should aim to explore these alternatives further.
Future Research Directions
To continue advancing the use of PCR and Taq polymerase, future studies could focus on:
- Improving the stability of Taq polymerase and its variants.
- Developing more efficient formulations to enhance amplification.
- Investigating the role of additives in PCR reactions.
By addressing these areas, researchers can broaden the applicability of PCR in diverse biological contexts, ensuring its continued relevance in genomic research.
Preface to PCR
The Polymerase Chain Reaction, commonly known as PCR, is a cornerstone technique in molecular biology. Its significance extends far beyond just the amplification of DNA; PCR has transformed biological research, diagnostics, and forensics. In this section, we will delve into the nuances and intricacies of PCR, elucidating its definition, historical context, and its profound impact on the field of molecular biology.
Definition of PCR
PCR is a laboratory method used to create multiple copies of a specific DNA segment. This process relies on the thermal cycling principle, consisting of repeated cycles of denaturation, annealing, and extension. Denaturation involves heating the DNA to separate its strands, while during annealing, primers bind to the target sequence. Finally, extension occurs when Taq polymerase synthesizes new DNA strands from the primers. In essence, PCR exponentially amplifies the target DNA, which can then be analyzed or utilized in various applications.
Historical Background
The invention of PCR can be traced back to 1983, credited to Kary Mullis, who sought a method to amplify specific DNA sequences efficiently. Before this breakthrough, techniques like cloning were time-consuming and labor-intensive. Mullis's innovation allowed for the rapid amplification of DNA, which opened new frontiers in genetics. The technique has since evolved, becoming more accessible and widespread, aiding numerous fields including medicine, environmental science, and genetic engineering.
Importance of PCR in Molecular Biology
The importance of PCR cannot be overstated. It has become an essential tool in molecular biology laboratories worldwide. It enables researchers to study genes, identify mutations, diagnose diseases, and even trace evolutionary relationships across species. Some benefits include:
- High Sensitivity: PCR can detect minute quantities of DNA, which is crucial in early disease detection.
- Specificity: Targeted amplification means unwanted sequences are not amplified, leading to clearer results.
- Versatility: PCR applications range from medical diagnostics to forensic analysis, illustrating its broad utility.
"PCR has redefined the strategies employed in genetic analysis, enabling precise investigations that were not feasible previously."
Overall, understanding PCR is fundamental for students, researchers, and professionals in the biological sciences. It lays the groundwork for more advanced topics and techniques in molecular biology.
The Role of Taq Polymerase
Taq polymerase is a fundamental component in the Polymerase Chain Reaction (PCR) process. Its role is critical in the amplification of DNA segments, making it an indispensable tool in molecular biology. The enzyme derives its name from the thermophilic bacterium Thermus aquaticus, which is where it was originally isolated. The significance of Taq polymerase can be summarized in several key aspects, including its robust nature, ability to withstand high temperatures, and overall contribution to the efficiency of PCR.
Origins of Taq Polymerase
The discovery of Taq polymerase traces back to the early 1970s when researchers sought to identify heat-stable DNA polymerases. It was identified in the hot springs of Yellowstone National Park, where Thermus aquaticus thrived in extreme heat. This enzyme's ability to function at elevated temperatures distinguishes it from other DNA polymerases, which tend to denature at high heat. The first application of Taq polymerase in PCR was made in 1988 by Kary Mullis, who developed PCR technology, revolutionizing genetic analysis and research in multiple fields.
