Comprehensive Guide to Western Blot Techniques
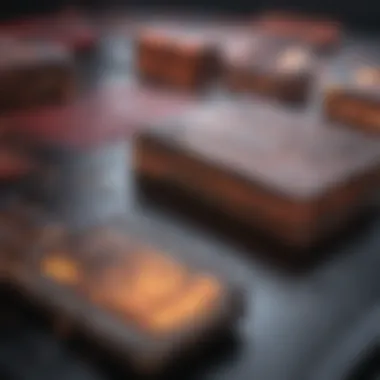
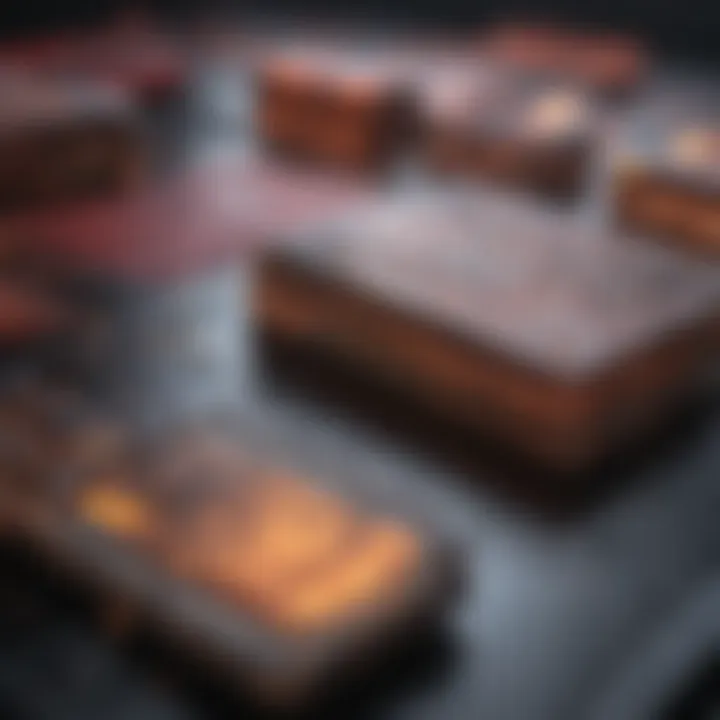
Intro
Western blotting has emerged as a cornerstone technique in molecular biology, critical for the detection and analysis of specific proteins within complex mixtures. This method holds a particular appeal due to its ability to deliver not only qualitative but also quantitative insight into protein expression. Over the years, despite the proliferation of various modern methods, the Western blot remains highly regarded for its robustness and reliability in the research community.
While the procedure is deceptively straightforward, the intricacies involved—from sample preparation through to detection—demand attention to detail and technical precision. Understanding these nuances is essential, not just for successful application but also for troubleshooting and optimizing results, which can often be marred by common pitfalls.
In this article, readers will explore the various milestones of the Western blotting process. We will delve deep into methodologies, equip researchers with practical insights, and discuss best practices that can transform the execution of this vital technique. Moreover, common challenges faced during the procedure will be examined, along with potential solutions that promote success.
With that said, let's embark on our journey through the methods that make Western blotting a revered player in the landscape of protein analysis.
Prelims to Western Blotting
In the realm of molecular biology, Western blotting stands as a fundamental technique for protein detection and analysis. It’s not just a method; it’s a bridge between the theoretical and practical applications in biochemistry and genetics. Western blotting allows researchers to take a peek into the protein world, shedding light on the intricacies of cellular processes and disease mechanisms. By revealing the presence and quantity of specific proteins, this technique can answer critical questions about cellular function and regulation.
Historical Context
To truly appreciate the importance of Western blotting, one can trace its roots back to the late 1970s. Developed by W. Keith Boyle and later refined by others, it initially filled a void in the scientific community’s capabilities to detect proteins with specificity. At that time, methods for protein analysis were limited; scientists were in desperate need of a technique that could provide clarity in complex biological systems.
The evolution of Western blotting can be seen as a response to the challenges researchers faced when studying proteins. It gained prominence in the following decades, becoming a staple in many labs worldwide. The development of polyacrylamide gel electrophoresis paved the way for this technique, as it allowed for the separation of proteins based on their size and charge. Researchers combined this powerful method with antibody-based detection to create a tool that transformed our understanding of molecular biology.
Importance in Molecular Biology
Western blotting is not just a relic of the past; it has remained crucial in modern-day research. Its versatility is evident in various fields, from oncology to immunology. It allows detection of proteins with high specificity, enabling scientists to observe protein expression levels, modifications, and interactions within a biological sample.
"Western blotting is indispensable in the toolbox of molecular biology; it provides data that can elucidate complex biological problems."
The significance of Western blotting extends to:
- Disease Diagnosis: It plays a crucial role in identifying disease markers, like in the case of HIV detection where antibodies are targeted.
- Research: In studies aimed at understanding signaling pathways or protein interactions, Western blotting serves as a method for validating findings.
- Quality Control: It ensures that recombinant proteins expressed in cell lines are produced correctly and in sufficient quantities.
Furthermore, Western blotting isn’t without its challenges, which adds layers of expertise required to produce reliable results. Factors like non-specific binding and sensitivity issues can hamper even the most skilled researchers. Understanding these nuances makes it clear: the importance of Western blotting lies not only in its utility but also in the knowledge it imparts to users about their experimental systems.
