Molecular Sieve Chromatography: A Comprehensive Study
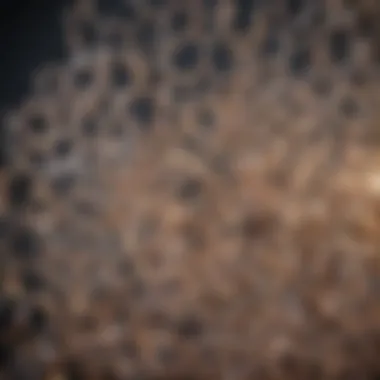
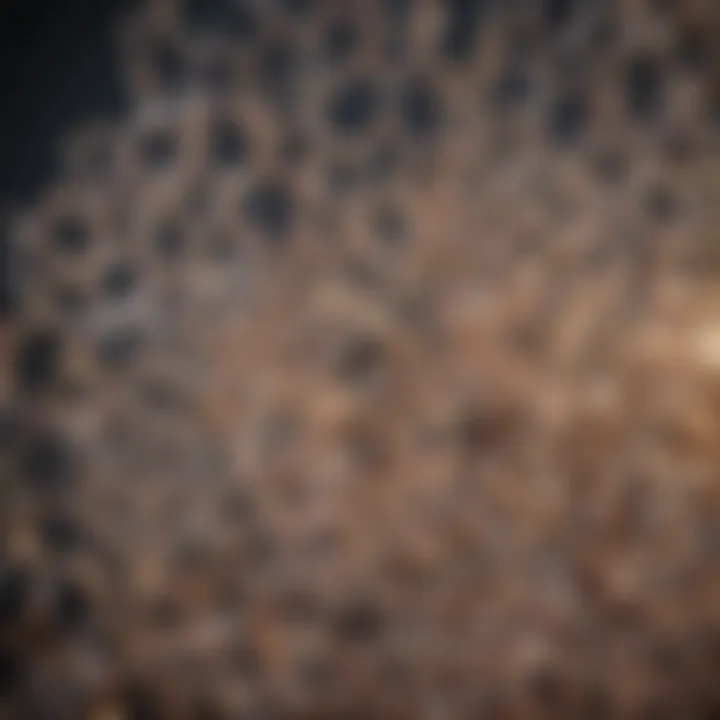
Intro
Molecular sieve chromatography represents a crucial method in separation science. The significance of this technique lies in its wide-ranging applications across disciplines like chemistry and biology. Understanding these methodologies is essential for both practitioners and students who are engaged in analytical work. The article will guide the reader through the fundamental aspects of molecular sieve chromatography, emphasizing its operational principles and various implementations.
Methodology
Study Design
In molecular sieve chromatography, the design often centers on the type of sieve used. These sieves are typically crystalline materials that selectively adsorb molecules based on their size. The research involves the selection of specific materials such as zeolites or silica gel, impacting the efficiency of separation processes.
Another critical element in the study design is the optimization of operating parameters, such as temperature and flow rate, which can greatly influence the separation's effectiveness.
Data Collection Techniques
Data collection in this context primarily revolves around monitoring the effluent from the chromatographic columns. Key metrics include:
- Retention times of the analytes.
- Peak areas from chromatograms, which can be integrated to determine concentrations.
- Comparisons between theoretical and observed results to assess the performance of the sieve used.
Instrumental techniques such as high-performance liquid chromatography (HPLC) and gas chromatography (GC) are often employed to collect and analyze the separation data effectively.
Discussion
Interpretation of Results
The interpretation of results from molecular sieve chromatography allows researchers to understand various factors such as molecular size, shape, and polarity affecting separation capabilities. This understanding can lead to more tailored applications, accommodating specific requirements in chemical or biological analysis.
Limitations of the Study
Despite its advantages, molecular sieve chromatography is not without limitations. The choice of material can sometimes restrict the range of molecules that can be separated. Moreover, the need for rigorous calibration means that variations in experimental conditions may affect reproducibility. These factors must be acknowledged when evaluating the results.
Future Research Directions
Looking forward, there are several promising avenues for future research in molecular sieve chromatography. Potential developments include:
- Advancements in synthetic materials that offer superior selectivity and efficiency.
- Integration of this technique with other analytical methods to create more comprehensive data.
