Exploring Mass Spectrometry Applications in Science
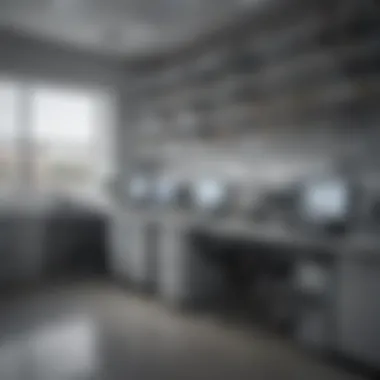
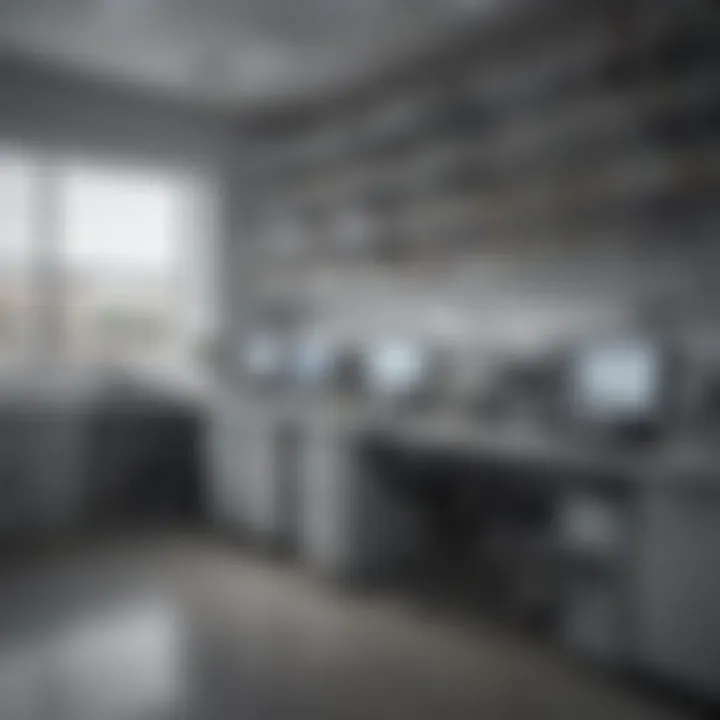
Intro
Mass spectrometry has emerged as a cornerstone technology in modern science, revered for its unparalleled ability to analyze a wide range of compounds with great precision. This technique measures the mass-to-charge ratio of ions, allowing researchers to identify and quantify even the smallest molecules. Its applications are manifold, spanning across disciplines such as chemistry, biology, and environmental science.
As we delve into this article, we will uncover the fundamental principles of mass spectrometry, scrutinize the advancements that have propelled its efficacy, and explore case studies that exemplify its value in research. By understanding both its historical context and current trends, readers will appreciate how mass spectrometry not only enhances analytical capabilities but also paves the way for future discoveries.
Methodology
In discussing the applications of mass spectrometry, it is crucial to first outline the methodology that underpins its use. The methodology section encompasses the design and data collection strategies that typify mass spectrometric analysis.
Study Design
The study designs involving mass spectrometry can vary including exploratory methods for novel material identification to hypothesis-driven investigations aimed at quantifying specific analytes. The approach often aligns with the research objectives, whether they involve targeted metabolomics, proteomics, or environmental sample analysis.
Data Collection Techniques
Data collection in mass spectrometry typically involves several key steps:
- Sample Preparation: This step is critical, as it can greatly influence the quality and accuracy of the results. Techniques such as liquid-liquid extraction or solid-phase microextraction are often employed to isolate target compounds from complex matrices.
- Ionization: Various ionization techniques such as Electrospray Ionization (ESI) and Matrix-Assisted Laser Desorption/Ionization (MALDI) are utilized, depending on the sample type and desired outcome.
- Mass Analysis: Once ions are generated, they are separated based on their mass-to-charge ratios within a mass analyzer, commonly a Time-of-Flight (TOF) or Quadrupole mass spectrometer.
- Data Interpretation: Advanced software tools are used post-analysis to interpret the mass spectra, leading to compound identification and quantification.
Mass spectrometry has revolutionized analytical chemistry, providing insights that were previously unattainable through traditional methods.
Discussion
The discussion portion of the article will encompass interpretations of the findings from various case studies demonstrating the applications of mass spectrometry. It will also address the limitations inherent in the current techniques and propose future research directions to advance the field.
Interpretation of Results
Various case studies shed light on the capabilities and outcomes of mass spectrometry. In proteomics, for instance, mass spectrometry has significantly improved our understanding of protein structures and functions, contributing to advancements in personalized medicine.