Principle of Western Blotting
Understanding the principle of Western blotting is paramount for anyone venturing into the field of molecular biology. This technique serves as a cornerstone for identifying and analyzing proteins within complex biological samples. In essence, it operates on the premise that proteins can be separated based on their size and charge, allowing researchers to pinpoint specific proteins of interest. The beauty of Western blotting lies in its ability to provide a visual representation of protein expression levels, offering insights that can lead to significant discoveries in the realm of biomedical research.
Basics of Protein Detection
At its core, protein detection through Western blotting is a methodical journey. Initially, proteins are extracted from cellular or tissue samples and then subjected to gel electrophoresis. Here, proteins migrate through a gel matrix, which separates them according to their size. The smaller proteins will travel farther than the larger ones, creating a distinct banding pattern on the gel.
Once proteins are separated, they undergo transfer to a membrane, where they are immobilized. This process is crucial because it transitions proteins from the gel, where they are often indistinguishable, to a medium that allows antibody binding.
This technique hinges on the principles of specificity and sensitivity. The exemplification of protein presence is made possible through antibodies, which are proteins designed to bind selectively to their target antigens. Hence, the interaction between the antibody and its respective protein acts as a beacon, illuminating the proteins of interest for further analysis.
In summary, the basics of protein detection in Western blotting can be summarized with the following steps:
- Sample preparation: Extract proteins from cells or tissues.
- Gel electrophoresis: Separate proteins based on size and charge.
- Transfer: Shift separated proteins to a membrane.
- Detection: Use antibodies to identify specific proteins.
Role of Antibodies
Antibodies are often labeled as the soul of Western blotting. Without them, the technique would lose its ability to discern one protein from another, reducing its efficacy dramatically. These Y-shaped proteins are produced by the immune system and are inherently designed to combat pathogens. In the context of Western blotting, they are harnessed for their highly specific target affinity.
Their role begins post-transfer, where specific antibodies are introduced to the membrane containing the immobilized proteins. Each antibody recognizes a unique epitope or a particular region on a protein. This interaction can be fragile, but it is also highly specific. There are primarily two types of antibodies used in Western blotting:
- Primary antibodies: Directly bind to the target protein, providing the initial detection phase.
- Secondary antibodies: Bind to the primary antibodies and are typically conjugated to a detection element, amplifying the signal.
The amplification aspect is noteworthy because it enhances the visibility of the target protein. This means that even small amounts of proteins can be detected efficiently, which can be particularly crucial when studying rare proteins or low-abundance targets. Furthermore, the use of different detection methods, such as chemiluminescence or fluorescence, can further elevate the resolution and sensitivity of the results obtained from the Western blot.
In summary, the role of antibodies in Western blotting is crucial. They contribute specificity, enhance sensitivity, and ultimately help in revealing the story behind the protein expression in the studied samples.
"Antibodies are not just tools; they are the keys that unlock the hidden narratives of proteins within biological samples."
Sample Preparation Techniques
In the realm of Western blotting, the significance of sample preparation cannot be overstated. Proper sample preparation is critical as it lays the foundation for accurate protein analysis. Different sample types require distinct handling and extraction processes, which can greatly affect the reproducibility and reliability of results. Factors such as the choice of lysis method, the efficiency of protein extraction, and the subsequent handling of samples all contribute to the quality of the final blot. Without a well-prepared sample, even the most advanced detection systems might yield misleading data.
Types of Samples
Understanding the various types of samples used in Western blotting is necessary for selecting the appropriate strategy for protein detection.
Cell Lysates
Cell lysates are a favored choice in Western blotting due to their high protein availability and simplicity. They are created by breaking open cells to release their contents, yielding a rich source of proteins for analysis. The key characteristic of cell lysates is their versatility; they can be derived from a variety of cell types including cultured cells, primary cells, or even transformed cell lines. One of the notable advantages of using cell lysates is the ability to study specific signaling pathways and metabolic processes, making them invaluable in research focused on cell biology.
However, it’s important to note that the method of lysis can influence the yield and integrity of proteins. If the sample isn’t handled meticulously, it can lead to the degradation of sensitive proteins, which may skew results.
Tissue Extracts
Tissue extracts provide a whole-new level of complexity when it comes to Western blotting. They are derived from solid tissues, thus offering a diverse representation of protein expression in a specific organ or region. The key feature that makes tissue extracts advantageous is their ability to reflect physiological conditions more accurately than cultured cells. By using tissue extracts, researchers gain insights into protein interactions in their native environments, which is critical for translational research.
On the flip side, obtaining tissue extracts can be more laborious compared to cell lysates. The extraction process may require more extensive purification steps, and the variability in tissue samples can lead to inconsistent results if not standardized properly.
Recombinant Proteins
Recombinant proteins are engineered proteins produced using recombinant DNA technology. They are a powerful tool in Western blotting, especially for producing specific antibodies. One major characteristic of recombinant proteins is their uniformity. Unlike naturally occurring proteins, which may vary in structure due to their biological source, recombinant proteins have a consistent composition which makes them ideal for experiments that demand high specificity.