- Further exploration of its applications in pharmaceutical compounds and bioengineering.
"Molecular sieve chromatography not only enhances our understanding of separation mechanisms but also proposes new pathways for research in analytical chemistry."
This in-depth analysis aims to paint a clear picture of molecular sieve chromatography, illustrating its operational depth and future potential in various scientific fields.
Foreword to Molecular Sieve Chromatography
Molecular sieve chromatography is a crucial technique within the realm of analytical chemistry, renowned for its capacity to separate molecules based on size. This method offers significant insights and benefits, not only enhancing the efficiency of analyses but also fostering a deeper understanding of various scientific phenomena. The importance of this topic in this article is underscored by its applications in diverse fields such as biochemistry, medicine, and environmental science. Molecular sieve chromatography provides a platform where molecular interactions can be scrutinized, yielding vital data that can inform research and practical applications.
Definition and Overview
Molecular sieve chromatography is a form of chromatography that employs molecular sieves, materials that selectively allow certain molecules to pass while blocking others based primarily on size. The process relies on porous structures which create a physical barrier that facilitates the separation of different molecular species. The fixed pores in these sieves lead to size exclusion, molecular entities larger than the pore sizes cannot penetrate and are effectively retained, while smaller molecules are able to elude capture and pass through. This property enables the purification and analysis of complex mixtures with intricate molecular profiles.
The technique can be contrasted with other chromatographic methods that may rely on chemical interactions; molecular sieve chromatography is mainly governed by the physical dimensions of the molecules involved. This fundamental principle makes it a versatile tool for various applications that demand high-purity separations.
Historical Context
The development of molecular sieve chromatography is rooted in the broader evolution of chromatography as a scientific discipline. Early forms of chromatography date back to the 19th century with the pioneering work of scientist Mikhail Tsvet, who utilized colored substances to analyze and separate compounds. However, it was not until the mid-20th century that the concept of molecular sieving began to take shape, driven by advancements in material science and the understanding of porous structures.
The invention of zeolites marked a significant milestone in this field. Zeolites, aluminosilicate minerals with unique porous frameworks, were first used in the 1960s for gas separation applications. As researchers recognized their potential for chromatographic separation, methodologies around molecular sieves evolved, paving the way for specific applications in biochemical isolations and further techniques were developed, including the adaptation of silica gel and polymeric materials for sieve applications. This continued evolution reflects how the integration of knowledge across disciplines has enriched molecular sieve chromatography and solidified its position as a key technique in modern analytical chemistry.
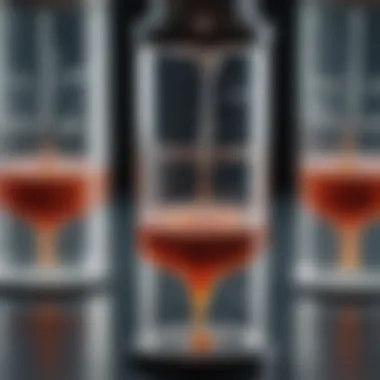
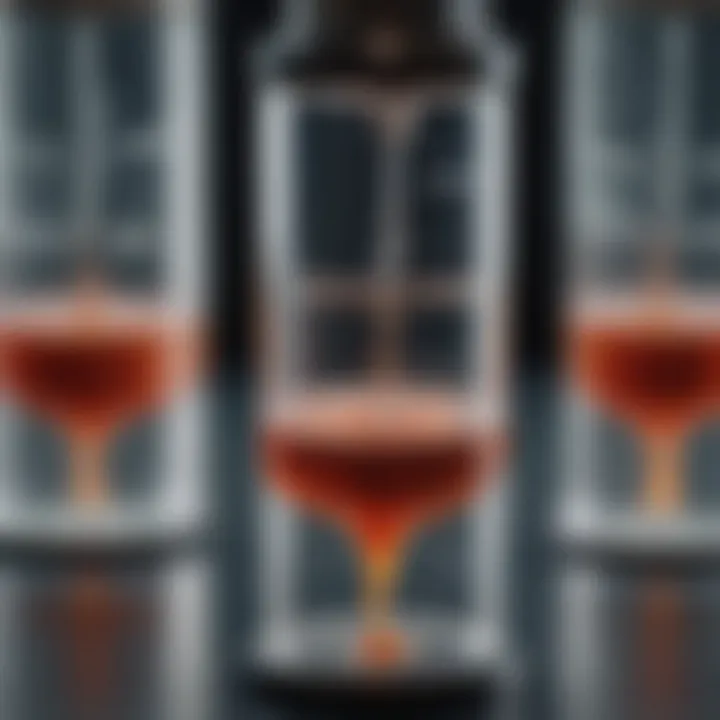
Fundamental Principles
Understanding the fundamental principles of molecular sieve chromatography is vital for grasping how this technique efficiently separates molecules based on size. This section delves into the mechanisms underlying this method, focusing on concepts central to its operation. By knowing these principles, researchers and professionals can enhance their analytical capabilities and refine their methodologies.