In environmental science, it has facilitated the tracking of pollutants and traces of hazardous compounds, enhancing our ability to safeguard ecosystems.
Limitations of the Study
Despite its profound capabilities, mass spectrometry does have limitations. Sensitivity issues, particularly with ionic species that appear in low abundance, can affect detection. Complexity in sample matrices may also introduce interferences that lead to misinterpretations.
Future Research Directions
Future research should focus on refining ionization methods and enhancing software algorithms for data interpretation. Additionally, developing portable mass spectrometers could expand the application of the technology into field analyses, significantly impacting environmental monitoring and on-site diagnostics.
Understanding the applications of mass spectrometry is vital. As it continues to evolve, it holds potential for even greater contributions to science across myriad fields.
Intro to Mass Spectrometry
Mass spectrometry has emerged as a cornerstone technique in many scientific disciplines. Understanding its underlying principles and historical context allows for a deeper appreciation of its diverse applications. In this article, we will detail these aspects comprehensively, situating mass spectrometry firmly within modern scientific exploration.
Historical Development
The roots of mass spectrometry can be traced back to the early 20th century. J.J. Thomson is often credited with the discovery of the electron and the development of the first mass spectrometer around 1913. This device was fundamental in demonstrating the existence of isotopes, which further laid the groundwork for advancements in physical chemistry.
Over the decades, the technology has undergone significant evolution. In the 1940s and 50s, techniques such as the mass spectrometer using gas ionization were developed. The introduction of quadrupole mass filters in the 1970s offered precision and speed, revolutionizing how researchers conducted analyses.
With innovations like time-of-flight and ion trap mass spectrometry coming in the late 20th century, the capabilities of mass spectrometry expanded vastly. Applications began stretching beyond simple molecular analyses to more complex areas, including proteomics and metabolomics. Each leap forward contributed not only to the scientific community's understanding of chemical compounds but also to real-world applications in fields such as drug development and environmental monitoring.
Basic Principles of Mass Spectrometry
At its core, mass spectrometry functions by measuring the mass-to-charge ratio of ions. The basic process can be broken down into three fundamental steps: ionization, mass analysis, and detection.
- Ionization: This is the initial step where a sample is converted into ions. Several methods exist, including electron impact and electrospray ionization. These methods vastly influence the types and states of ions produced, and therefore the data generated later in the analysis.
- Mass Analysis: After ionization, the ions are separated based on their mass-to-charge ratio. Various devices, like quadrupole mass filters or time-of-flight analyzers, are employed in this phase. Each device provides unique advantages, impacting the specificity and sensitivity of the analysis.
- Detection: Finally, the separated ions are detected, and their quantities measured. This information is converted into a mass spectrum, an invaluable tool providing insights into the composition of the original sample.
The integration of these steps allows researchers to understand complex chemical mixtures. Moreover, advances in technology continuously improve the resolution and sensitivity of mass spectrometry, opening new avenues of research.
"Mass spectrometry has become the microscope of the molecular world, revealing details of complex mixtures with unprecedented precision."
In summary, the historical and basic principles of mass spectrometry provide a solid foundation for appreciating its applications in modern scientific inquiries. Its importance cannot be overstated, as it continues to drive discoveries across multiple scientific domains.
Fundamentals of Mass Spectrometry
Mass spectrometry is a fundamental analytical tool in modern science. Understanding the basic principles and components of mass spectrometry provides the foundation for its diverse applications. The fundamentals consist of ionization techniques and mass analyzers. These elements are crucial as they determine how samples are prepared, how the ions are formed, and how their masses are measured. Each technique presents specific advantages and constraints which influence their suitability for various applications.
Ionization Techniques
Ionization is the first major step in mass spectrometry. It transforms neutral molecules into ions, enabling their detection and analysis. Different ionization techniques can affect the mass spectrometric results notably. Each method has unique characteristics, making them suitable for different types of samples.
Electron Impact Ionization
Electron impact ionization is one of the oldest and most widely used techniques. It involves bombarding a sample with high-energy electrons to generate positive ions.