Using recombinant proteins presents the benefit of studying proteins in isolation, without the influence of cellular context that might alter behavior. However, a downside is that they may not entirely mimic the biological relevance observed in native proteins due to their artificial expression systems.
Methods of Lysis
To extract proteins effectively, several methods of lysis can be employed. The choice of method impacts the yield, purity, and functionality of the proteins extracted, which ultimately affects the entire Western blotting process.
Chemical Lysis
Chemical lysis utilizes specific reagents to solubilize cell membranes and release proteins. This method is beneficial because it allows for the inclusion of protease inhibitors that can help maintain protein integrity during the extraction process. A key feature of chemical lysis is its ability to preserve post-translational modifications, which are crucial for understanding protein functions.
However, one downside is that the chemical agents can also interfere with downstream applications if not removed properly post-lysis. For instance, certain detergents commonly used in chemical lysis can affect binding interactions during antibody incubation.
Physical Disruption
Physical disruption methods employ mechanical means to break open cells, such as bead milling or sonication. This type of lysis can be rapid and efficient, allowing for more intact proteins to be harvested quickly. The key characteristic that makes physical disruption a popular choice is control; the researcher can fine-tune energy input to optimize cell lysis without compromising protein integrity.
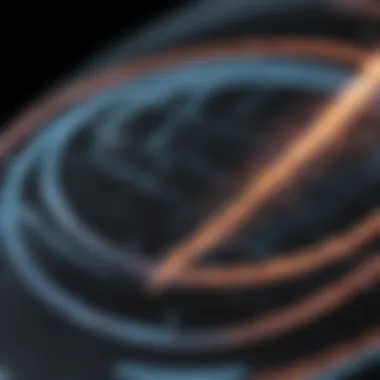
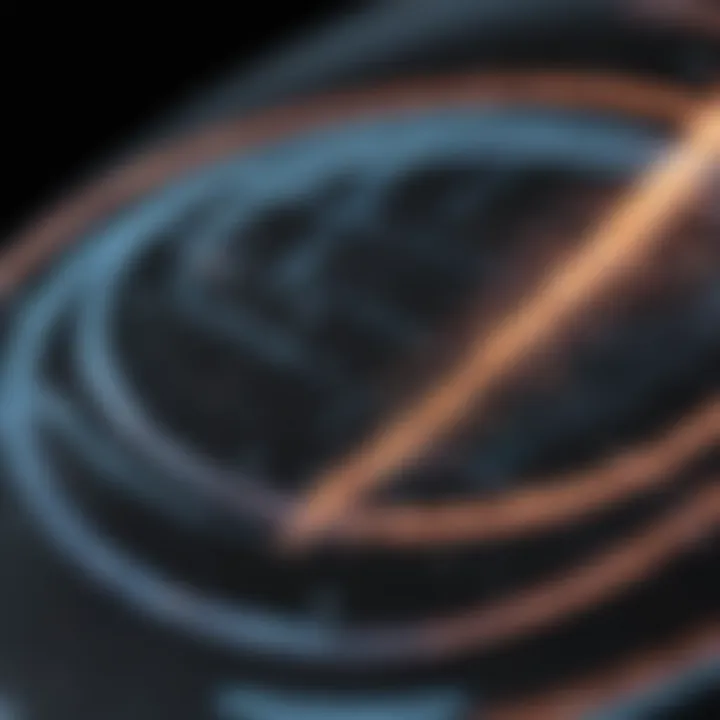
Nevertheless, this method sometimes leads to shear stress that can damage sensitive proteins or create unwanted artifacts. Thus, balancing effectiveness and gentleness is paramount when using physical disruption techniques.
Gel Electrophoresis Process
The gel electrophoresis process serves as a cornerstone in Western blotting, acting as the vital step where proteins are separated based on their size and charge. This separation is not just a technical necessity; it lays the groundwork for the subsequent stages of detection and analysis. Understanding how gel electrophoresis works and its nuances is crucial for researchers aiming to achieve accurate and reproducible results in their protein studies. Without this step, the entire procedure would fall apart, leading to ambiguity in results and interpretations.
Types of Gels Used
Different types of gels are employed in gel electrophoresis, and the choice can significantly influence the outcome of the protein separation. Here, we take a closer look at SDS-PAGE and Native PAGE, two of the most commonly used methods.
SDS-PAGE
SDS-PAGE, or Sodium Dodecyl Sulfate Polyacrylamide Gel Electrophoresis, is a method that enables the separation of proteins primarily based on their molecular weight. One of the standout characteristics of SDS-PAGE is its use of the anionic detergent SDS, which denatures proteins and imparts a uniform negative charge proportional to their size. This standardization is what makes SDS-PAGE such a popular choice in Western blotting; it simplifies the analysis of protein samples, ensuring that size, not charge, dictates their movement through the gel.
The primary advantage of SDS-PAGE lies in its effectiveness and reproducibility. Researchers can reliably separate proteins ranging from a few kilodaltons to several hundred kilodaltons, making it versatile for various applications. That said, it does have some drawbacks, such as the inability to study protein interactions and complex formations, since the proteins are denatured.
Native PAGE
Native PAGE is another valuable method, offering a different perspective on protein behavior. Unlike SDS-PAGE, it preserves the native state of proteins, meaning they maintain their natural configuration and charge throughout the electrophoretic process. This feature makes Native PAGE advantageous for studying protein-protein interactions, enzyme activities, and complex formations, where the physiological state of the protein is crucial for meaningful insights.
