Latest Physics Discoveries: Insights and Implications
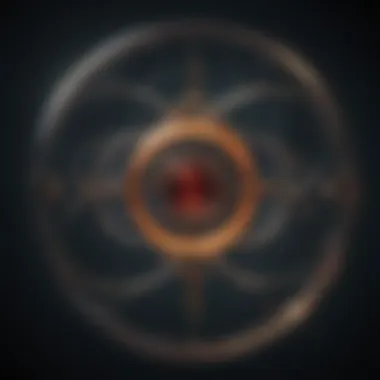
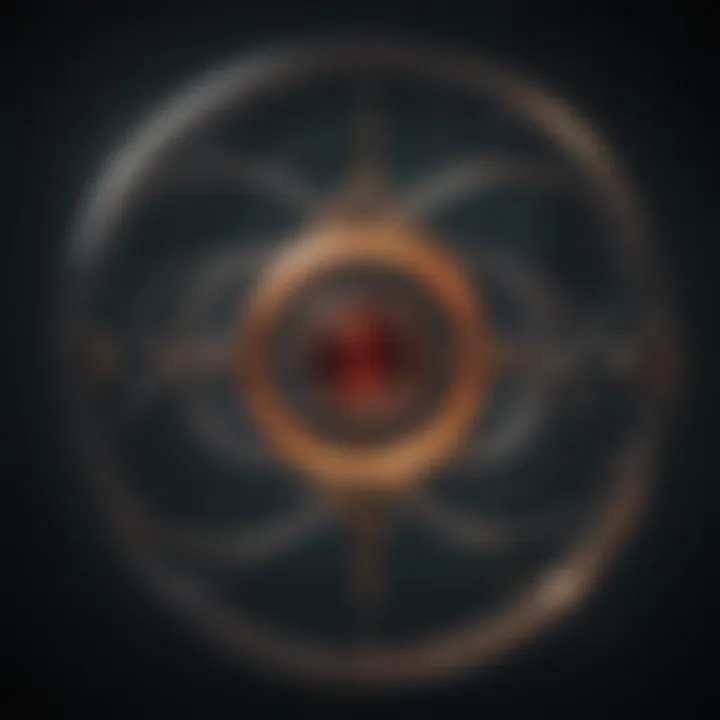
Intro
Physics is an ever-evolving field where new discoveries continuously reshape our understanding of the universe. In recent years, numerous advancements have emerged, providing fresh insights into the workings of fundamental forces and particles. The importance of these discoveries is not limited to theoretical physics; they extend to practical applications and future technologies. This article delves into the highlights of these groundbreaking findings, ensuring clarity for both specialists and those unfamiliar with advanced concepts.
Methodology
Study Design
This overview is structured to analyze significant recent physics discoveries through a systematic approach. The examination includes both theoretical frameworks and experimental studies. It combines literature review with case studies from various research papers to capture a comprehensive picture of recent advancements. The objective is to synthesize information in a way that reveals the interconnections between different discoveries and their implications.
Data Collection Techniques
Information gathering encompasses several techniques. First, peer-reviewed journals are consulted, ensuring the latest research is accurately represented. Websites like Wikipedia and Britannica are useful for context and auxiliary information. Additionally, expert discussions and forums, such as those found on Reddit, contribute valuable insights from the physics community.
Discussion
Interpretation of Results
Recent breakthroughs have challenged previously held notions in physics. For instance, the discovery of gravitational waves has confirmed predictions made by Einstein’s general relativity. Scientists continue investigating the implications of these waves in our understanding of cosmic events. Moreover, advances in quantum entanglement are paving new pathways in quantum computing, indicating profound changes in technology.
Limitations of the Study
While this overview strives for a comprehensive view, the limitations are notable. The rapid pace of discovery means some findings may be omitted. Additionally, the complexity of certain concepts can make it challenging to present information in an accessible manner for non-experts. It is essential to approach each discovery critically, recognizing that ongoing research may alter interpretations.
Future Research Directions
Future research in physics appears promising. As scientists delve deeper into the implications of dark matter and dark energy, many questions remain unanswered. Initiatives targeting fusion energy could revolutionize energy production. Furthermore, as we advance in our technological capabilities, the potential exists for new disciplines within physics to emerge, widening the field and our understanding of the universe.
"Theoretical and experimental breakthroughs in physics continue to challenge and refine our understanding of the cosmos. Each discovery acts as a stepping stone to further inquiries."
Prolusion to Recent Advances in Physics
The landscape of physics is continually evolving, driven by research, technology, and new methodologies. Recent advances in physics are not merely academic; they have tangible implications for various aspects of daily life, technology, and our broader comprehension of the universe. As we delve into these discoveries, it becomes evident that they significantly influence myriad fields, from engineering to cosmology and from quantum theory to material science.
Important elements of modern physics arise from a fusion of theoretical foundations and empirical observations. The integration of distinct areas of study—from gravitational waves to dark matter research—highlights a commitment to unraveling some of the most pressing questions about existence.
Defining the Scope of Modern Physics
Modern physics is an intricate domain where multiple principles intersect, offering profound insights into both the micro and macro cosmos. Key elements comprise concepts such as relativity, quantum mechanics, thermodynamics, and electromagnetism. Collectively, they provide a framework for explaining natural laws that govern physical phenomena.
- Relativity Theory: Developed by Albert Einstein, this theory describes how the laws of physics are the same for all non-accelerating observers. It ushered in a new understanding of space and time.