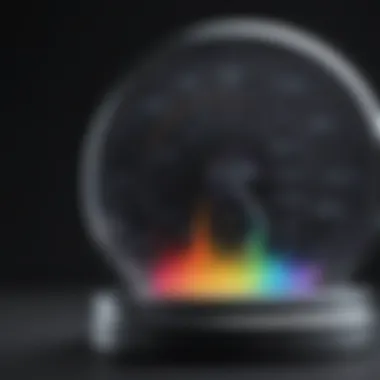
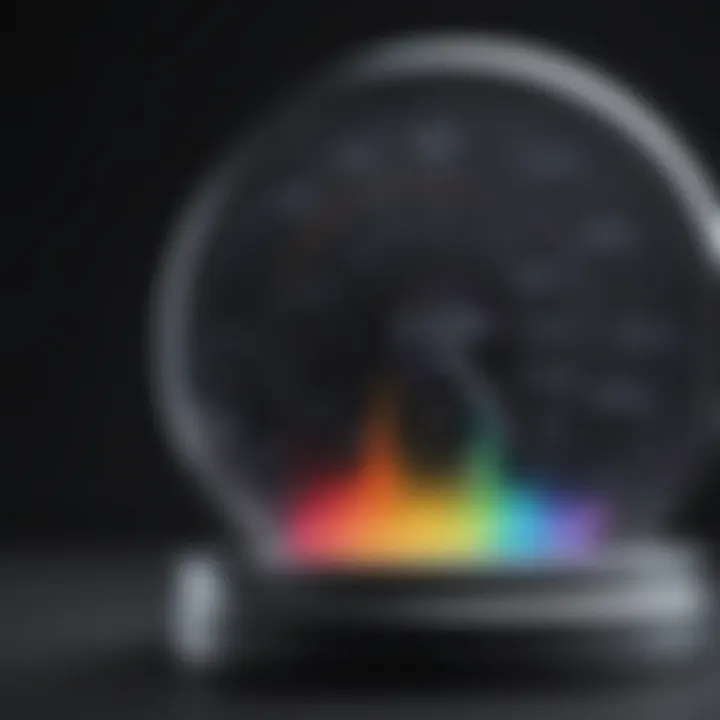
- Key characteristic: It provides high sensitivity and is effective for small, volatile molecules.
- Benefit: Electron impact ionization is a popular choice for analyzing gas-phase samples in organic chemistry.
- Unique feature: It often leads to fragmentation, which can provide detailed structural information about the analyte, though this can complicate data interpretation.
The main disadvantage is its limited applicability to larger biomolecules, which may not survive the ionization process without undergoing significant fragmentation.
Electrospray Ionization
Electrospray ionization (ESI) allows for the analysis of larger biomolecules such as proteins and peptides. It works by creating an aerosol of charged droplets from a liquid sample, which evaporates to form charged ions.
- Key characteristic: ESI is effective for high-mass molecules and can produce a multitude of charge states.
- Benefit: It enables the analysis of complex mixtures without prior separation, particularly beneficial in proteomics and metabolomics.
- Unique feature: The technique is soft, meaning it does not fragment the molecules significantly, preserving their integrity for better analysis.
However, a disadvantage of ESI is susceptibility to variations in solvent composition which can affect reproducibility.
Matrix-Assisted Laser Desorption/Ionization
Matrix-assisted laser desorption/ionization (MALDI) is a powerful technique particularly favored in the field of proteomics. It uses a laser to excite a matrix material, which then ejects ions from the analyte matrix.
- Key characteristic: MALDI is suitable for analyzing large biomolecules and is coupled often with time-of-flight (TOF) mass analyzers.
- Benefit: It allows for rapid analysis with minimal sample preparation and high sensitivity.
- Unique feature: The technique can create a mass spectrum with high resolution and accuracy, enabling identification of complex mixtures.
The downside of MALDI can be the need for a suitable matrix, which may not be compatible with all types of analytes, affecting its versatility.
Mass Analyzers
The mass analyzer is the second crucial step in mass spectrometry. It separates the ions based on their mass-to-charge ratios (m/z). This is integral to the identification and quantitation of the analyte species. Different types of mass analyzers have distinct advantages and characteristics, influencing their use in specific applications.
Quadrupole Mass Filter
The quadrupole mass filter is a commonly used mass analyzer. It consists of four parallel rods that create oscillating electric fields to filter ions based on their m/z ratio.
- Key characteristic: It provides fast data acquisition and is suitable for quantitative analysis.
- Benefit: The quadrupole is popular in routine analyses due to its robustness and ease of operation.
- Unique feature: It allows for selective ion monitoring, which is ideal for targeted analysis.
However, it may have limitations in resolution compared to other advanced analyzers.
Time-of-Flight Mass Analyzer
Time-of-flight (TOF) mass analyzers measure the time it takes for ions to travel a known distance to determine their mass.
- Key characteristic: TOF provides high mass accuracy and resolution.
- Benefit: It is particularly effective for analyzing large biomolecules like proteins.