However, Native PAGE comes with its challenges. The results can be less reproducible than those obtained from SDS-PAGE due to variation in protein conformation and intrinsic charge. This inconsistency can pose challenges in quantifying protein concentrations accurately, thereby necessitating an experienced hand for interpreting results effectively.
In summary, both SDS-PAGE and Native PAGE offer distinct approaches to gel electrophoresis, each catering to unique research needs. The selection of gel type hinges on the specific requirements of the study. Researchers must weigh the benefits and shortcomings of each to determine the most suitable technique for their experimental goals.
Loading and Running the Gel
Once the gel is prepared, the next crucial step involves loading the samples and running the gel. This stage determines how effectively the proteins migrate and separate, impacting the quality of the output data. A delicate hand and precision are critical; even minor inconsistencies during loading can skew results significantly.
During this process, samples are mixed with a loading buffer, typically containing a tracking dye that enables visualization of the migration front. Careful pipetting techniques ensure that samples are deposited at the bottom of the gel wells without disturbing adjacent wells, which could lead to cross-contamination and blurred bands.
Once the samples are loaded, the gel is submerged in an appropriate buffer solution, allowing the electrical current to facilitate the movement of proteins through the gel matrix. Factors such as voltage, time, and buffer composition all influence the efficiency of separation. Researchers often optimize these parameters for best results, always keeping an eye on the balance between speed and resolution.
Monitoring the progression of the dye band throughout the run provides a visual cue, ensuring that separation is occurring as expected. It’s a dance of molecules, where every step matters – and it sets the stage for the next phase, where proteins will be transferred and further analyzed.
"Understanding the gel electrophoresis process is not just about following steps; it's about mastering the art of separation, one gel at a time."
By closely attending to the intricacies of loading and running the gel, researchers pave the way for precise interactions in the subsequent Western blotting steps.
Transfer Techniques
Transfer techniques are a crucial stepping stone in the Western blotting process, bridging the gap between gel electrophoresis and the detection phase. The efficiency of transferring proteins from the gel to a membrane impacts the subsequent detection and analysis, making it a key area of focus for researchers. Here, we will discuss various transfer methods, their distinct characteristics, and how they contribute to enhancing the overall outcome of Western blotting.
Methods of Transfer
Wet Transfer
Wet transfer is perhaps the most traditional of the transfer techniques. It involves placing the gel and membrane in a buffer solution and applying an electric field across them. One of the main benefits of wet transfer is its efficiency in transporting proteins, especially those with larger sizes, from the gel to the membrane.
The key characteristic of wet transfer is its reliance on a full immersion setup. This not only ensures a uniform distance between the gel and the membrane but also facilitates optimal transfer conditions. Many researchers prefer this method owing to its stability and reproducibility.
A unique feature of wet transfer is the ability to create a gel sandwich that maintains a constant buffer flow, which can help prevent protein aggregation during the transfer process. However, while wet transfer is widely used, it has its drawbacks; it can be time-consuming and require more reagents than other methods.
Semi-Dry Transfer
Semi-dry transfer presents a more contemporary approach, often celebrated for its speed and efficiency. In this method, the gel is sandwiched between a membrane and filter papers, and the transfer occurs in a dry environment, with only a small volume of buffer used.
The key aspect of semi-dry transfer is its reduced transfer time, making it suitable for high-throughput experiments. This method has gained popularity in labs where time constraints are a factor, and results are needed promptly.
One unique feature is the ability to easily vary the voltage settings during the transfer process. While faster, semi-dry transfer can sometimes lead to uneven protein migration or losses due to insufficient buffer in the system, which is a consideration researchers must keep in mind.
Dry Transfer
Dry transfer methods have recently entered the spotlight as a convenient alternative. This method employs specific devices designed to move proteins from the gel to a membrane without the usage of liquid buffers, offering ease of operation and clean setup.
The primary characteristic of dry transfer is its simplicity and minimalistic approach, which can be attractive for labs looking to streamline their processes. Also, since there's no liquid buffer, the potential for contamination is lowered.
However, a unique challenge with dry transfer is ensuring complete protein transfer, which could lead to variability in results. This method, while accessible, might not provide the same level of protein recovery for all types of proteins compared to wet or semi-dry transfers.
Factors Affecting Transfer Efficiency
When discussing transfer techniques, several external variables can significantly influence the efficiency of protein transfer:
- Voltage and Time: The applied voltage and the duration of the transfer are critical variables. An optimal setting ensures effective protein movement without compromising integrity.
- Gel Composition: Different gels (e.g., SDS-PAGE) may require distinct adjustment in transfer protocols to prevent protein loss.
- Membrane Type: Choosing the right membrane (PVDF vs. nitrocellulose) plays an essential role in protein binding efficiency.
- Buffer Composition: The ionic strength and pH of the buffer can impact the overall efficacy of the transfer.
- Temperature: Higher temperatures can sometimes enhance transfer rates, but at the risk of damaging proteins.
Understanding these factors provides insight into how researchers can optimize their transfer protocols, ultimately enhancing the reliability of Western blotting results.
"Effective transfer techniques are the linchpin of successful Western blotting and can make or break the analysis results."