Size Exclusion Mechanism
At the heart of molecular sieve chromatography is the size exclusion mechanism. This principle operates on the premise that molecules of various sizes behave differently when passed through a porous medium. The porous medium, often comprised of structured materials like zeolites or silica gels, allows smaller molecules to penetrate its pores while larger molecules are generally excluded. This selective permeability is crucial, as it enables the separation of compounds based on their molecular dimensions.
The size exclusion principle not only maximizes separation efficiency, but it also minimizes interaction with the stationary phase. This is different from other chromatography types, which might separate based on chemical affinity rather than size. The key aspects that define this mechanism include:
- Pore Size Distribution: Varying pore sizes in the material influence how molecules interact during chromatography. Each material, whether it is a zeolite or silica gel, has specific rational pore dimensions tailored for particular applications.
- Molecular Weight Considerations: The larger a molecule, the more likely it will be excluded from entering pores. The method is, therefore, especially suited for the fractionation of large biomolecules, such as proteins, polysaccharides, and lipids.
- Separation Efficiency: The ability to achieve high-resolution separation is a hallmark of molecular sieve chromatography. The sharper the distinctions achievable, the more effective the technique in analytical settings becomes.
"Molecular size and pore characteristics play a critical role in the behavior of compounds during the chromatographic process."
Diffusion and Transport Factors
The processes of diffusion and transport are essential for understanding how molecules behave in the context of molecular sieve chromatography. These factors govern the migration of molecules through the sieve material and significantly affect the overall chromatographic performance.
- Diffusion: In chromatography, diffusion refers to the random motion of molecules within the mobile phase as they travel through the stationary phase. The rate of diffusion directly impacts how quickly a molecule can move. Factors like temperature and viscosity of the mobile phase influence diffusion rates.
- Transport mechanisms: These are concerned with how efficiently molecules travel through the chromatography column. Efficient transport means that the molecules will reach the detector faster, improving the analysis time. Factors contributing to transport include:
- Mass Transfer Resistance: This is a crucial consideration in optimization. Resistance occurs when molecules encounter hurdles exiting the stationary phase back into the mobile phase. Reducing this resistance increases the speed and efficiency of the chromatography process.
- Column length and diameter
- Particle size of the media
- Flow rate of the mobile phase
By comprehending the dimensions of diffusion and transport factors, practitioners can manipulate conditions for optimal separation. Adapting parameters such as flow rates and column integrity can significantly improve the outcomes of their analytical results.
Types of Molecular Sieves
Understanding the various types of molecular sieves is essential in the context of molecular sieve chromatography. Each type has unique properties, advantages, and applications, all of which are crucial in achieving effective separation of compounds. By analyzing the characteristics of zeolites, silica gels, and polymeric materials, researchers and professionals can select the most appropriate materials for their specific needs.
Zeolites
Zeolites are a class of microporous, crystalline aluminosilicates that play a significant role in molecular sieve chromatography. Their unique structure allows them to selectively adsorb molecules based on size and shape. Zeolites contain a network of interconnected pores that can trap smaller molecules while allowing larger ones to pass through. This property makes them highly effective in the separation processes.
The advantages of using zeolites include their high thermal stability and ability to be modified chemically. Researchers can enhance their performance by tailoring the pore sizes or introducing functional groups, thus improving selectivity or affinity for target molecules. Applications often seen in petroleum refining, gas separation, and catalysis highlight zeolites' versatility and efficiency in analytical chemistry.