- Unique feature: It can accommodate a wide range of mass values in a single analysis, supporting high-throughput applications.
One disadvantage is that it can be sensitive to the initial ionization process which might lead to varied results based on the sample composition.
Orbitrap
The Orbitrap mass analyzer combines features of both quadrupole and TOF mass analyzers. It captures ions in a cylindrical electrode arrangement, measuring their oscillation frequencies to determine mass.
- Key characteristic: It offers exceptional mass resolution and accuracy.
- Benefit: The Orbitrap can analyze complex mixtures with high precision, useful in proteomics and metabolomics.
- Unique feature: It can measure a large number of ions simultaneously, enhancing data acquisition rates.
However, it can require more sophisticated equipment and calibration, which may limit accessibility for routine analyses.
Mass spectrometry combines these ionization techniques and mass analyzers to enable diverse applications in modern science, proving critical in advancing analytical capabilities.
Applications in Chemistry
The role of mass spectrometry in chemistry is pivotal. This technique facilitates the in-depth study of chemical compounds, their structure, and behaviors. With its ability to determine the mass-to-charge ratio of ions, mass spectrometry provides crucial insights that traditional methods may not uncover. The precision and sensitivity of mass spectrometry make it indispensable in diverse chemistry applications.
Analytical Chemistry
Analytical chemistry relies heavily on mass spectrometry to identify and quantify substances in complex mixtures. This method allows chemists to analyze samples with remarkable specificity, essential for both academic research and industrial applications. One of the strongest benefits is its ability to detect trace amounts of compounds, which is crucial in fields like pharmaceuticals and environmental analysis. Techniques such as electron impact ionization and electrospray ionization enhance sensitivity, enabling the detection of even low-abundance analytes.
The integration of mass spectrometry with chromatography techniques, such as gas chromatography and liquid chromatography, further increases its analytical power. This approach not only streamlines sample preparation but also improves the resolution of compound separation. Following these techniques allows chemists to produce highly accurate datasets that aid in research and product development.
Drug Development and Metabolism Studies
In the realm of drug development, mass spectrometry provides invaluable support. It assists in the characterization of drug compounds and metabolite profiling during the development stages of new medications. By using mass spectrometry, scientists can monitor the absorption, distribution, metabolism, and excretion (ADME) of drugs within biological systems. This holistic view helps to understand the drug's efficacy and safety profile.
Quantitative analysis is essential for evaluating drug responses and toxicity. Mass spectrometry can isolate specific metabolites, offering a detailed understanding of how the body interacts with a drug. It enables researchers to identify potential biomarkers associated with drug metabolism, influencing decision-making throughout the drug development process.
Environmental Analysis
Environmental analysis is another critical application of mass spectrometry. Researchers utilize this technique to detect pollutants and assess environmental hazards effectively. The ability to identify trace levels of contaminants in air, water, and soil makes mass spectrometry an essential tool in environmental chemistry.
For instance, monitoring pesticide residues in agricultural products is vital for food safety. Mass spectrometry enables the detection and quantification of these hazardous substances with high accuracy. The data gathered allows regulatory bodies and environmental agencies to enforce safety standards and make informed decisions on health risks.
Moreover, the adaptability of mass spectrometry means it can be applied in various sectors such as wastewater management, where it aids in tracking contaminants and evaluating treatment processes. Understanding how pollutants behave and degrade helps in developing better environmental policies and practices.
Mass spectrometry has transformed how chemists approach analytical challenges in various sectors, providing unmatched precision and insight.