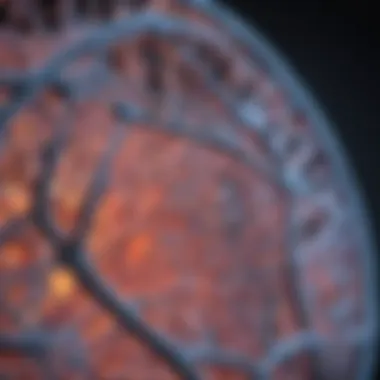
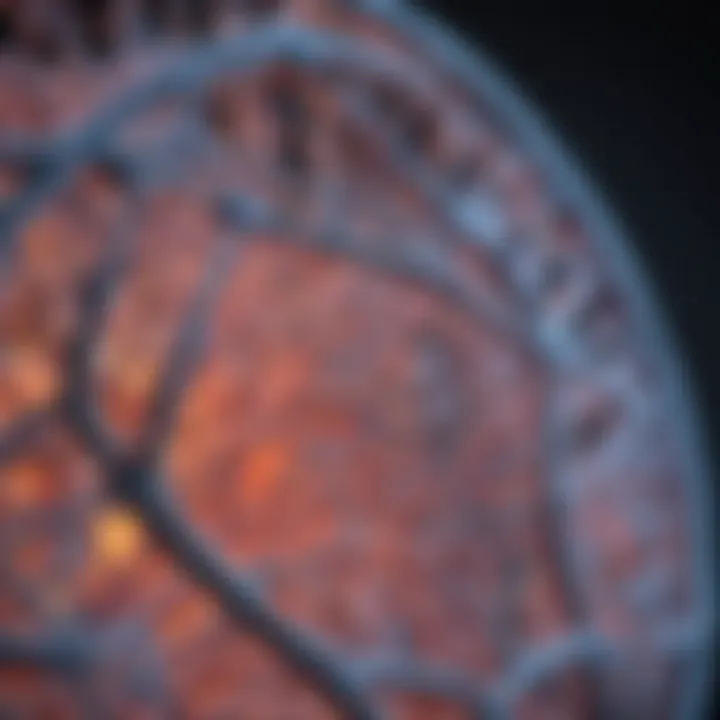
Characteristics of Taq Polymerase
Taq polymerase exhibits several key characteristics that contribute to its efficacy in PCR:
- Thermostability: Taq polymerase can remain active at temperatures around 95°C, which is essential for the denaturation step of PCR.
- 5’ to 3’ polymerase activity: The enzyme synthesizes new DNA strands by adding nucleotides only in the 5’ to 3’ direction.
- Lack of proofreading ability: Taq polymerase does not possess 3’ to 5’ exonuclease activity, meaning it cannot correct errors during DNA synthesis. While this may seem disadvantageous, the rate of DNA amplification primarily compensates for this characteristic, allowing for efficient results in many applications.
- Optimal temperature for activity: The enzyme shows maximum activity at approximately 75-80°C, which is critical for the extension phase of PCR.
Mechanism of Action
The mechanism of action of Taq polymerase during PCR involves several distinct steps. Initially, when the reaction mixture is heated to approximately 95°C, the double-stranded DNA denatures into single strands. Upon cooling, the temperature usually drops to around 50-65°C for the annealing step, allowing primers to bind to complementary sequences on the single-stranded DNA.
Once the primers are in place, the temperature is raised again to the optimal processing temperature set for Taq polymerase. At this stage, the enzyme synthesizes new DNA strands by adding nucleotides to the primers in the 5’ to 3’ direction, effectively extending the DNA strands. With each cycle of heating and cooling, the amount of DNA amplifies exponentially due to Taq polymerase activity, leading to millions of copies of the target DNA sequence.
Taq polymerase's capabilities make it suitable for diverse applications, ensuring consistent results across various experiments.
In summary, Taq polymerase plays a crucial role in PCR by providing the necessary enzymatic activity to amplify DNA sequences. Its origins, unique characteristics, and action mechanism all contribute to its position as a vital tool in molecular biology.
Components of a PCR Reaction
The Polymerase Chain Reaction (PCR) is a cornerstone technique in molecular biology, effectively allowing the amplification of specific DNA sequences. Understanding the components of a PCR reaction is essential for optimizing the protocol and achieving the desired results. Each element plays a specific role, influencing the efficiency and accuracy of the amplification process.
DNA Template
The DNA template is the starting material for any PCR reaction. It provides the specific sequence that researchers aim to amplify. The template can originate from various sources, including genomic DNA, cDNA, or plasmids.
Selecting a high-quality DNA template is critical. Impurities or degraded DNA can inhibit the amplification process and lead to non-specific results. Typically, the concentration of the DNA template should be in a range from 1 to 10 nanograms per microliter. Too much template can cause non-specific binding, while too little can lead to low yield.
Primers
Primers are short, single-stranded oligonucleotides that are essential for PCR. They anneal to the specific target region of the DNA template and provide a starting point for the Taq polymerase to synthesize the new DNA strand. Designing effective primers is crucial for the success of the PCR.