Each transfer method has its strengths and weaknesses, and the choice often depends on the specific requirements of the experiment. Mastery of these methods paves the way for accurate protein analysis, underlining the importance of this stage in the Western blotting process.
Blocking and Incubation Steps
Blocking and incubation steps are pivotal to the successful execution of Western blotting. These phases are designed to minimize background noise and ensure that the results reflect the true expression of proteins of interest. Without proper blocking, antibodies can bind non-specifically, leading to misleading data. The ultimate aim during these steps is to create a controlled environment where only the targeted molecules will engage with the antibodies, providing clearer and more precise results.
Blocking Agents
Bovine Serum Albumin
Bovine Serum Albumin, commonly referred to as BSA, is a protein derived from cows that serves as a widely used blocking agent in Western blotting. One key characteristic of BSA is its ability to effectively saturate the available binding sites on the membrane, thereby preventing non-specific binding of antibodies during incubation.
The benefits of using BSA include its high solubility in buffer solutions and its affordability, making it a popular choice in many laboratories. Moreover, BSA is known to maintain good stability under various storage conditions, which adds to its practicality in research settings. However, it's worth noting that one disadvantage of BSA might be its potential to block some specific interactions, particularly with certain antibodies.
A unique feature of BSA lies in its versatility; it can also be used in various concentrations depending on the specific experimental needs. This adaptability allows researchers to optimize their protocols effectively.
Non-fat Dry Milk
Non-fat dry milk is another widely adopted blocking agent, particularly favored for its cost-effectiveness and simplicity. One of its primary attributes is its rich nutrient profile, which can help to diminish background noise in samples where proteins may have various affinities to the membrane.
The main advantage of non-fat dry milk is that it’s readily available and easy to work with, providing a straightforward solution for blocking. It also tends to have a neutral pH, which is conducive for various types of experiments. However, it has a drawback: some antibodies may not perform optimally in the presence of milk proteins, which can affect the sensitivity and specificity of detection.
Moreover, a unique aspect of non-fat dry milk is its effectiveness in blocking innate antibodies that might arise from serum samples. Researchers frequently use it as a primary option when sensitivity isn’t the central concern.
Antibody Incubation
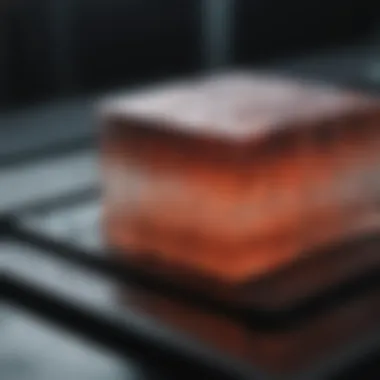
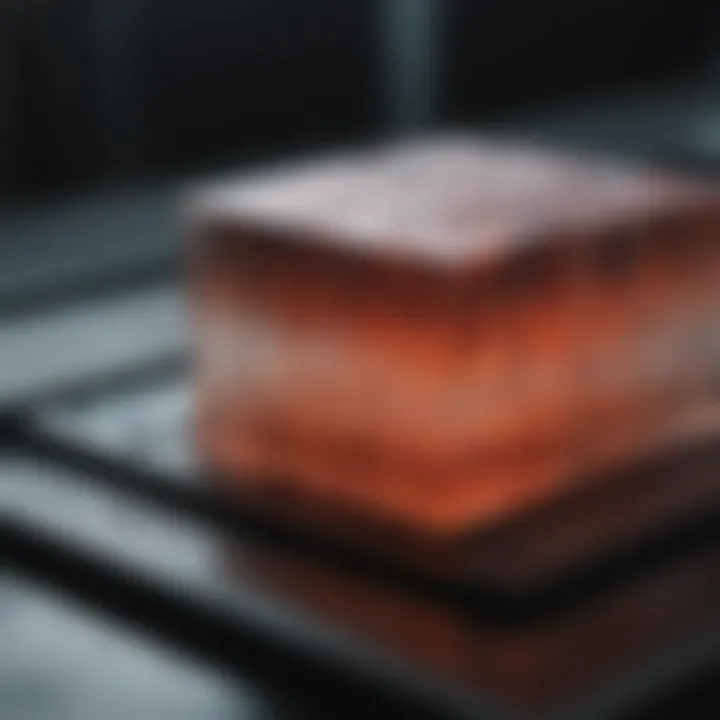
Antibody incubation is a fundamental step in the Western blot process. During this stage, the selected primary antibodies are introduced to the membrane containing the separated proteins. The concept here is straightforward: allow the antibodies to find their specific targets and bind to them, a process often enhanced by optimal conditions such as temperature, duration, and dilution.
When incubating antibodies, two primary considerations come into play: duration and temperature. Typically, overnight incubation at 4°C is common, as it helps in maximizing binding affinity while minimizing background interference. In some scenarios, shorter incubations at room temperature can be acceptable, though the signal might not be as strong or clean.
Additionally, the antibody dilution is crucial. Too concentrated an antibody can lead to excessive background staining, while too dilute may produce weak signals. Hence, fine-tuning dilution factors based on prior experimentation or using titration methods often yields the best results.
Detection Methods
The detection methods utilized in Western blotting are pivotal, serving as the final link in the chain of processes that transform raw data into meaningful results. These methods help researchers determine the presence, quantity, and functional status of proteins in a sample, offering insights into biological pathways and disease mechanisms. Understanding the nuances of detection methods is essential, as each technique offers distinct benefits and certain limitations that can influence experimental outcomes.