Mass Spectrometry in Biology
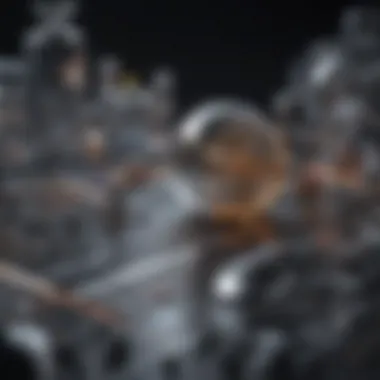
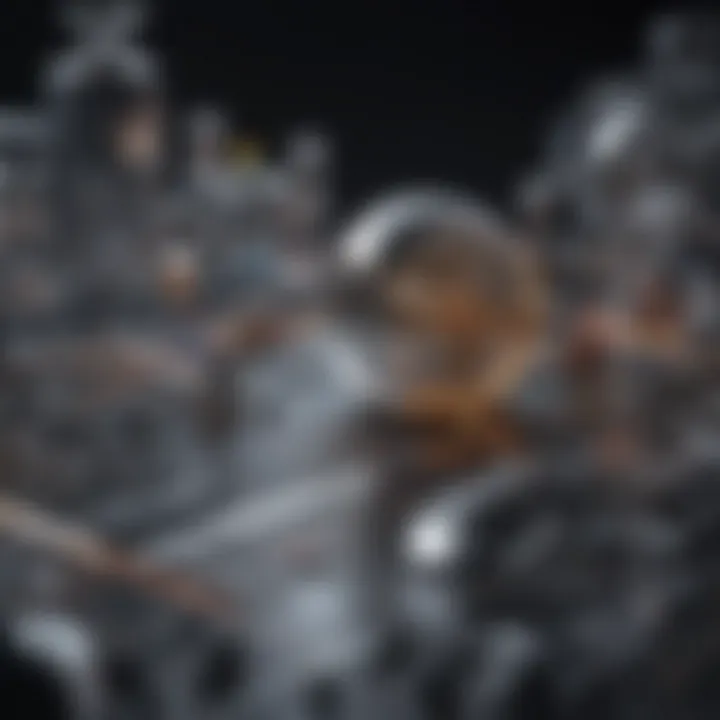
Mass spectrometry (MS) has emerged as a crucial tool in the biological sciences. Its ability to analyze complex biomolecules with high sensitivity and specificity places it at the forefront of various research areas. This section explores how mass spectrometry contributes to the understanding of biological systems and phenomena.
Proteomics
Proteomics, the large-scale study of proteins, capitalizes on mass spectrometry for protein identification and quantification. It enables researchers to investigate the structure and function of proteins, which are vital for cellular processes. By utilizing techniques such as liquid chromatography coupled with mass spectrometry (LC-MS), scientists can separate and analyze proteins from complex mixtures effectively.
Key aspects of proteomics include:
- Protein profiling: MS allows for the comprehensive profiling of proteins within a sample. This is essential for understanding cellular responses to various stimuli.
- Post-translational modifications: Mass spectrometry can detect and characterize modifications such as phosphorylation or glycosylation, which are critical for protein function.
- Biomarker discovery: In clinical settings, proteomics facilitates the identification of biomarkers for diseases, helping in early diagnosis and treatment planning.
In summary, proteomics enriches our knowledge of biological mechanisms and aids in identifying potential therapeutic targets.
Metabolomics
Metabolomics involves the study of metabolites, the small molecules produced during metabolic processes. Mass spectrometry plays a pivotal role in characterizing and quantifying metabolites. This discipline is particularly useful for understanding metabolic pathways and their alterations in diseases.
Considerations in metabolomics include:
- Comprehensive profiling: MS provides extensive data on the composition of metabolites in biological samples, offering insights into metabolic states.
- Disease association studies: By comparing metabolomic profiles of healthy and diseased individuals, MS helps identify potential biomarkers and therapeutic targets.
- Dynamic processes: Metabolomics studies are crucial for understanding real-time metabolic changes in response to physiological conditions or treatments, allowing for a deeper understanding of disease mechanisms.
The integration of metabolomics with MS presents a powerful approach for the study of biology at a molecular level.
Genomic Applications
Mass spectrometry has begun to play a role in genomic applications. While sequencing techniques primarily determine DNA sequences, mass spectrometry aids in studying nucleic acids' modifications and interactions. Its ability to analyze large biomolecules provides insights into genomic organization and epigenetics.
Highlights of genomics applications include:
- DNA and RNA analysis: MS can characterize nucleotides and their modifications, contributing to understanding gene expression and regulation.
- Epigenetic changes: Mass spectrometry allows for the investigation of chemical modifications on DNA and histones, crucial for gene regulation.
- Integration with other techniques: Combining MS with next-generation sequencing offers comprehensive insights into the genomic and epigenomic landscapes.
Innovative Advancements in Mass Spectrometry
The field of mass spectrometry continues to evolve, driven by technological advancements and the increasing demand for precision in scientific research. Innovative advancements in mass spectrometry not only enhance the capabilities of existing techniques but also open new avenues for exploration in various applications. These advancements include high-resolution mass spectrometry, integration with chromatography techniques, and the development of miniaturized and portable systems. Understanding these advancements is crucial for researchers and professionals aiming to leverage mass spectrometry in their work.
High-Resolution Mass Spectrometry
High-resolution mass spectrometry represents a significant leap in analytical capabilities. Traditional mass spectrometry often struggled with the overlapping signals of ions. However, with high-resolution systems, it becomes possible to resolve ions with very similar mass-to-charge ratios. This leads to improved specificity and sensitivity in detecting compounds, which is vital, particularly in complex mixtures.