- Quantum Mechanics: This branch explores matter and energy on atomic and subatomic levels, introducing concepts like wave-particle duality and uncertainty.
Recent advancements, particularly in areas such as quantum entanglement and gravitational wave detection, represent leaps forward in application and understanding. These investigations offer essential perspectives not only for theoretical physicists but also carry implications for technological innovation and future research. Understanding these foundations allows us to appreciate the complex interdependencies that shape our modern worldview.
The Concept of Quantum Entanglement
Quantum entanglement is a fundamental phenomenon in quantum mechanics that has significant implications for our understanding of the physical world. It describes a situation where two or more particles become interconnected in such a way that the state of one particle directly affects the state of another, regardless of the distance separating them. This concept challenges classical intuitions about locality and separability, prompting deeper inquiries into the nature of reality.
The Fundamentals of Entanglement
To grasp quantum entanglement, one must first understand the principles that govern it. When particles become entangled, their quantum states are no longer independent. Instead, they form a single quantum state. For example, consider two photons that are generated in an entangled state. If one photon is measured and its polarization determined, the polarization of the second photon can be instantly inferred, even if it is light-years away.
This behavior is not limited to photons. It applies to any quantum systems, including atoms and electrons. The phenomenon was famously described by Albert Einstein, who referred to it as "spooky action at a distance." However, experiments have since validated quantum entanglement, underscoring its validity as a crucial feature of quantum theory. The implications for information transfer and quantum communication are profound, with potential applications in quantum computing and cryptography.
Recent Experimental Confirmations
Recent advancements in technology have allowed scientists to conduct experiments that confirm the principles of quantum entanglement under increasingly sophisticated conditions. One notable experiment conducted by the team at the University of Science and Technology of China in 2017 demonstrated entanglement over a distance of 500 kilometers using a satellite. This achievement marked a milestone, illustrating that entangled particles can maintain their connections over vast distances without losing coherence.
Moreover, another significant experiment involved the use of a Bell test. This type of experimental setup is designed to demonstrate that entangled particles display correlations that violate classical expectations. The results of these tests avoid local hidden variable theories, suggesting that the behavior of entangled particles cannot be explained without invoking fundamental quantum mechanics.
"Entanglement is not just a peculiarity of quantum mechanics; it’s a powerful resource for the next generation of technologies."
Advancements in Gravitational Wave Detection
Gravitational waves are ripples in spacetime caused by the acceleration of massive objects, like colliding black holes or neutron stars. The detection of these waves marks a significant advancement in modern physics. It opened a new avenue for observing cosmic events that were previously invisible to traditional electromagnetic observations. This area of research not only deepens our understanding of the universe but also tests the predictions of general relativity. The growth of this field highlights the intersection of theory and experimental physics, making it a crucial area for future research.
The Role of LIGO and Virgo
The Laser Interferometer Gravitational-Wave Observatory (LIGO) and Virgo represent pioneering projects in the detection of gravitational waves. Launched in 2015, LIGO made headlines with its first detection of gravitational waves, confirming the existence of collisions between black holes. This event was a monumental achievement in physics, demonstrating experimental capabilities that allow us to explore the fundamental phenomena of the universe.
LIGO consists of two large detectors located in Washington and Louisiana, which use laser beams to measure the miniscule changes in distance caused by gravitational waves. Virgo, located in Italy, complements LIGO's observations, enhancing the overall sensitivity and allowing for a more precise localization of gravitational wave sources. The collaboration between these facilities exemplifies the global effort in advancing gravitational wave astronomy.
The success of these observatories has ushered in a new era of multi-messenger astronomy, where data from gravitational waves combine with electromagnetic observations, thus enriching the understanding of cosmic events. As these detection methods improve, researchers are able to observe more stellar phenomena, expanding our knowledge of the universe.
Significant Observations and Their Interpretations
The observations made by LIGO and Virgo have provided remarkable insights into the nature of black holes and neutron stars. Each detected event offers a unique perspective, prompting a reevaluation of what we understand about the dynamics and formations of these exotic objects. For example, the detection of the merger of two black holes showed that they were more massive than expected, leading to discussions on how these bodies form and evolve over time.
Another significant finding was a gravitational wave signal from a neutron star collision. This event not only confirmed theories about heavy element formation through such mergers but also allowed for the first observation of electromagnetic radiation emitted during the event. This signals a shift in astrophysics, where gravitational wave detection acts as a new window into cosmic phenomena.
These interpretations extend beyond mere curiosity. Understanding the mechanisms behind these cosmic events has implications for the large-scale structure of the universe and the fundamental laws of physics. As more events are detected and analyzed, a richer narrative of the cosmos continues to unfold.
"Gravitational waves have opened up a new paradigm in astrophysics. They allow us to see the universe in a completely new way." – physicist involved in LIGO research.
Breakthroughs in Dark Matter Research
The exploration of dark matter represents one of the most complex and intriguing areas in modern physics. Its significance lies not only in its mysterious nature but also in its pivotal role in shaping our understanding of the cosmos. Approximately 27% of the universe is believed to consist of dark matter, yet it does not emit, absorb, or reflect light, making it invisible and difficult to study. This section delves into both the theoretical frameworks that have emerged and the recent experimental findings that contribute to this field.
Theoretical Frameworks and Models
Various theoretical frameworks have been proposed to explain the existence and behavior of dark matter. Weakly Interacting Massive Particles (WIMPs) are among the most favored candidates in current particle physics. These hypothetical particles interact through the weak nuclear force, which makes them difficult to detect. Researchers often employ models like supersymmetry, which suggests that each particle has a heavier partner, potentially providing a dark matter candidate.