Zeolites can be synthesized or obtained naturally, offering flexibility in choosing the optimal material for specific applications.
Silica Gels
Silica gels are another popular choice in molecular sieve chromatography. They consist of porous silica particles that provide large surface areas and uniform pore sizes. The primary advantage of silica gels lies in their ability to interact with a wide range of compounds through adsorption processes. This makes them suitable not just for size exclusion but also for affinity chromatography.
In terms of customization, silica gels can undergo various chemical modifications to enhance their adsorption characteristics. For example, the addition of functional groups can improve the selectivity of the separation process. Silica gels are commonly employed in preparative chromatography, especially in purifying biomolecules and natural products. Their effectiveness in environmental analysis is also notable, as they can be used to separate pollutants from water or soil samples.
Polymeric Materials
Polymeric materials represent a more recent development in the realm of molecular sieves. These materials can be engineered to present specific physical and chemical properties, allowing for enhanced separation capabilities. Polymeric sieves offer advantages such as flexibility, ease of processing, and the ability to fine-tune pore structure during synthesis.
Applications for polymeric materials span various fields, including biomedical research, where they can be used for drug delivery systems or enzyme separation. Their relatively lower cost compared to inorganic materials can also make them an appealing option for large-scale applications. Despite these benefits, some limitations exist. For instance, polymers tend to have lower thermal stability than zeolites or silica, which restricts their use in high-temperature processes.
Instrumentation and Methodology
Understanding the instrumentations and methodology in molecular sieve chromatography is crucial for accurate and reliable analysis. These aspects serve as the backbone of effective separation processes, greatly influencing the results and overall efficiency of experiments. The right instruments can affect the resolution and speed of separations. Moreover, the methodologies adopted can optimize the performance, leading to better outcomes in research and applications.
Chromatographic Systems
Chromatographic systems are fundamental to molecular sieve chromatography. They comprise several components that work in unison to achieve separation. Typically, a chromatographic system includes a sample injector, a column packed with molecular sieves, a mobile phase delivery system, a detector, and a data processing unit.
- Sample Injector: This component introduces the sample into the system. Its precision is critical, as it should deliver consistent sample volumes to ensure reproducibility.
- Column: The column is where the actual separation occurs. The choice of molecular sieve material, such as zeolites or silica gels, directly impacts the separation process. The column should be appropriately selected based on the sample characteristics and the desired separation outcome.
- Mobile Phase Delivery System: This system controls the flow rate of the mobile phase, which can be either a liquid or gas. Flow rate can significantly influence the bed dynamics in the column and, subsequently, the separation efficiency.
- Detector: Different detectors can be employed based on the nature of the sample. Common types include UV-VIS detectors and mass spectrometers. Each provides specific advantages depending on the detection needs.
- Data Processing Unit: This component processes and analyzes the data obtained from the detector. Advanced software tools can enhance the interpretation of chromatographic results, making it easier to identify peaks and determine retention times.
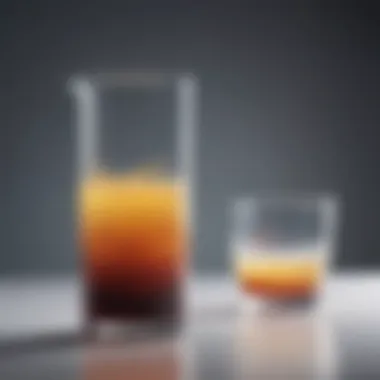
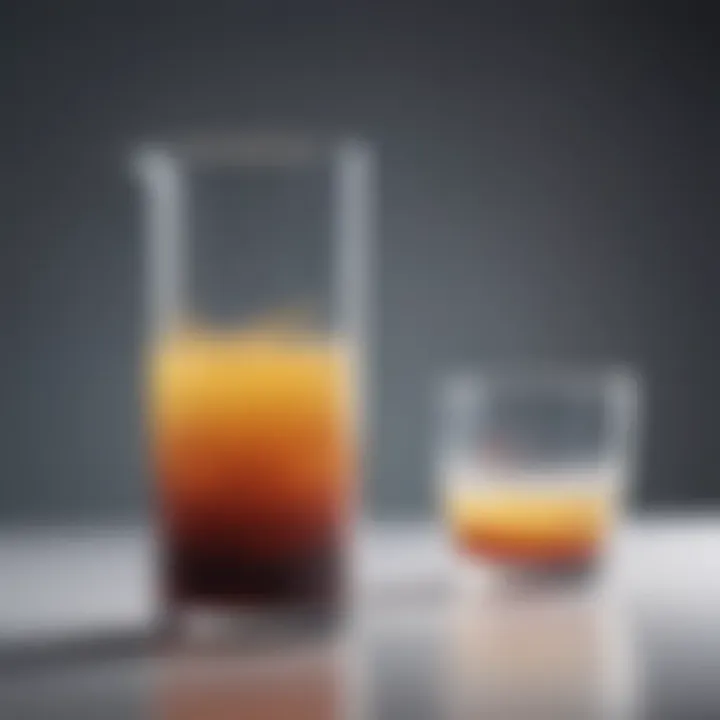
Choosing the right components and having them configured properly is essential for the success of the chromatography experiments.