Primers should be complementary to the target sequence, with an optimal length of around 18-24 nucleotides. Mismatches at the 3' end of the primer can greatly reduce amplification efficiency. The melting temperature (Tm) of primers should also be closely matched to ensure they bind effectively under the thermal cycling conditions used in PCR. It is common to use software for primer design to predict these properties accurately.
Nucleotide Triphosphates
Nucleotide triphosphates (dNTPs) are the building blocks of DNA. A typical PCR reaction requires a balanced mix of the four dNTPs: dATP, dTTP, dGTP, and dCTP. These nucleotides provide the necessary substrates for Taq polymerase during DNA synthesis.
Concentration of dNTPs should be around 200 micromolar for each type. An imbalance can lead to incorporation errors or incomplete synthesis. The quality of the dNTPs will also affect the outcome. Poor-quality nucleotides can lead to low yield or artifacts in the PCR product.
Buffer System
The buffer system in a PCR reaction serves to maintain a stable pH and ionic strength, which is vital for enzyme activity and DNA stability. The most commonly used buffer is Tris-HCl, often supplemented with MgCl₂, as magnesium ions are essential for Taq polymerase function.
A typical buffer system should provide a final concentration of 1.5 to 2.5 mM of magnesium. The exact amount may need optimization based on the specific DNA template and primers used. Additionally, pH should be between 8.0 and 9.0 to ensure optimal enzyme activity. Proper buffer conditions can significantly enhance yield and specificity in PCR reactions.
By paying careful attention to the individual components, researchers can optimize the PCR process, increase yield and reduce errors.
Steps of the PCR Process
The Polymerase Chain Reaction (PCR) is a cornerstone of molecular biology, known for its efficiency in amplifying specific DNA sequences. The process itself is a carefully orchestrated series of steps. Each one plays a vital role in the overall success of PCR. Understanding these steps—denaturation, annealing, extension, and cycle repetition—is essential for researchers and practitioners who aim to achieve optimal results in their experiments.
Denaturation
Denaturation is the first step in the PCR cycle. It involves heating the reaction mixture, typically to around 94-98 degrees Celsius. This high temperature serves to disrupt the hydrogen bonds between complementary bases in the DNA double helix. As a result, the DNA strands separate or "denature." This step is crucial because it creates two single-stranded DNA templates, which are necessary for amplifying the target sequence. The efficiency of this step is important. If the temperature is too low, incomplete denaturation can lead to inefficient amplification and low yield.
Annealing
Following denaturation is the annealing step, where the temperature is lowered to allow primers to bind to the single-stranded DNA. The specific temperature for this step usually ranges from 50 to 65 degrees Celsius and depends on the melting temperature of the primers used. During annealing, the forward and reverse primers bind to their complementary sequences on the target DNA template. This step is critical because correct primer binding is necessary for initiating the synthesis of new DNA strands. Failure in this step can result in non-specific binding or no amplification at all.
Extension
The extension phase takes place after annealing. In this step, the temperature is raised again to around 72 degrees Celsius, which is the optimum working temperature for Taq polymerase. This enzyme synthesizes new DNA strands by adding nucleotide triphosphates to the primer-template complexes. The duration of this step varies depending on the length of the target DNA. As the polymerase moves along the template, it constructs complementary DNA strands, leading to the exponential increase of the targeted sequence. The efficiency of Taq polymerase during this step heavily influences the overall yield.
Cycle Repetition
Lastly, PCR involves repeating the cycle of denaturation, annealing, and extension multiple times—usually 20 to 40 cycles. Each repetition doubles the amount of target DNA, creating billions of copies from a single template molecule. This cycle repetition is what gives PCR its exponential amplification. However, it's not as simple as just repeating the cycles. The timing and temperatures for each phase must be optimized to balance efficiency and specificity. A well-optimized cycle can significantly enhance yield and reduce issues like non-specific amplifications.
PCR is a delicate balance between temperature control and reaction timing, crucial for ensuring high-quality DNA amplification.