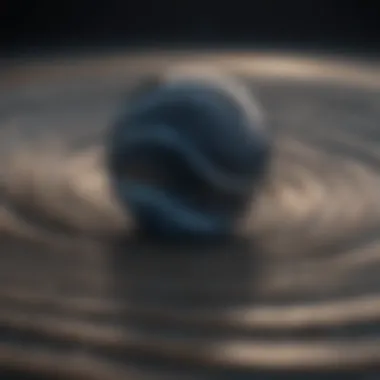
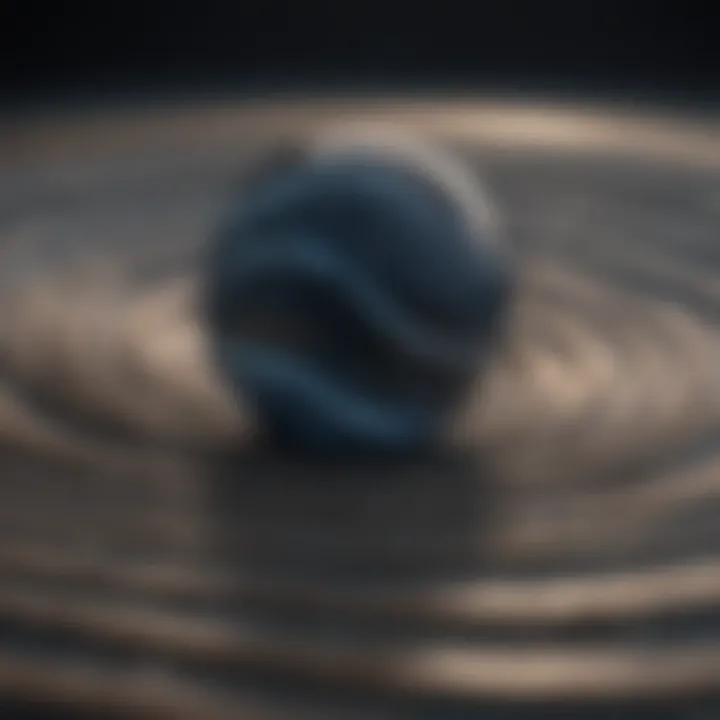
Another popular model is modified gravity theories, which propose alterations to our understanding of gravity at cosmological scales. For example, the MOND (Modified Newtonian Dynamics) theory posits that the laws of gravity change at very low accelerations, potentially explaining the observable effects attributed to dark matter without requiring its existence. These frameworks are crucial as they guide experimental efforts, seeking to validate these theories through empirical evidence.
"The nature of dark matter remains one of the biggest enigmas in physics. Understanding it could redefine our knowledge of the universe."
Recent Experimental Findings
Recent years have seen significant advancements in the search for dark matter, with numerous experiments striving to detect or infer its properties. The Large Hadron Collider (LHC) at CERN has conducted searches targeting WIMPs through high-energy collisions, although conclusive evidence is still pending.
Moreover, underground laboratories like the LUX-ZEPLIN experiment have been designed to detect dark matter interactions directly. This project aims to uncover the potential of WIMPs by using liquid xenon as a detection medium, lowering background noise from other particles. Recent updates indicate that researchers are getting closer to establishing parameters that would either confirm or rule out certain models of dark matter.
Additionally, astronomical observations, particularly from galaxy clustering and cosmic microwave background radiation, continue to provide indirect evidence of dark matter's existence. The studies of gravitational lensing—where light from distant galaxies is bent due to dark matter's gravitational influence—strengthen the argument for its presence.
These combined efforts and findings highlight the ongoing journey into understanding dark matter. As research progresses, future breakthroughs may unravel the mysteries surrounding this elusive component of our universe.
Developments in High-Energy Particle Physics
High-energy particle physics is a cornerstone of modern physics, providing profound insights into the fundamental components of matter. This field delves into the behavior and interactions of subatomic particles, which are the building blocks of everything in the universe. Recent advancements in this area are reshaping our understanding of the universe at both cosmological and quantum levels. Understanding these developments is crucial for students, researchers, and educators as it affects various scientific spheres, including cosmology, astrophysics, and even materials science.
Key Experiments at CERN
One of the leading research facilities in this field is CERN, the European Organization for Nuclear Research. The Large Hadron Collider (LHC) at CERN is the largest and most powerful particle accelerator in the world. Through collisions of protons at unprecedented energies, the LHC allows physicists to explore new realms of particle physics.
- Key experiments at CERN have yielded significant results, such as the discovery of the Higgs boson in 2012. The detection of the Higgs boson confirmed the existence of the mechanism that gives mass to other particles, which was a key prediction of the Standard Model of particle physics.
Additionally, CERN's experiments in heavy-ion collisions explore the state of matter in the universe just after the Big Bang, known as the quark-gluon plasma. These experiments help scientists understand the strong interaction, which binds protons and neutrons in an atomic nucleus.
"The success of the LHC demonstrates not just the power of our scientific curiosity, but also the effectiveness of international collaboration in pursuit of knowledge."
Implications of Higgs Boson Research
The implications of Higgs boson research extend far beyond confirming a significant theoretical model. It plays a vital role in understanding mass and its origins. Scientists propose that Higgs interactions are crucial for the universe as we know it. By studying the properties of the Higgs boson, researchers can test the limits of the Standard Model and search for new physics beyond it.
Key points regarding the implications include:
- Testing the Limits of the Standard Model: Results from Higgs boson studies may reveal discrepancies that suggest new particles or forces of nature.