The benefits of high-resolution mass spectrometry include:
- Enhanced Detection: Ability to identify trace components in samples.
- Unambiguous Identification: Reduced chances of false-positive results by distinguishing isobaric ions.
- In-depth Analysis: Facilitates complex analysis such as metabolomics and proteomics.
High-resolution techniques have been adopted in clinical diagnostics, where precise identification can impact patient treatment outcomes. With these advancements, researchers can now analyze biomolecules at unprecedented levels, significantly improving the understanding of metabolic pathways and disease mechanisms.
Integration with Chromatography Techniques
The integration of mass spectrometry with chromatography techniques is a transformative advancement in analytical chemistry. Coupling methods like gas chromatography (GC) and liquid chromatography (LC) with mass spectrometry enhances the separation and identification of compounds in a mixture. The synergy between these techniques allows for comprehensive analysis even in challenging samples.
Key advantages of this integration include:
- Improved Resolution: Better separation of components before mass analysis.
- Broader Application Scope: Suitable for both volatile and non-volatile substances.
- Efficiency: Reduces analysis time while maintaining reliability and accuracy.
For instance, in pharmaceutical research, this integration is vital for identifying drug metabolites and ensuring purity in compound development. The enhanced analytical capability provided by chromatography-mass spectrometry combinations leads to faster and more reliable results, which is critical in regulatory environments.
Miniaturization and Portable Systems
The miniaturization of mass spectrometry equipment has made significant strides in recent years. Portable mass spectrometers are now capable of performing analyses outside of traditional laboratory settings. This advancement offers the flexibility to conduct experiments in the field, providing immediate results.
Benefits of miniaturization include:
- Accessibility: Enables testing in remote locations, such as disaster sites or rural areas.
- Real-time Analysis: Facilitates on-the-spot results, which can be crucial for decision-making.
- Cost Efficiency: Smaller systems often require less expensive maintenance and operation.
Miniaturized devices have found applications in food safety, environmental monitoring, and even in clinical diagnostics. The ability to analyze samples onsite significantly reduces the time from sample collection to result reporting, which can enhance response times in emergencies or critical situations.
"The advancements in mass spectrometry are not just about improving accuracy but also about making technology accessible and practical for real-world applications."
In summary, the innovative advancements in mass spectrometry bring transformative changes in research and application. From high-resolution techniques that refine analytical processes to portable systems that expand the reach of mass spectrometry, these innovations promise to enhance the impact of this powerful analytical tool in various scientific domains.
Case Studies of Mass Spectrometry Applications
In the field of mass spectrometry, real-world applications emphasize the significance of this analytical tool. Case studies allow researchers to grasp the scope of its utility in solving complex problems across various domains. By exploring specific instances of mass spectrometry applications, we can see its versatility and effectiveness in diverse scientific challenges. These case studies not only validate the principles of mass spectrometry but also showcase its role in advancing fields such as clinical diagnostics, food safety, and forensic analysis.
Case Study in Clinical Diagnostics
Mass spectrometry has revolutionized clinical diagnostics in numerous ways. It offers rapid and accurate detection of biomarkers critical for disease detection and monitoring. For instance, a notable application is in the detection of metabolites related to diseases such as diabetes and cancer. Researchers utilize techniques like Electrospray Ionization to analyze small sample volumes, making it feasible for routine usage in clinical laboratories.
One case study illustrates how mass spectrometry is applied to detect specific protein markers in blood samples. In this study, the analysis identified early-stage cancer biomarkers, enabling a more timely diagnosis. This application highlights the shift towards precision medicine, where diagnostics can be tailored to individual patient profiles, improving treatment outcomes significantly.