Chemiluminescence
Chemiluminescence is perhaps the most widely employed detection method in Western blotting. It relies on a chemical reaction that emits light, allowing for the visualization of protein bands on a membrane. The method is highly sensitive, often capable of detecting picogram levels of protein. This sensitivity makes it particularly valuable in situations where protein expression is low or when the subject of interest is expressed at very low levels.
When using this approach, researchers typically employ horseradish peroxidase (HRP)-conjugated antibodies, which react with a substrate to produce light. The resulting luminescence can be captured using photographic film or digital imaging systems, thus facilitating protein quantification. However, one thing to keep in mind is that the signal can diminish over time, necessitating prompt imaging.
"Chemiluminescence shines a light, literally, on the proteins of interest, revealing their presence with remarkable sensitivity."
Fluorescence
Fluorescence detection is another powerful method that hinges on the unique property of certain molecules to emit light upon excitation. In Western blotting, this usually involves the use of fluorescently labeled antibodies that bind to the target proteins. The primary advantage here is the ability to analyze multiple proteins simultaneously through different excitation wavelengths, a feature that offers considerable efficiency and depth in data collection.
The imaging equipment for fluorescence detection often consists of specialized cameras and filters that isolate the wavelengths emitted from the fluorescent dyes. This means that researchers can obtain a wealth of information from a single blot, making it attractive for complex studies involving multiple biomarkers. However, care must be taken with sample handling, as fluorescent signals can be lost due to photobleaching or improper storage conditions.
Colorimetric Detection
Colorimetric detection is the oldest of the three methods but remains popular due to its simplicity and cost-effectiveness. This approach typically involves the use of enzyme-linked antibodies, similar to chemiluminescence, but instead of generating light, these reactions result in a colored precipitate visible by eye or through a spectrophotometer.
While colorimetric detection is definitely more accessible than its luminescent or fluorescent counterparts, it lacks the sensitivity levels that are sometimes necessary for certain experimental scenarios. For researchers dealing with low-abundance proteins or those needing precise quantification, this method might not be the best choice. Nonetheless, its ease of use and readily available reagents make it a go-to for many routine applications in molecular biology.
Common Challenges in Western Blotting
Western blotting serves as a cornerstone in molecular biology, especially when it comes to protein detection and analysis. Its importance is widely recognized, yet the path to achieving clean, repeatable results can be fraught with challenges. Understanding these common troubles can help researchers fine-tune their methodologies, optimize protocols, and improve the robustness of their findings. This section will shed light on two frequent obstacles faced during the Western blotting process: non-specific binding and low sensitivity.
Non-Specific Binding
Non-specific binding represents a significant hurdle in Western blotting. This term refers to the unintended interactions between antibodies and proteins that are not the intended targets. The result? A blot that presents as a muddled mess of bands, where the real target's signal is obscured. This highlights the necessity for precision in experimental design.
The root causes of non-specific binding often lie in the choice of blocking agents or the incubation conditions. Using an inadequate blocking agent can allow antibodies to bind to unrelated proteins, which can lead to increased background signal and noise. Common blocking agents like Bovine Serum Albumin or non-fat dry milk, while effective, aren't one-size-fits-all solutions. It takes some experimentation to strike the right balance tailored to specific sample types.
Strategies to minimize non-specific binding include:
- Optimizing Blocking Conditions: Adjusting the concentration of blocking agents or extending the blocking time can enhance specificity.
- Dilution of Antibodies: Sometimes, a more diluted solution provides cleaner results, reducing non-specific interactions.
- Washing Steps: Increasing the duration and frequency of washing steps can significantly decrease background noise.
"The art of Western blotting lies in the details; small adjustments can yield significant improvements."
Low Sensitivity
Low sensitivity is another common challenge in Western blotting that can render your analysis insufficient. Sensitivity refers to the minimum amount of target protein that can be reliably detected in a sample. When sensitivity is low, even abundant proteins can go undetected, leading to false conclusions about expression levels.
Several factors can influence the sensitivity of the assay. The choice of antibody is paramount; primary antibodies must have a high affinity for their targets. A weak antibody may not bind well enough to the protein, resulting in diminished detection. Likewise, the detection method employed—be it chemiluminescence, fluorescence, or colorimetric—also plays a critical role in determining sensitivity outcomes.
To improve sensitivity, consider the following:
- Concentration Optimization: Experimenting with the concentrations of both the primary and secondary antibodies can lead to better signal detection.
- Utilizing Enhanced Detection Kits: These kits often come with optimized reagents designed to boost sensitivity.
- Reducing Sample Limitation: Ensuring enough sample is loaded into the gel can also help enhance the detection of low-abundance proteins.
Both non-specific binding and low sensitivity not only affect individual experiments but can also influence the overall credibility of research findings. Addressing these challenges requires patience, systematic experimentation, and a willingness to adapt protocols. The road might be bumpy, but with careful navigation, researchers can indeed arrive at robust and reliable results.