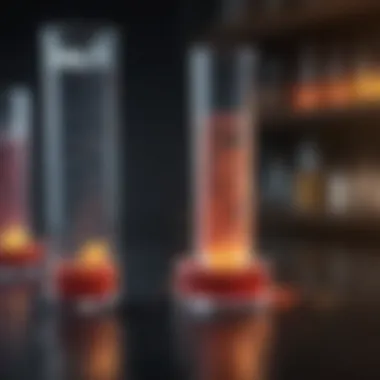
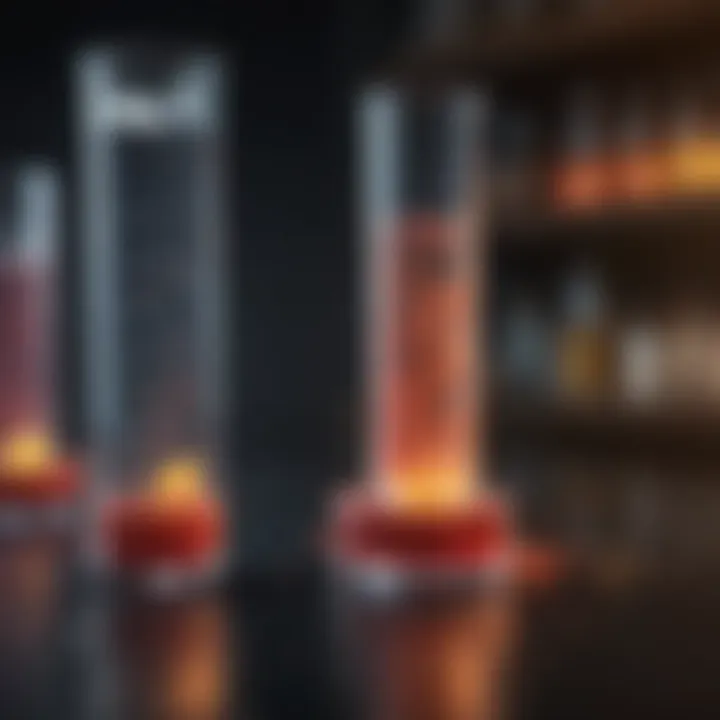
In summary, the steps of the PCR process encompass a sequence of carefully controlled reactions. Each step must be optimized for specific conditions in order to guarantee high efficiency and accuracy in DNA amplification. Understanding these steps in detail equips researchers with the know-how to troubleshoot problems and improve their molecular biology applications.
Factors Influencing PCR Efficiency
The success of the Polymerase Chain Reaction (PCR) heavily relies on several critical elements that can either enhance or hinder the amplification process. Understanding these factors is essential for designing effective experiments. Each stage of PCR can be affected by specific considerations that impact both yield and specificity of the target DNA. Here, we explore the main determinants of PCR efficiency: template quality and quantity, primer design, thermal cycling conditions, and enzyme selection.
Template Quality and Quantity
The quality and quantity of the DNA template serve as the foundation for any PCR process. High-quality DNA template is free from contaminants and degradation, facilitating optimal amplification. If the template is fragmented or contains impurities such as proteins or phenol, it may inhibit the polymerase activity, leading to poor yields.
Furthermore, the amount of template DNA used is also critical. Generally, a range of 1 to 10 nanograms of genomic DNA is sufficient for most PCR reactions. Too little template can result in weak or non-specific bands, while too much can cause non-specific amplification and decrease specificity.
Primer Design
The design of primers has a significant impact on the efficiency of PCR. Primers need to be specific to the target region, and their melting temperatures should be closely matched to ensure proper annealing. Mismatches at the 3' end of the primer can lead to decreased efficiency and non-specific products.
It is advisable to use primers that are 18-25 nucleotides long, with a GC content of 40-60%. This range tends to provide good binding while maintaining stability. Additionally, avoiding secondary structures such as hairpins and dimers will further improve the success of the reaction. Using specialized software for primer design can yield effective and specific primers based on the target sequence.
Thermal Cycling Conditions
Optimal thermal cycling conditions are crucial for successful PCR. The three main phases—denaturation, annealing, and extension—must be carefully balanced in terms of temperature and duration. Denaturation typically occurs around 94-98 degrees Celsius, ensuring template strands separate.