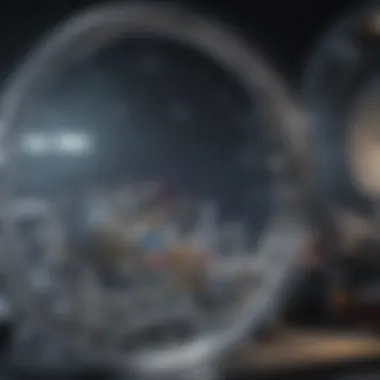
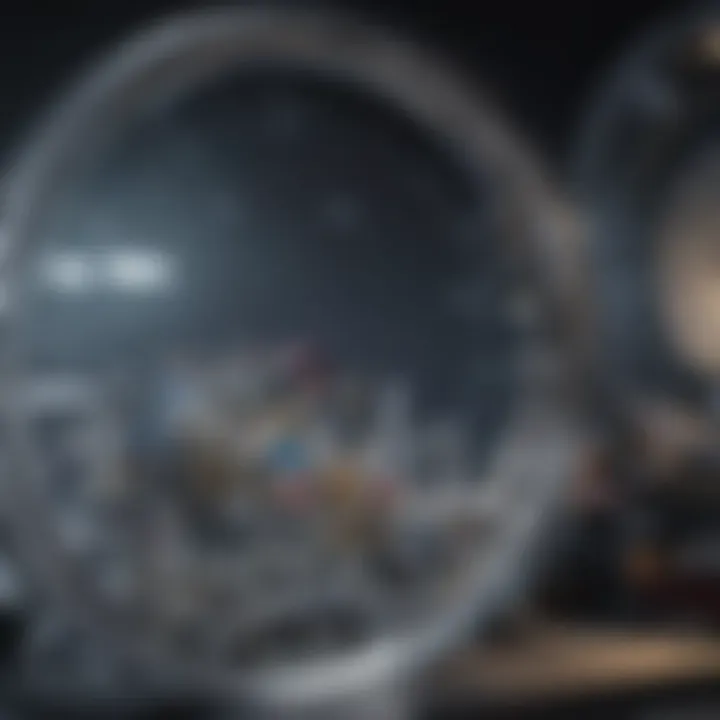
Case Study in Food Safety Testing
In food safety, mass spectrometry plays an essential role in ensuring the integrity of food products. The detection of contaminants, including pesticides and heavy metals, is critical for consumer safety. In one case study, scientists employed Liquid Chromatography-Mass Spectrometry to examine fruit samples for pesticide residues.
The results showed the presence of several banned substances, prompting regulatory action and raising public awareness about food safety. This demonstrates how mass spectrometry not only aids in quality assurance but can also influence agricultural practices. The ability to monitor food safety continuously underscores mass spectrometry's importance in the food industry, ensuring compliance with health regulations and protecting public health.
Case Study in Forensic Analysis
Forensic science benefits immensely from mass spectrometry, particularly in drug testing and toxicology. In a relevant case study, forensic experts utilized Gas Chromatography-Mass Spectrometry to analyze unknown substances found at a crime scene. The accuracy of mass spectrometry allowed investigators to identify compounds that were previously unknown, providing critical evidence in criminal investigations.
Moreover, this application supports law enforcement in understanding drug interactions and potential health hazards associated with illicit substances. Mass spectrometry's ability to provide conclusive and reproducible data enhances the reliability of forensic evidence presented in court. Its emergence as a cornerstone in forensic analysis emphasizes the ongoing evolution of mass spectrometry in solving real-world challenges.
The integration of mass spectrometry in various fields showcases its transformative impact, illustrating not only its scientific capabilities but also its importance in societal issues.
Through these case studies, the adaptability and precision of mass spectrometry become evident. This analytical technique stands out as a leader in innovation, confirming its essential role in modern science and research.
Challenges in Mass Spectrometry Applications
The field of mass spectrometry has made significant contributions across various scientific disciplines. However, the application of this powerful analytical technique is not without its challenges. Understanding these challenges is crucial for researchers and practitioners working in the field. Addressing technical limitations and complexities in data interpretation can enhance not only the accuracy of results but also the overall effectiveness of mass spectrometry in research and application.
Technical Limitations
Despite its advanced capabilities, mass spectrometry faces several technical limitations. The resolution and sensitivity of the mass spectrometer can be influenced by factors such as the type of ionization method used and the design of the mass analyzer. For instance, high-resolution mass spectrometry is powerful but can be complicated by the presence of isobaric interferences in samples. These interferences can obscure results, making it challenging to distinguish between different analytes that have similar mass-to-charge ratios.
Some mass spectrometers also exhibit limitations concerning sample complexity. In biological matrices, for example, the presence of myriad compounds can lead to ion suppression, affecting the quantification of target molecules. This is a significant consideration, especially in proteomics where complex mixtures of proteins are analyzed. Furthermore, the requirement for sample preparation can introduce variability into the results. This variability can arise from the extraction methods used or the loss of analytes during processing.
The technical limitations must continuously be addressed through innovation in mass spectrometry equipment and methodology.
Data Interpretation Complexities
Data interpretation is another critical challenge faced when using mass spectrometry. The data generated from this method is complex and multidimensional. Researchers often encounter difficulties in deconvoluting this data into meaningful information. Factors such as overlapping peaks, noise, and the presence of contaminants in the sample can lead to misinterpretation.