- The Nature of Dark Matter: Understanding Higgs interactions could yield insights into dark matter, one of the universe's greatest mysteries.
- Future Generations of Particle Colliders: The findings from the LHC set the framework for future technology and experiments. Facilities like the Future Circular Collider may continue exploring beyond the Higgs boson.
Research in this domain not only deepens our understanding of the universe but also inspires the next generation of physicists, who will tackle the questions that remain unanswered. The conservation of energy, the role of fundamental forces, and the properties of matter are all prime areas where ongoing research will continue to evolve our scientific narrative.
The Role of Quantum Computing in Physics
Quantum computing stands at the intersection of physics and information technology, representing a profound shift in how we process and analyze data. Its significance lies in the unique capabilities it offers, especially when addressing complex problems that are intractable for classical computers. In the realm of theoretical physics, quantum computers hold promise for simulating physical systems that adhere to quantum mechanics, such as molecules and materials. This ability can lead to insights that may otherwise remain hidden using traditional computational models.
Furthermore, quantum computing can facilitate advancements in areas like optimization problems and machine learning. By harnessing quantum bits or qubits, which can represent multiple states simultaneously, these systems can perform calculations at unprecedented speeds. This extraordinary processing power can accelerate experiments and potentially revolutionize fields that depend on large data analysis.
Foundations of Quantum Computing
The foundations of quantum computing are rooted in the principles of quantum mechanics. Unlike classical bits that represent either a 0 or a 1, qubits can exist in a state known as superposition, where they embody both states simultaneously. This property greatly enhances computational capability.
One critical aspect of quantum computing is entanglement. This phenomenon allows qubits that are entangled to be interconnected, so that the state of one can instantly affect the state of another, regardless of the distance separating them. This can be fundamental for certain algorithms, providing enhanced speed and efficiency in problem-solving tasks.
A foundational model of quantum computing can be derived from well-known algorithms such as Shor’s algorithm, which efficiently factors large numbers, and Grover's algorithm, which searches unsorted databases quickly. These examples illustrate not only the principles of quantum mechanics at play but the potential practical implications as well.
Impact on Research Methodologies
As quantum computing technology matures, its impact on research methodologies in physics is becoming increasingly relevant. Traditional experimental methods often require significant time and resources. In contrast, quantum computing allows for more efficient designs and analyses of experiments.
This capability leads to an increase in research productivity, driven by the speed at which quantum computers can run simulations and optimize variables. Here are some specific ways quantum computing affects methodologies:
- Enhanced Simulation: Quantum computers can model physical systems at a level of detail unattainable by classical techniques.
- Rapid Prototype Testing: Researchers can use quantum computing frameworks to prototype and test theories faster.
- Data Analysis: The ability to sift through vast data sets quickly provides a competitive edge in research.
The transition to integrating quantum computing in research will likely lead to new discoveries and accelerated innovation in physics. The collaboration among physicists, computer scientists, and engineers will shape the future landscape of scientific inquiry.
Insights into the Nature of Black Holes
Understanding black holes is crucial in modern physics. They represent regions in space where gravity is so strong that nothing, not even light, can escape from them. This characteristic makes black holes both fascinating and challenging to study. They help scientists explore fundamental questions about the nature of space, time, and matter. Moreover, advancements in this field create a better understanding of our universe.
Observational Techniques and Discoveries
Recent developments in observational techniques have enhanced our ability to study black holes. One notable advancement is the Event Horizon Telescope (EHT) project. It used a global network of radio telescopes to capture the first image of a black hole's event horizon, located in the galaxy M87. The image provided compelling evidence for theories regarding black holes. Additionally, gravitational wave detections from the merging of black holes have further validated existing theoretical models.
New tools, such as very long baseline interferometry (VLBI) and advanced computational techniques, allow astronomers to gather and analyze data in unprecedented detail. As these technologies improve, researchers can collect more accurate information about the properties and behaviors of black holes, bringing us closer to deciphering their mysteries. Furthermore, analyzing how black holes interact with their surroundings provides critical insights into the processes governing galaxy formation and development.
Theoretical Implications for Physics
The study of black holes poses interesting theoretical implications for physics. They challenge classical notions of gravity and mechanics. For example, the existence of Hawking radiation suggests that black holes can emit radiation, potentially leading to their evaporation over time. This idea significantly impacts our understanding of thermodynamics and information conservation in quantum physics.
Furthermore, black holes serve as testing grounds for various fundamental theories, including general relativity and quantum mechanics. The discrepancies between these frameworks highlight gaps in our understanding of the universe. Addressing these gaps may lead to significant breakthroughs in unified theories of physics.
"Black holes are not just astronomical objects; they are laboratories for testing the laws of physics under extreme conditions."
As scientists extend their efforts to unravel black hole mysteries, they must confront complex questions regarding spacetime and the nature of reality itself. This has implications that resonate throughout many branches of science and could redefine our understanding of the universe.
The Standard Model and Beyond
The Standard Model of particle physics serves as a comprehensive framework that describes the fundamental particles and their interactions. It has been the cornerstone of modern physics. However, while it provides a robust structure that successfully explains many aspects of the subatomic world, it also has notable limitations that scientists strive to address. Recognizing these limitations helps physicists understand the boundaries of current theories and opens the door to new research pathways.
Limitations of the Standard Model
Despite its successes, the Standard Model encounters several significant issues:
- Inability to Incorporate Gravity: One of the most profound limitations is its inability to seamlessly incorporate gravitational forces into its framework. While it handles electromagnetism and the strong and weak nuclear forces proficiently, a theory of quantum gravity remains elusive.