Operational Protocols
Operational protocols refer to the standard procedures followed during the molecular sieve chromatography processes. They are critical for ensuring the reliability and accuracy of experimental results.
- Sample Preparation: Proper sample preparation is the first step. This includes filtration or dilution processes that can help in obtaining pure samples for accurate analysis.
- Selection of Conditions: Settings such as temperature, flow rate, and pressure must be carefully selected based on the specific types of molecular sieves used. Parameters should be optimized based on preliminary tests, as they can influence resolution and efficiency.
- Calibration: Regular calibration of the system is essential to maintain accuracy. This involves running known standards and checking against expected results. Calibration should be done frequently to ensure high precision.
- Monitoring and Adjustment: During the run, it is important to monitor the system and make adjustments if the results deviate from expectations. Parameters like baseline and peak shapes must be consistently analyzed to ensure reliable outcomes.
- Post-Analysis Procedures: After completing the runs, proper data interpretation and documentation of the results are crucial. This includes retaining raw data for future reference and complying with regulatory standards when applicable.
Adhering to defined operational protocols will foster reproducibility and accuracy in molecular sieve chromatography, thereby advancing analytical chemistry.
"The quality of the data produced from molecular sieve chromatography highly depends on the precision of instrumentation and the rigor of operational protocols."
By grasping both instrumentation and methodology, researchers can leverage molecular sieve chromatography more effectively for varied applications, enhancing their scientific inquiries.
Applications in Analytical Chemistry
Molecular sieve chromatography plays a vital role in analytical chemistry, offering a refined method for separating and purifying complex mixtures. This technique is invaluable in various scientific disciplines due to its ability to isolate specific atoms and molecules based on size. Automating and optimizing this separation process yields significant improvements in both the accuracy and efficiency of chemical analyses.
Several key considerations define the importance of molecular sieve chromatography in analytical settings. Firstly, it facilitates the purification of biomolecules, enabling researchers to extract proteins, nucleic acids, and other vital components from biological samples. Efficient purification is crucial in various applications, particularly in the fields of biotechnology and pharmaceuticals. Secondly, the technique is extensively applied in environmental analysis, allowing for the detection and quantification of pollutants. This capability is essential for environmental protection and compliance monitoring. Finally, in pharmaceutical applications, molecular sieve chromatography is instrumental in drug formulation and quality control, ensuring that active ingredients meet stringent regulatory standards.
"Molecular sieve chromatography stands out for its precision, reliability, and versatility in analytical chemistry settings."
The subsequent sections will delve into each of these applications, elucidating techniques and outcomes that demonstrate the transformative potential of molecular sieve chromatography in scientific research.
Comparative Advantages over Other Techniques
Molecular sieve chromatography has carved a distinct niche in the realm of analytical chemistry. Its effective separation capabilities, especially for complex mixtures, set it apart from other techniques. This section delves into its comparative advantages, focusing on two core attributes: separation efficiency and reproducibility and reliability.
Separation Efficiency
The separation efficiency of molecular sieve chromatography largely hinges on its structural configurations. Unlike traditional chromatography, which may rely significantly on interactions between the analyte and stationary phase, molecular sieve chromatography operates primarily on size exclusion principles. This mechanism allows for the effective separation of analytes based solely on their molecular dimensions.