Annealing temperatures generally should be 5 degrees lower than the melting temperature of the primers, allowing them to bind to the template effectively. The extension phase relies on the appropriate temperature for the polymerase used, often reaching 72 degrees Celsius. Adjusting these values according to the specific components in the reaction can yield improved efficiencies and higher fidelity.
Enzyme Selection
Choosing the right DNA polymerase is a fundamental aspect of achieving efficient amplification. Taq polymerase is widely used due to its robustness and ability to function at high temperatures. However, there are alternative enzymes like Pfu polymerase, known for its high fidelity, which may be more suitable for applications requiring superior accuracy.
Factors such as the polymerase’s processivity and error rate should be taken into account when selecting the enzyme. For instance, a high-fidelity polymerase may be necessary for cloning applications, while Taq’s rapid activity is ideal for standard applications where speed is preferred over accuracy.
The choice between speed and accuracy can directly affect the outcomes of PCR, thus understanding the experimental goals is essential.
In summary, meticulous attention to template quality and quantity, precise primer design, carefully optimized thermal cycling conditions, and appropriate enzyme selection are key factors that influence PCR efficiency. By addressing these considerations, researchers can significantly enhance the reliability and effectiveness of PCR in their experiments.
Common PCR Applications
The significance of Polymerase Chain Reaction (PCR) extends into multiple fields, proving itself as a cornerstone technique in molecular biology. Knowing the common applications of PCR is essential for students, researchers, educators, and professionals working in various disciplines. Let us explore the prominent areas of PCR's usefulness, emphasizing how it enhances our understanding of genetics, health diagnostics, forensic science, and evolutionary biology.
Genetic Engineering
PCR plays a pivotal role in genetic engineering. This process allows for the amplification of specific DNA sequences, which is fundamental for introducing desirable traits into organisms. Techniques such as cloning, mutagenesis, and gene expression analysis heavily rely on PCR. For instance, researchers can create transgenic plants with enhanced resistance to diseases or adverse environmental conditions. The precision of PCR ensures that the specific genes of interest are targeted and amplified accurately, minimizing the risk of off-target effects.
Diagnostics
In the medical field, PCR is crucial for diagnosing diseases. It helps detect pathogens by amplifying their DNA or RNA from clinical samples, making it a reliable method for identifying infectious agents. This capability is especially important in detecting viral infections such as HIV or SARS-CoV-2, the virus responsible for COVID-19. The speed and sensitivity of PCR allow for early diagnosis, which is vital for effective treatment and management of diseases. Moreover, it is also used to identify genetic mutations associated with inherited disorders.
Forensic Science
PCR has revolutionized forensic science by enabling the analysis of minute quantities of genetic material from crime scenes. DNA profiling, which is instrumental in criminal investigations, utilizes PCR to amplify DNA samples collected from various sources. This technology can match DNA from suspects to samples found in crime scenes, providing powerful evidence in court. PCR's ability to work with degraded or low-quantity samples makes it invaluable in forensic investigations where conditions may not be ideal.
Research in Evolutionary Biology
In evolutionary biology, PCR serves as a tool to understand genetic relationships among species. Researchers employ PCR to extract and amplify DNA from ancient or rare specimens, allowing them to study phylogenetic relationships. This work helps elucidate evolutionary pathways and adaptations over time. Special protocols, like ancient DNA extraction techniques, rely on the specificity of PCR to recover genetic information that enhances our understanding of biodiversity and evolution.
PCR's broad applications not only advance scientific knowledge but also foster innovations in health, technology, and forensic analysis.
In summary, common PCR applications display the versatility and importance of this technique in various scientific fields. By facilitating genetic engineering, aiding diagnostics, enhancing forensic analysis, and supporting research in evolutionary biology, PCR continues to shape our understanding of molecular biology and its practical implications.
Troubleshooting Common PCR Problems
Successful PCR amplification is often fraught with challenges. Each of these challenges requires an understanding of the underlying processes as well as investigation and adjustment of specific experimental conditions. The significance of addressing common PCR problems lies in their impact on yield and specificity. By understanding these issues and applying the appropriate troubleshooting techniques, researchers can improve the reliability and reproducibility of their results. Below are some of the most frequent challenges encountered in PCR and methods to mitigate them effectively.