- Dark Matter and Dark Energy: The Standard Model does not account for dark matter or dark energy, which together constitute a large percentage of the universe's total energy density. Without a clearer understanding of these components, the model leaves significant questions unanswered.
- Matter-Antimatter Asymmetry: The universe appears to be composed predominantly of matter rather than antimatter. The Standard Model provides insufficient explanations for this asymmetry, which poses a challenge to fundamental physics.
- Neutrino Mass: Neutrinos were traditionally thought to be massless; however, experiments have shown they have mass. The Standard Model does not adequately explain how neutrinos acquire mass, indicating gaps in our understanding of particle interactions.
These limitations signal the need for theories that go beyond the Standard Model. They invite physicists to explore new realms of particle physics, seeking a more unified understanding of the universe.
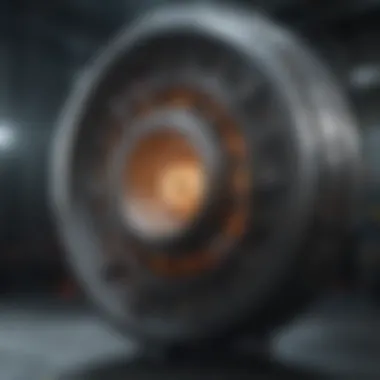
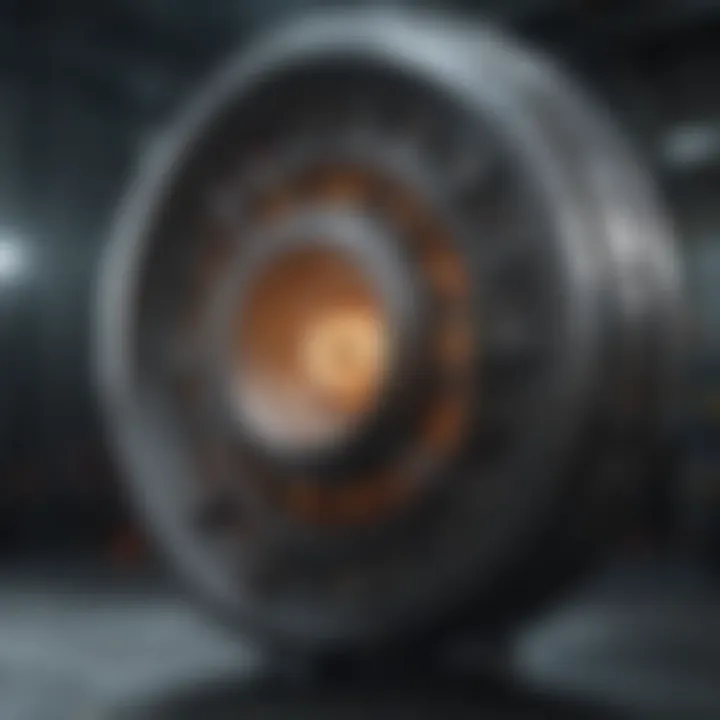
Emerging Theories and Hypotheses
Scientists are actively proposing new theories that aim to address the shortcomings of the Standard Model. These theories include:
- Supersymmetry: This theory suggests the existence of a partner particle for every known particle. It aims to resolve issues like the hierarchy problem and may provide a candidate for dark matter.
- String Theory: String theory proposes that all particles are one-dimensional strings rather than point-like particles. It integrates gravity within its framework and aims to unify all fundamental forces.
- Loop Quantum Gravity: Unlike string theory, loop quantum gravity does not require extra dimensions. It attempts to quantize spacetime itself and could provide a viable quantum gravity framework.
- Extra Dimensions: Some theories posit the existence of extra spatial dimensions beyond the traditional three. This idea could help explain the weakness of gravity compared to other forces.
As research progresses, the physics community remains hopeful that these innovative approaches will lead to new discoveries. The quest to expand the Standard Model is an integral part of modern physics. With each emerging theory, physicists inch closer towards a more complete comprehension of the structures that govern our universe.
"The search for a theory beyond the Standard Model is not just about adding complexity. It is about unearthing the deeper truths of the universe."
Field Developments in Cosmology
Field developments in cosmology represent critical advancements that can expand our understanding of the universe. This area is a blend of theoretical innovations and observational discoveries, which ultimately shape how we perceive cosmic phenomena. By investigating cosmology, researchers aim to address fundamental questions about the origin, evolution, and future of the universe. The significance of these developments is immense, as they can lead to new insights about dark energy, dark matter, and the overall structure of the cosmos.
One primary aspect of modern cosmology is the emphasis on interdisciplinary approaches, which have enhanced the collective knowledge across various scientific communities. As we study the universe, collaboration among physicists, astronomers, and data scientists becomes essential. This collaboration helps bridge gaps between theoretical physics and observational astronomy, allowing for a more cohesive understanding of cosmic revelations.
Expanding Universe Theories
The theory of the expanding universe is fundamental to contemporary cosmology. This concept originated from Edwin Hubble's observations in the 1920s, which indicated that galaxies recede from us, with their velocity proportional to their distance. This observation gave birth to the notion of expansions, leading to the formulation of the Big Bang theory.
Recent research has focused on how the universe’s expansion is not only ongoing but also accelerating. This acceleration is attributed to dark energy, a mysterious force that constitutes a major portion of the universe. Understanding the dynamics of dark energy remains a central focus for cosmologists.