One major advantage is the minimal retention time variability in molecular sieve systems. Factors like temperature and pressure can be maintained without significant fluctuations, allowing for sharper peak resolution. This attribute is particularly pivotal when analyzing biomolecules, which can vary greatly in size and shape. Given the application of varying sieve sizes, this technique can also be tailored to specifically target desired molecular weights, enhancing its efficiency even further.
- High selectivity: Molecular sieves permit high selectivity based on size, minimizing unrelated component interactions in the separation process.
- Wide applicability: They are versatile in application, catering to small organic compounds as well as larger biological macromolecules.
"Molecular sieve chromatography's reliance on size rather than chemical nature aids in high-resolution separation and purifies compounds effectively."
Reproducibility and Reliability
In scientific research, the reliability of results can never be compromised. Molecular sieve chromatography boasts superior reproducibility compared to other separation techniques, which is crucial in laboratories where consistency in results is paramount. This reproducibility arises from the method's inherent design.
The use of well-characterized and precisely manufactured sieves ensures that experimental runs yield comparable outcomes under similar conditions. Furthermore, the operational protocols are generally straightforward and consistent, minimizing human error—another factor impacting reproducibility. Factors influencing chromatography behavior are more easily controlled, leading to a lower level of variability in results.
- Standardization: Reliable methods and operational protocols have been established, contributing to clearer documentation and repeat experiments.
- Long-term stability: The materials used in molecular sieve construction often exhibit long-term stability. This contrasts with certain materials used in other chromatographic techniques that may degrade over time.
Limitations and Challenges
Understanding the limitations and challenges in molecular sieve chromatography is crucial for both practitioners and researchers. This section highlights specific obstacles that can affect the performance of this technique, impacting its application and effectiveness in various settings. By recognizing these limitations, one can develop more robust methods for overcoming them and ensuring successful outcomes in analytical and preparative scenarios.
Material Cost and Availability
The high cost of materials used in molecular sieve chromatography can be a significant limitation. Zeolites, silica gels, and polymeric materials come with various price points, but some of the high-quality variants can be quite expensive. This raises concerns about the feasibility of large-scale applications in industries where budget constraints are common. Moreover, the availability of these materials can pose challenges, particularly in remote regions or markets with limited access. It requires careful planning and sourcing to ensure that the necessary materials are on hand when needed.
"The cost and availability of molecular sieve materials are vital as they dictate the feasibility of method adoption in both research and industry settings."
Another consideration is the inconsistency in the quality of materials. Some batches may not meet the specified performance criteria due to manufacturing variations or contamination. This inconsistency can lead to unreliable results, which complicates validation efforts in research and industrial processes. Therefore, a thorough evaluation of suppliers and a focus on quality assurance should be prioritized to mitigate these challenges.
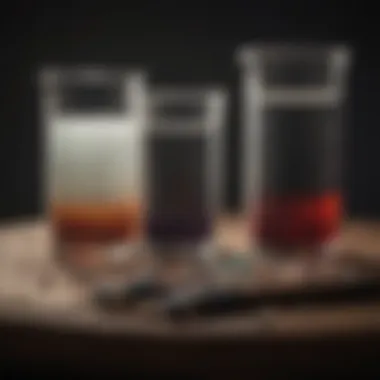
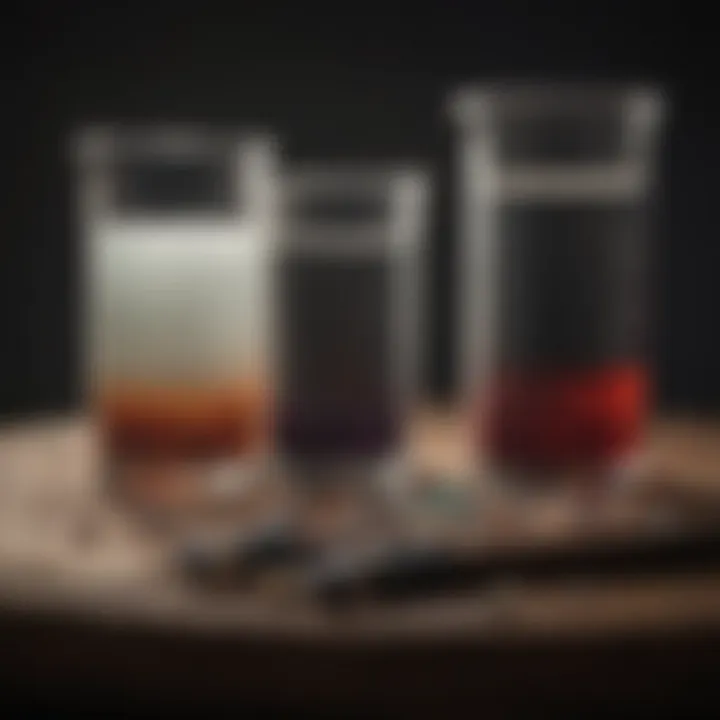
Column Fouling and Maintenance
Column fouling is a crucial challenge encountered in molecular sieve chromatography. This occurs when impurities or other substances accumulate on the column, obstructing the flow of the mobile phase and diminishing separation efficiency. Fouling can arise from a variety of sources, including sample components, poorly prepared mobile phases, or even degradation of the column material over time.