Low Yield
Low yield is a common problem that can severely limit the effectiveness of PCR. Several factors can contribute to this issue. First, the quantity and quality of the DNA template play a crucial role. If the template quantity is too low or if it is degraded, amplification will be insufficient. Another consideration is primer design. Poorly designed primers may not bind efficiently, leading to low amplification rates.
To troubleshoot low yield, consider the following steps:
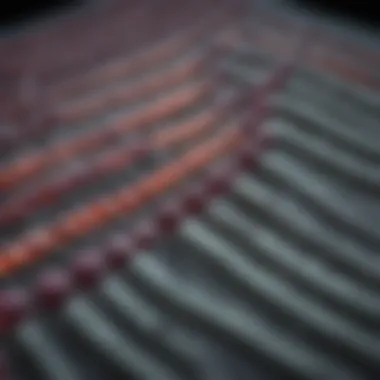
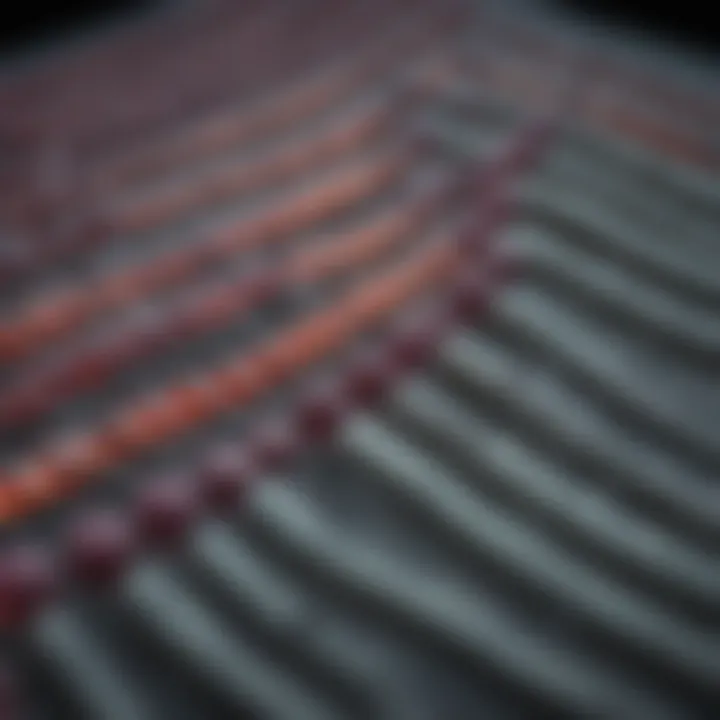
- Increase the amount of DNA template: Use a higher initial concentration of the template to improve yield.
- Check primer quality: Ensure primers are fresh, properly designed, and not degraded.
- Optimize thermal cycling conditions: Adjust parameters such as annealing temperature and time to enhance binding of the primers to the template.
Making these changes may lead to significant improvements in amplification yield.
Non-Specific Amplification
Non-specific amplification occurs when primers bind to unintended sites on the DNA template. This can lead to the formation of unwanted products, affecting the specificity of the PCR. Non-specific products can arise from several factors, including high annealing temperatures or inadequate primer design.
To address non-specific amplification, you can take the following actions:
- Lower the annealing temperature: A slight reduction in the temperature may favor specific primer binding.
- Improve primer specificity: Design primers that are more complementary to the target sequence, and reduce their secondary structures.
- Use a hot start polymerase: This type of polymerase minimizes non-specific binding by remaining inactive at lower temperatures, thus improving specificity when the reaction condustion begins.
These strategies can help to achieve more specific amplification and minimize background noise.
Inhibition of PCR
Inhibition is another major challenge during PCR. Various substances, such as contaminants in the DNA sample or components of the PCR mix, can inhibit the polymerase enzyme's activity and lead to failed amplification. Common inhibitors include proteins, phenol, and alcohol.
To mitigate inhibition:
- Purify DNA samples: Use purification techniques to remove potential inhibitors from the DNA template.
- Diluate the sample: Sometimes, simply diluting the sample can reduce the concentration of inhibitors.
- Optimize enzyme concentration: Increasing the amount of Taq polymerase in the reaction may compensate for some level of inhibition.
By focusing on these strategies, researchers can identify and address the source of inhibition more effectively.