To accurately interpret results, sophisticated bioinformatics tools are necessary. These tools need to align with the specific kind of data generated, whether from proteomics, metabolomics, or other applications. However, the diversity of these tools can create a steep learning curve for practitioners. As scientific questions evolve, a need for new algorithms and data analysis strategies also emerges.
Additionally, mass spectrometry often generates large datasets, which require significant computational resources to analyze. This demand for computing power can be a barrier, particularly in smaller laboratories or institutions with limited access to advanced computing facilities.
Future Directions in Mass Spectrometry
The field of mass spectrometry is ongoing and evolving with new methodologies and technologies. The importance of understanding future trends in mass spectrometry cannot be overstated. This section focuses on emerging technologies and potential new applications, stressing how these developments can reshape research across various scientific domains.
Emerging Technologies
Advancements in mass spectrometry technologies promise to enhance the capability and reach of this analytical tool. Key developments include:
- Nanoelectrospray Techniques: These enable analysis of very small samples, beneficial in proteomics and metabolomics.
- Improved Ionization Methods: Innovations such as Atmospheric Pressure Chemical Ionization facilitate measurements in ambient conditions.
- Hybrid Mass Analyzers: These instruments combine different mass spectrometric techniques, increasing the precision and specificity of analyses.
Emerging technologies not only improve performance but also lower costs. This democratizes access to mass spectrometry in laboratories around the world, fostering greater innovation in research.
Potential New Applications
The future of mass spectrometry lies in its ability to expand into new areas. Some potential applications to consider include:
- Personalized Medicine: Mass spectrometry can assist in the customization of treatment plans based on individual metabolic profiles.
- Biomarker Discovery: The identification of unique biomarkers can lead to earlier diagnostics and better understanding of diseases.
- Food Safety Testing: Rapid screening of contaminants can improve safety measures in the food supply.
Developing these applications requires collaboration among scientists, engineers, and healthcare professionals. Addressing the complexities and limitations of current technologies is crucial for the successful integration of mass spectrometry into these new areas.
"As mass spectrometry continues to advance, its integration into various disciplines will become increasingly relevant, paving the way for innovative breakthroughs in science."
The future directions outlined in this section reflect the potential for mass spectrometry to remain at the forefront of scientific discovery.
Finale
The conclusion is a fundamental part of this article because it sums up everything that has been discussed. It serves to remind readers of the significance of mass spectrometry in various scientific fields. By synthesizing key insights raised throughout the paper, it reinforces the value of employing mass spectrometry in both research and practical applications. This section emphasizes the breakthroughs that have risen from mass spectrometry, regarding analytical efficiency and sensitivity.
In addition, the conclusion also highlights the limitations and challenges the field faces. Acknowledging these hurdles is crucial for driving future advancements. This call for continued research and innovation can mobilize scientific communities towards overcoming these obstacles. Moreover, it serves as an invitation to engage with the ongoing developments in the field, as technologies evolve rapidly.
Ultimately, the importance of this conclusion lies in its ability to connect past information with future possibilities. It wraps up the discussion neatly while stimulating thoughts on how mass spectrometry can grow and adapt, ensuring its relevance to new research challenges.
Summary of Key Insights
In summarizing the key insights of the article, we can highlight several pivotal points:
- Diverse Applications: Mass spectrometry is versatile, used in areas like chemistry, biology, and environmental science.
- Technological Advancements: Innovative developments such as high-resolution mass spectrometry and portable systems enhance its utility.
- Case Studies: Real-world applications in clinical diagnostics, food safety testing, and forensic analysis underscore its practical significance.
- Research Challenges: The field is not without limitations, particularly in data interpretation and technical barriers.
These insights collectively underlie the importance of mass spectrometry as an essential tool in modern science, showcasing its capacity to uncover new knowledge across numerous domains.
Implications for Future Research
The implications for future research surrounding mass spectrometry are both broad and critical. As emerging technologies continue to permeate the field, there are several areas worthy of exploration:
- Integration of New Techniques: Future research can focus on combining mass spectrometry with genetic sequencing technologies or advanced imaging methods to enrich analysis.
- Environmental Monitoring: Mass spectrometry holds the potential to deepen environmental research, examining pollutants at minute levels.
- Personalized Medicine: The evolution of mass spectrometry could lead to breakthroughs in individualized treatment plans by analyzing biomolecules specific to patients.
- Machine Learning and Data Analysis: Incorporating machine learning may vastly improve the interpretation of mass spectrometry data, making it more reliable and efficient.
In summary, the future of mass spectrometry is promising. Continuous exploration and adaptation to new challenges and advancements will ensure its crucial role in scientific research.