New mathematical models are being developed to explore the implications of an accelerating universe. Various studies use observations from supernovae and cosmic background radiation to derive key parameters about the rate of expansion.
Advances in Cosmic Microwave Background Studies
The cosmic microwave background (CMB) is regarded as a vital relic from the early universe. It represents the remnant radiation from the Big Bang, providing a snapshot of the universe approximately 380,000 years after its formation. Advances in CMB research have been significant, particularly with the launch of missions like the Planck satellite, which has made precision measurements of temperature and polarization fluctuations.
These observations offer insights into the initial conditions that shaped the universe. They play a crucial role in testing cosmological models, assisting scientists in estimating fundamental parameters such as the universe's age, spatial curvature, and composition.
Moreover, anomaly detections in CMB data might uncover new physics beyond the existing models. Studies of the CMB continue to challenge our understanding of both dark matter and dark energy, posing exciting prospects for the future.
The exploration of expanding universe theories and cosmic microwave background studies not only enriches our knowledge of the universe's history but also prompts critical questions about its fate.
Innovations in Materials Science and Physics
Innovations in materials science have a crucial role in physics, often acting as a bridge between theory and practical application. These advancements not only enable enhanced functionalities of materials but also contribute to a deeper understanding of physical properties at the atomic and molecular levels. The focus is on developing new materials or enhancing existing ones, leading to significant implications in various fields such as electronics, renewable energy, and medical devices.
The exploration of material properties hinges on understanding interactions at the micro and nano scales. Therefore, the continuous innovation in this sector pushes the boundaries of what is possible in other scientific domains. For instance, new materials may lead to more efficient solar panels, stronger yet lightweight structures, or improved electronic components. The benefits of these developments are numerous:
- Increased Efficiency: Enhanced materials can significantly improve the efficiency of devices and systems.
- Sustainability: Many innovations focus on incorporating eco-friendly materials, contributing to environmental sustainability.
- Cost-Effectiveness: Advanced materials often reduce manufacturing costs over time due to their improved performance and longevity.
In this context, we delve into two major segments of materials science: high-temperature superconductors and nanostructured materials.
High-Temperature Superconductors
High-temperature superconductors have transformed the landscape of materials science. These materials can conduct electricity with zero resistance at relatively higher temperatures compared to traditional superconductors. This phenomenon has tremendous potential for applications in power transmission, magnetic levitation, and medical imaging techniques such as MRI.
The discovery and subsequent research into these superconductors can be linked directly to the developments of materials capable of functioning at elevated temperatures, making them more applicable for real-world uses. Researchers are exploring various compositions, aiming to uncover new superconductive properties that could operate at room temperature. This breakthrough could lead to a fundamental shift in energy systems—reducing energy loss and enhancing the capacity of electric grids.
Despite these promising advancements, challenges remain:
- Material Stability: Ensuring that high-temperature superconductors maintain their properties under practical conditions.
- Cost of Production: Finding efficient manufacturing processes that do not lead to prohibitive costs.
Nanostructured Materials and Their Properties
Nanostructured materials represent another significant area of innovation in materials science. These materials have unique properties that emerge when structures are engineered at the nanoscale. The manipulation at such dimensions alters aspects like strength, electrical conductivity, and thermal properties, leading to unprecedented enhancements in material performance.
The applications of nanostructured materials are extensive:
- Electronics: They allow the development of smaller, faster, and more efficient electronic devices.
- Medicine: In drug delivery systems, nanoparticles can improve the transport of medications directly to target cells, enhancing effectiveness and reducing side effects.
- Energy Storage: Nanostructured materials are pivotal in developing more efficient batteries and capacitors.
Ongoing research aims at understanding the mechanisms behind the unique behavior of these materials to further push the limits of technology. As new synthesis methods are developed, these materials promise a future where novel applications emerge on a regular basis.
"Innovations in materials science will shape the technologies of tomorrow, laying the groundwork for future discoveries across various scientific domains."
Renewable Energy and Physics
Renewable energy represents a crucial intersection between physics and sustainable development. With the increasing urgency to combat climate change, understanding and innovating in renewable energy technologies has become imperative. The role of physics in optimizing these technologies is fundamental. From harnessing solar and wind energy to improving efficiency in energy consumption, physicists analyze and solve complex problems that influence global energy strategies.
As societies transition from fossil fuels to renewable sources, physicists’ insights shape the research, development, and implementation of these technologies. Benefits extend beyond environmental sustainability; they include economic opportunities, energy security, and reduced dependence on non-renewable resources.
Thermodynamics and Efficiency
Thermodynamics plays a vital role in renewable energy systems, influencing how energy is captured, converted, and used. The principles of thermodynamics guide engineers and scientists in optimizing power generation systems, such as heat engines and photovoltaic cells. Understanding energy transfer, conversion efficiency, and loss mechanisms is crucial.
Efficiency improvements can be achieved by:
- Evaluating heat loss in thermal systems
- Optimizing materials for energy conversion
- Innovating storage solutions for intermittent renewable sources
Through thermodynamics, researchers develop strategies to maximize energy efficiency, leading to reduced operational costs and enhanced performance.
Innovations in Solar Cell Technology
Recent advancements in solar cell technology signify a leap toward more effective renewable energy harnessing. Traditional silicon-based cells are being supplemented by newer materials, such as perovskites, which show promise in improving solar energy conversion efficiency.
The implications of these innovations are substantial:
- Higher Efficiency: New materials can achieve higher efficiency rates compared to traditional models.