Best Practices
In the realm of Western blotting, adhering to best practices is not just a recommendation; it’s a necessity for obtaining reliable results. The significance of these practices cannot be overstated, as they influence the accuracy, reproducibility, and overall success of the experiment. Even small deviations from established protocols can lead to considerable discrepancies in the results. Therefore, a thorough understanding and implementation of these best practices play a pivotal role in enhancing the quality of research outcomes.
Optimizing Protocols
Optimizing protocols is the cornerstone of effective Western blotting. It involves fine-tuning various steps in the procedure to achieve maximum sensitivity and specificity. Factors such as antibody concentration, incubation time, and temperature can vastly impact the quality of the results.
- Antibody Selection: Choosing the right antibody is crucial. Consider factors like the antibody's affinity and specificity for the target protein. Using monoclonal antibodies can lead to more consistent results, whereas polyclonal antibodies may enhance detection sensitivity but possibly introduce background noise.
- Concentration Adjustment: It’s vital to optimize the antibody concentration. Too much can lead to non-specific binding, while too little may hinder the detection of low-abundance proteins. It’s wise to run a dilution series to find the sweet spot.
- Incubation Conditions: Incubation times and temperatures can be adjusted based on the specific reaction conditions. Some antibodies perform better at room temperature, while others may need to incubate in colder or warmer environments. Check manufacturer suggestions, but don’t hesitate to experiment based on past findings in your lab.
- Blocking Agents: The choice of blocking agent should align with the type of antibody used and the sample matrix. For example, bovine serum albumin is preferable for some applications, while non-fat dry milk may be better suited in others. Test different agents to determine the one that minimizes background while still allowing your target protein to be detected.
By adopting a tailored approach in optimizing each of these aspects, researchers can greatly improve the reliability of the Western blot assay.
Validation Techniques
Once protocols are optimized, validation techniques ensure that the results obtained are accurate and reproducible. These validation steps serve as checkpoints in the experimental process.
- Controls: Always include positive and negative controls. Positive controls confirm that the assay is working as intended, while negative controls help identify possible non-specific interactions or background signal.
- Replicates: Performing experiments in replicates is essential. This allows for statistical analysis that can discern true variation from experimental error.
- Cross-validation with Alternative Methods: Whenever possible, validating results obtained from Western blotting with complementary techniques, such as ELISA or mass spectrometry, adds an additional layer of confidence to your findings. This cross-validation ensures that any observed signals are indeed reflective of the target proteins and not artifacts of the procedure.
- Documentation: Keep precise records of all experimental conditions and outcomes. This transparency not only helps in troubleshooting issues but also aids others in replicating your work.
By rigorously applying these validation techniques, researchers can fortify the reliability of their results, thus contributing meaningfully to the field of molecular biology.
Quantification and Data Analysis
Quantification and data analysis represent critical components in the Western blotting process. Beyond simply detecting proteins, the quantification aspect allows researchers to draw significant correlations within their biological samples. Estimating the amounts of proteins not only elucidates biological pathways but also paves the way for further investigations, making this step vital in molecular biology assessments.
In the modern lab setting, the tools and techniques utilized for quantification have evolved. More researchers are taking advantage of sophisticated image analysis software, which automates many of the processes involving quantitative measurement while also reducing human error. The benefits here are twofold: precision increases, and researchers can focus their attention on interpreting data, rather than spending excessive time on calculations.
However, it’s essential to approach quantification with a discerning eye. Factors like sample preparation and the blocking steps can influence the final readings, making it necessary for scientists to be thoroughly aware of their methodologies. Disparities in protein loading or variations in transfer efficiency can skew results, leading to incorrect conclusions. Therefore, careful consideration of all variables involved in the analysis is crucial.
"Quantification in Western blotting isn't just about numbers; it's a narrative—the story behind the proteins. Understanding it gives depth to the scientific inquiry."
Image Analysis Software
Modern image analysis software plays an indelible role in how quantification is carried out in Western blotting. These programs are specifically designed to assess the intensity of protein bands on blots and translate them into quantifiable data. Here are a few key advantages of using such software:
- Accuracy: Automated software minimizes human bias and calculation errors that often accompany manual measurements.
- Time Efficiency: They allow labs to process data rapidly, providing quicker turnarounds in research developments.
- User-Friendly Interfaces: Most software comes with intuitive layouts that reduce the learning curve for new users.
Some popular software packages include ImageJ, gel analyzer tools, and proprietary solutions from companies like Bio-Rad. Utilizing these tools not just enhances your processing capabilities but also contributes to the overall credibility of your findings by ensuring rigorous data evaluation.
Interpreting Results
Interpreting the results from Western blots can feel like putting together a jigsaw puzzle. It’s not just about identifying if a band is present; it's about understanding its significance in the overarching biological context. Here are the primary considerations when interpreting these results:
- Normalization: It is imperative to normalize the data against a loading control to ensure that variations in protein loading don’t skew results. Common loading controls include housekeeping proteins like β-actin or GAPDH.
- Statistical Analysis: Employing proper statistical methods can help validate your findings. Performing statistical tests allows you to deduce if your results are statistically significant, strengthening your conclusions.
- Biological Replicates: Always interpret the data within the context of experiments involving multiple biological replicates to ensure the robustness of your conclusions.
- Correlation with Hypotheses: Finally, results should be discussed in light of the original research question or hypothesis. Does the intensity of the observed bands support or contradict your initial hypothesis?