Understanding common PCR problems and their solutions is crucial for optimizing amplification processes and achieving reliable results.
Future Directions in PCR Technology
The field of Polymerase Chain Reaction (PCR) technology is evolving rapidly, driven by advancements in molecular biology. This evolution promises to enhance the efficiency, fidelity, and versatility of PCR processes. As the significance of PCR grows in various domains, including diagnostics and genomics, understanding future directions is essential. Innovations in this area can lead to more streamlined procedures, reduced costs, and improved results in scientific experiments.
Advancements in Polymerase Technology
New developments in polymerase technologies are critical to enhancing PCR. Researchers are focusing on creating enzymes with higher specificity and processivity. The aim is to minimize errors during amplification. As a result, modified versions of Taq polymerase and alternatives, like Phusion and Q5 polymerases, are being explored. These advanced enzymes have properties such as:
- Increased resistance to inhibitors
- Improved performance at high temperatures
- Enhanced ability to amplify complex templates
These advancements allow for more reliable outcomes in difficult conditions. Additionally, the emergence of thermostable polymerases from extreme environments contributes to this progress. They bring new capabilities to PCR, widening the context of applications.
Integration with Next-Generation Sequencing
Integrating PCR with next-generation sequencing (NGS) is a significant trend. This combination allows for seamless amplification and sequencing of DNA, streamlining workflows in genomic studies. In particular, PCR-based NGS can achieve:
- Targeted enrichment of specific DNA regions
- Increased throughput of sequencing data
- Rapid generation of valuable genetic information
The fusion of these technologies means that researchers can extract more information from less sample material. This is particularly critical in fields such as cancer research and personalized medicine, where sample size may be limited. Moreover, improved workflow reduces time and costs while enhancing the quality of data.
Real-time PCR Innovations
Real-time PCR, or quantitative PCR (qPCR), continues to innovate through developments in detection techniques. The shift towards more sensitive and specific assays fosters more accurate quantification of nucleic acids. Innovations include:
- Use of fluorescent probes for real-time monitoring
- Advanced software tools for data analysis
- Enhanced multiplexing capabilities, allowing for multiple targets in a single reaction
These enhancements lead to improved quantitative accuracy and faster results. Moreover, the application of real-time PCR in clinical diagnostics and research projects showcases its growing importance in detecting pathogens and measuring gene expression.
"Through advancements in PCR technology, we can expect more reliable and efficient amplification methods benefiting a wide range of scientific fields."
As we look toward the future, these trends indicate not only improvements within PCR methods but also a broader impact across various areas of research and application, providing significant opportunities and benefits.
Closure
The conclusion of this article encapsulates the essential insights gained from exploring PCR and the pivotal role of Taq polymerase. Understanding these concepts is crucial for anyone involved in molecular biology. The importance lies in their extensive application in various fields, from diagnostics to genetic research. These techniques not only facilitate the amplification of DNA sequences but also enhance the precision of genetic analysis.
For researchers and educators, recognizing the limitations and best practices enables more effective experimental designs. Moreover, ongoing advancements in PCR technology and polymerase selection will likely lead to more efficient and reliable results.
Summary of Key Points
- PCR is a fundamental technique for DNA amplification, revolutionizing molecular biology.
- Taq polymerase, derived from Thermus aquaticus, is an essential enzyme that withstands high temperatures during PCR cycles.
- Choosing appropriate thermal cycling conditions and high-quality reagents can significantly enhance PCR efficiency.
- Various applications of PCR, including diagnostics and forensic science, highlight its importance in real-world scenarios.
- Future developments in PCR technology focus on increasing speed, accuracy, and integration with next-generation sequencing methods.
The Significance of Taq Polymerase in PCR
Taq polymerase stands out due to its heat resistance, making it suitable for the high temperatures needed in PCR denaturation. Its ability to synthesize new DNA strands efficiently under these conditions has made it a cornerstone of molecular biology techniques. By optimizing the usage of Taq polymerase, researchers can improve the specificity and yield of PCR results, contributing to the advancement of genomics.
Overall, the insights discussed in this article provide a solid foundation for comprehending PCR protocols and Taq polymerase's critical functions. The understanding of these elements will certainly foster improved practices in experimental design and reinforce the significant role of PCR in modern science.