- Lower Production Costs: Developing cheaper materials can lower the overall cost of solar panels, making them more accessible.
- Flexible Applications: Innovations allow for more versatile applications, from residential rooftops to large-scale solar farms.
Overall, continuous research in solar technologies illustrates the synergy between physics and technological development in addressing energy challenges.
Applied Physics in Technology and Engineering
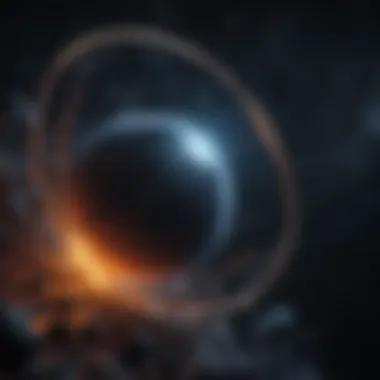
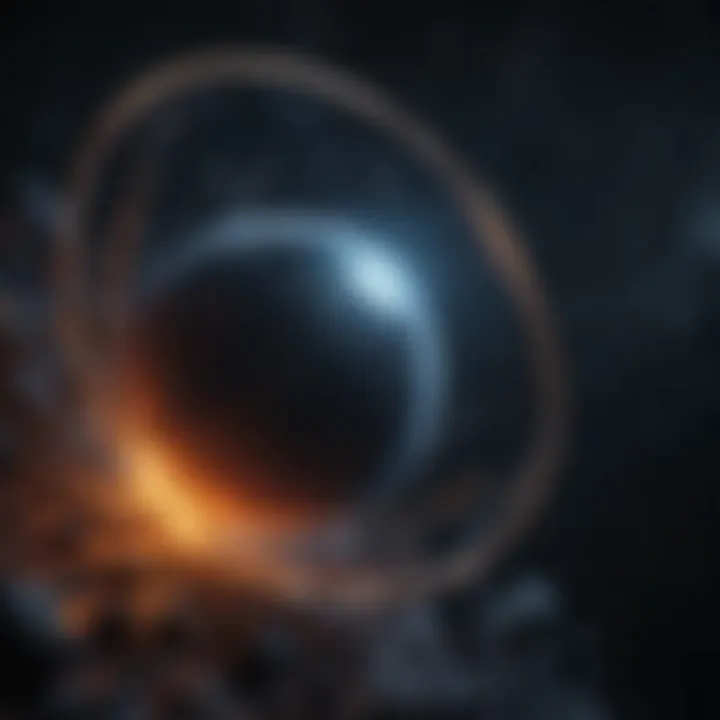
Applied physics plays a crucial role in bridging the gap between theoretical physics and practical engineering applications. This discipline emphasizes the use of physical principles in the development and enhancement of technology. Understanding this topic is vital because it illustrates how abstract theories can lead to real-world innovations that transform industries and improve everyday life.
Specific Elements in Applied Physics:
- Interaction of physics principles with engineering challenges.
- Development of new materials and technologies.
- Solving real-life problems using scientific methods.
Applied physics serves as a foundation for a myriad of engineering disciplines. This includes civil, mechanical, aerospace, and electronics engineering. Researchers and engineers often rely on applied physics to develop technologies that evolve systems and processes, propelling the industry forward.
Benefits of Applied Physics:
- Facilitates efficient technology transfer from laboratories to industries.
- Informs design processes for better product performance.
- Supports innovation in renewable energy systems and automation.
Understanding applied physics enables professionals to harness scientific knowledge effectively. This knowledge leads to advancements in areas such as telecommunications, computing, and biomedical engineering. As technology continues to advance, the need for expertise in applied physics becomes increasingly significant.
Emerging Technologies and Their Applications
As the landscape of technology evolves, emerging technologies are reshaping industries and redefining what is possible. Innovations like nanotechnology, smart materials, and biotechnology are at the forefront. These technologies arise from a careful application of physical principles to solve complex issues.
Nanotechnology, for instance, plays a key role in medicine, electronics, and materials science. It involves manipulating matter at an atomic or molecular scale.
Benefits include:
- Enhanced drug delivery systems.
- Improved energy storage solutions.
- Development of stronger and lighter materials.
"Emerging technologies, rooted in applied physics, have the potential to revolutionize not just technology but the way we interact with the world."
Smart materials, which can alter their properties in response to environmental changes, are another exciting area. They are used in various applications, including structural monitoring and responsive consumer products.
Advances in Robotics and Automation
The field of robotics and automation has witnessed tremendous growth in recent years. Advances in applied physics have directly influenced the design and functionality of robots.
Some key areas of advancement are:
- Sensor technologies for better perception.
- AI integration for smarter decision-making.
- Enhanced mobility and dexterity.
These innovations lead to increased efficiency and safety in sectors like manufacturing, healthcare, and logistics.
Improvements in sensors allow robots to interact with their environment more accurately. This results in application in surgeries or difficult tasks in hazardous environments.
The Interdisciplinary Nature of Physics
Physics is not an isolated discipline; it is deeply intertwined with various other fields of study. This multidisciplinary nature is crucial for understanding complex phenomena. In recent years, the intersection between physics and other sciences has led to significant breakthroughs. These collaborations expand the horizons of traditional physics, allowing for innovative approaches to old problems. For researchers and students alike, grasping the interdisciplinary nature could enhance their insights into physics.
"Interdisciplinary research fosters creativity and innovation, leading to discoveries that would be impossible in a single field."