Maintaining column integrity requires a proactive approach. Regular cleaning and regeneration of the columns must be programmed into the operational protocols. However, this maintenance can be labor-intensive and may lead to increased downtime in laboratory settings. Proper selection of materials, along with routine monitoring of column performance, can diminish the impact of fouling. Implementing preventive strategies may save costs in the long run by extending the lifespan of columns and minimizing the need for replacements.
Optimization of Molecular Sieve Chromatography Techniques
Optimizing molecular sieve chromatography techniques is a critical component in achieving effective separation processes in analytical and preparative contexts. As this method is widely utilized in various fields, such as biochemistry and environmental science, understanding how to fine-tune these processes is essential. Optimization affects the resolution, analysis time, and reproducibility of results. Thus, it is prudent to explore specific factors that influence the effectiveness of these techniques.
Parameter Optimization
Parameter optimization involves the careful adjustment of numerous variables that affect the chromatography process. Key parameters include flow rate, temperature, and mobile phase composition. These parameters need to be systematically studied to discern their effect on the efficiency of separation.
- Flow Rate: A higher flow rate may reduce the time of analysis but can compromise resolution. Conversely, a low flow rate can lead to better separation but at the cost of increased analysis time. Finding a balance is paramount.
- Temperature: The operating temperature can significantly influence the interaction between the analyte and the molecular sieve. Elevated temperatures may enhance diffusion but can also affect the integrity of the sample. Hence, temperature profiles should be evaluated based on the specific materials used.
- Mobile Phase Composition: The choice of solvents or additives in the mobile phase is pivotal. The polarity, pH, and ionic strength must be optimized to promote the best separation performance.
By utilizing techniques such as response surface methodology or factorial design, researchers can efficiently study the effect of various parameters on chromatography outcomes. This targeted approach not only saves time but also helps in achieving reproducible results across different experiments.
Method Development Strategies
Developing robust methodologies in molecular sieve chromatography is vital for reliable results. It requires systematic experimentation and a clear understanding of the physicochemical properties of both the analytes and the stationary phases.
- Preliminary Scoping: This involves running preliminary experiments to gather basic information about the behavior of specific analytes under different conditions. This phase often relies on a trial-and-error method to identify potential separation conditions.
- Validation: Once a method is conceived, it needs to be validated. This includes assessing selectivity, sensitivity, and specificity. Validation criteria also encompass repeatability and reproducibility, ensuring that results can be consistently obtained across different lab settings.
- Continuous Improvement: The process of method development should not be static. Continuous data analysis and feedback systems can help to refine methods further. Innovations in materials science, such as newer molecular sieve compositions or improved chromatographic systems, can lead to better performance and efficiency.
Effective optimization and development strategies are essential for maximizing the benefits of molecular sieve chromatography techniques.
The optimization of these techniques is not merely an academic exercise; it has practical implications. For instance, in pharmaceutical applications, achieving a high degree of purity in active compounds can drastically affect product efficacy and safety. In summary, a thorough approach to optimization and method development within molecular sieve chromatography can enhance the reliability and application of this powerful analytical tool.
Future Trends and Innovations
The field of molecular sieve chromatography is constantly evolving. As research continues to advance, several future trends and innovations can reshape its usage in analytical chemistry and related fields. Understanding these developments is crucial as they can lead to enhanced efficiency, greater accuracy, and broader applications. Progress in this area not only signifies technological advancements but also holds the potential to address some existing limitations in molecular sieve chromatography.
Integration with Mass Spectrometry
One significant trend is the increasing integration of molecular sieve chromatography with mass spectrometry. This combination offers powerful capabilities in identifying and quantifying compounds with high specificity. By combining these two techniques, researchers can achieve a more detailed analysis of complex mixtures. The merging of separation and detection provides better resolution and sensitivity, making it invaluable for analyzing biomolecules and environmental samples.
Additionally, mass spectrometry appears as an ideal companion. It can give insights into molecular weights and structural information through fragmentation patterns. More researchers are adopting this dual approach because it streamlines workflows in laboratories, reduces analysis times, and improves data reliability. This trend shows promise for future applications, particularly in pharmaceuticals, where precise compound identification is critical.
Emerging Materials and Technologies
Another exciting area of development is the emergence of new materials and innovative technologies. There are continuous efforts to explore advanced materials with enhanced sieving capabilities. For example, metal-organic frameworks and carbon-based materials have caught the attention of many researchers. These materials could potentially surpass traditional zeolites and silica gels in terms of selectivity and efficiency.
Furthermore, advancements in fabrication techniques allow for the creation of more uniform and tailored sieve structures. This is particularly important for improving the size-selective properties of the chromatography process. With precise engineering, researchers can design molecular sieves to target specific analytes more effectively, leading to higher separation performance.
Technological innovations such as automation and real-time monitoring systems are also gaining traction. These tools can ensure more reproducible results and higher throughput in laboratories. Preparing samples and executing chromatographic techniques can be optimized through automation, reducing human error.
By keeping abreast of these trends, professionals in the field can equip themselves with the necessary skills and knowledge to implement these advancements. It is evident that the future holds significant possibilities for molecular sieve chromatography, paving the way for more innovative applications across various scientific disciplines.
The End
The conclusion serves as a vital synthesis of the entire discussion surrounding molecular sieve chromatography. It is not merely a summary; rather, it encapsulates the significance of the techniques, their applications, and the challenges within this field. The foundation of molecular sieve chromatography lies in its unique capacity to separate molecules based on size, which underscores its importance in both analytical and preparative chemistry. The efficacy of this separation technique contributes significantly to advancements in various scientific disciplines.
Summary of Key Points
In revisiting the essential elements discussed in this article, several key points emerge:
- Foundational Principles: Molecular sieve chromatography operates primarily on the premise of size exclusion. This core principle allows for the efficient separation of different molecules, which has vast implications in fields like biochemistry and environmental science.
- Types of Sieves: Various materials, such as zeolites, silica gels, and polymeric substances, play distinct roles in enhancing separation techniques. Each material offers unique properties that can be exploited based on the specific application.
- Instrumentation: The technological aspects of chromatographic systems are crucial for effective implementation. Detailed methodologies ensure that these systems function optimally.
- Applications: The real-world relevance of molecular sieve chromatography is evident across multiple sectors. From purifying biomolecules to environmental analysis, the applications are numerous and varied.
- Challenges and Innovations: Ongoing research addresses existing limitations, including material cost and column fouling, paving the way for future advancements in the methodology.
Implications for Future Research
The field of molecular sieve chromatography is ripe for exploration and innovation. Future research endeavors promise to yield significant advancements in this discipline, particularly in the following areas:
- Integration with Advanced Technologies: Collaborations between molecular sieve chromatography and other techniques, such as mass spectrometry, can enhance the sensitivity and resolution of analyses.
- Development of New Materials: The quest for more efficient, economical, and durable materials remains a priority. Research into novel polymeric and inorganic materials could revolutionize current methodologies.
- Sustainability in Applications: As environmental concerns continue to rise, developing sustainable practices within the chromatography space will be crucial. Focused research can lead to greener methods and materials.
- Technological Innovations: As computational power increases, the potential for automation and greater precision in chromatographic processes will expand.