Collaboration Across Scientific Fields
The collaboration between physics and other scientific disciplines is vital in advancing knowledge. For instance, the synergy between physics and biology has invigorated fields like biophysics. By employing physical principles, biophysicists analyze biological structures and processes at a molecular level. This approach unveils important insights into the mechanisms of life. Furthermore, physics plays a significant role in chemistry through quantum mechanics. Understanding electron behavior leads to advancements in material science and nanotechnology.
Another important collaboration involves physics and computing. The rise of quantum computing combines principles of quantum mechanics and computer science. This merger aims to solve problems deemed intractable by classical computers. Moreover, interdisciplinary teams often lead to the development of algorithms that improve simulations and analytical capabilities across various fields.
Physics and Its Influence on Other Disciplines
Physics exerts a profound influence on several other disciplines. In environmental science, understanding thermodynamics is essential for studying climate change and energy dynamics. Researchers apply concepts from physics to develop sustainable energy solutions. In medicine, physics principles underpin imaging technologies like MRI and CT scans, which are vital for medical diagnostics.
Moreover, the field of economics is increasingly exploring concepts from physics to model complex systems. Techniques such as statistical mechanics are used to analyze market behaviors. This intersection allows economists to better understand fluctuations and trends in finance.
Future Directions in Physics Research
Physics is a dynamically evolving discipline, consistently pushing the boundaries of our understanding of reality. The future directions of research in physics hold great significance, as they not only extend the frontiers of knowledge but also potentially lead to transformative technologies. Researchers are now looking at new theories and experimental setups to answer unresolved questions that lie at the core of modern physics.
Key topics include quantum information science, gravitational physics, and the quest for a unifying theory that bridges general relativity and quantum mechanics. These areas promise to generate insights about the fundamental constituents of the cosmos and the nature of time and space itself.
Emerging Areas of Study
As the landscape of physics research continues to evolve, several emerging areas are gaining attention:
- Quantum Technologies: Integration of quantum mechanics into practical technology is developing rapidly. Quantum sensors and quantum communication hold promise for enhanced measurement techniques and secure information transfer.
- Astrophysical Phenomena: Observations from the Event Horizon Telescope and gravitational-wave detectors have opened the door to studying black holes and cosmic events in unprecedented detail.
- Supersymmetry and String Theory: Researchers are revisiting early theories like supersymmetry and string theory, aiming to provide explanations for dark matter and the unification of forces.
- Nanotechnology in Physics: Advances in fabrication techniques are leading to the development of novel materials with unique properties, fueling innovations in energy storage and conversion.
These areas reflect a growing trend toward interdisciplinary approaches, linking physics with chemistry, material science, and engineering. The dialogue between disciplines fosters innovation and facilitates solving complex problems that transcend traditional boundaries.
Challenges for Future Physicists
While the possibilities are promising, challenges loom ahead for the next generation of physicists.
- Interdisciplinary Understanding: As physics overlaps with other scientific fields, future physicists must not only be proficient in physics but also understand concepts from allied disciplines like mathematics, biology, and computer science.
- Funding and Resources: Research in cutting-edge areas often requires significant funding. Securing grants can become a daunting task, especially as competition intensifies for limited funding pools.
- Ethical Implications: With advancements in technology, especially in quantum computing and AI, ethical considerations grow more pressing. Physicists will need to address the implications their research holds for society and the environment.
- Complexity in Experimentation: As experiments in physics grow more sophisticated, the risks of misinterpretation increase. The complexity surrounding large projects, such as those at CERN, requires meticulous planning and collaboration.
"The future of physics research is not solely driven by the pursuit of knowledge but also by the responsibility that knowledge entails."
Epilogue
In this article, the conclusion serves a crucial role in synthesizing the knowledge gained from recent developments in the realm of physics. This section wraps up the discussions, reflecting on various discoveries and their significance to our understanding of the universe. It is essential to highlight that every breakthrough discussed leads to further questions and investigations, thus underlining the iterative nature of scientific research.
Summary of Key Findings
Across the sections, several key findings have emerged:
- Quantum entanglement has unveiled profound connections within quantum systems, prompting reevaluation of fundamental concepts.
- The advancements in gravitational wave detection have not only confirmed general relativity but also opened a new window into cosmic observations.
- Research into dark matter continues to evolve with innovative experimental approaches that may soon reveal its elusive nature.
- Developments in high-energy particle physics are reshaping our understanding of the fundamental components of matter and forces.
- Quantum computing is set to revolutionize research methodologies, enabling simulation and analysis on a scale not previously possible.
- Insights into black holes are refining our theoretical models, while generating new questions about spacetime.
These findings represent only a fraction of the ongoing journey in physics, yet each one contributes significantly to the bigger picture of the scientific narrative.
Implications for Society and Future Research
The importance of these discoveries extends beyond academic circles. Each breakthrough has potential implications for several aspects of society:
- Technological Advancements: Innovations in areas like quantum computing and materials science can lead to new technologies, enhancing everyday life.
- Interdisciplinary Influence: Discoveries in physics often stimulate advancements in related fields, such as engineering, medicine, and environmental studies, thus fostering collaboration.
- Educational Opportunities: The complexity and dynamism of modern physics can inspire educational reform, promoting critical thinking and scientific literacy among students.
Looking forward, the challenges posed by these discoveries indicate that future researchers will need to tackle intricate questions. Addressing matters such as the true nature of dark matter or the realities of quantum mechanics requires relentless curiosity and collaboration across scientific disciplines. The findings and implications laid out here reaffirm the vital role physics plays not only in our understanding of the cosmos but in shaping the very fabric of future technologies and societal progress.