Hence, effective and thoughtful interpretation could lead to breakthroughs or raise new questions, paving the path forward in molecular research.
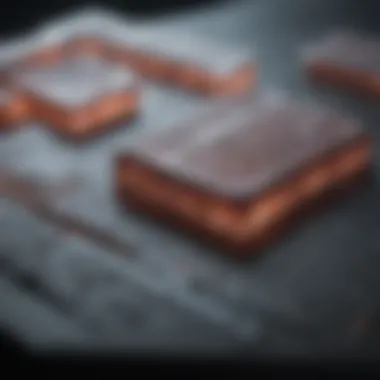
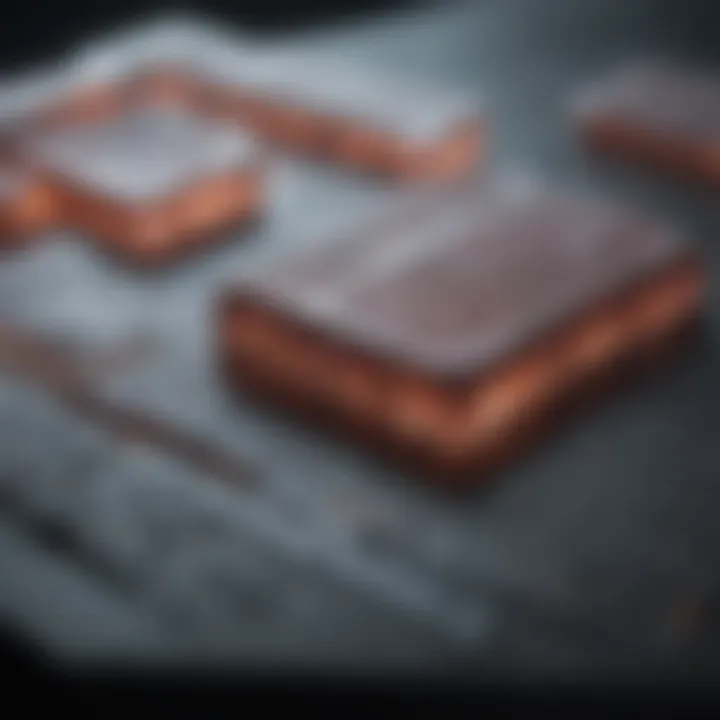
Applications of Western Blotting
The versatility of Western blotting extends far beyond basic protein detection; it serves as a cornerstone in both clinical diagnostics and research applications. In the realm of molecular biology, its applications hold significant value due to the precise information it provides regarding protein expression, modifications, and interactions. This section discusses the practical implications of Western blotting, reinforcing its essential role in advancing scientific inquiry and patient diagnosis.
Clinical Diagnostics
In the clinical setting, Western blotting finds itself amidst a host of diagnostic tools. Its most notable application is in the confirmation of specific diseases. For instance, when screening for HIV, the Western blot test is often used as a follow-up to ELISA tests. It allows for the identification of antibodies against viral proteins, providing a more reliable confirmation of infection. The specificity of Western blotting helps in materializing clear results which can direct treatment plans effectively.
Moreover, it’s also employed in diagnosing other conditions like Lyme disease or certain types of cancers. The technology's capacity to separate proteins effectively and identify them through specific antibodies grants clinicians a chance to make informed medical choices. However, reliance on this method requires robust sample handling and thoughtful interpretation of results, as false positives or negatives could lead to misdiagnosis. Thus, while Western blotting enhances diagnostic accuracy, it comes with its own share of challenges that must be understood well.
Research Applications
The domain of research greatly benefits from the application of Western blotting, revealing insights into cellular behavior and protein dynamics. It's a vital tool for exploring various biochemical pathways and assessing the effects of drugs on protein activity.
Protein Expression Studies
One of the primary research applications is in studying Protein Expression. By assessing the levels of specific proteins in different conditions or treatments, researchers can glean a richer understanding of biological processes.
For instance, differential expression analysis can be used to compare healthy tissues to diseased ones, allowing scientists to pinpoint biomarkers associated with diseases. This aspect of protein expression studies is crucial for exploratory research in oncology, as it aids in identifying potential therapeutic targets.
The key characteristic of protein expression studies is their ability to provide quantifiable data on protein levels. Researchers appreciate this benefit since it lays a solid foundation for hypotheses and subsequent experimental design. However, the downside can sometimes include limitations in detecting low-abundance proteins, which might lead to an incomplete picture.
Signal Transduction Analysis
Another compelling aspect of Western blotting is its effectiveness in Signal Transduction Analysis. This technique permits the study of protein modifications, such as phosphorylation, which are critical in signal transduction pathways. By determining which proteins are activated or inhibited in response to external signals, scientists can construct detailed maps of cellular communication.
Signal transduction studies significantly benefit from the precise quantification of phosphorylated versus non-phosphorylated proteins. This insight is vital in fields like neurobiotechnology and cellular biology, where understanding these pathways can lead to improved therapeutic strategies. Yet, it's important to highlight that alterations in protein localization and interplay can complicate interpretation, requiring careful experimental design and controls.
Summary
In summation, the applications of Western blotting resonate through both clinical diagnostics and research environments. Its relevance in medical diagnoses cannot be understated, while its ability to unravel the complexities of protein behavior in research further establishes its pivotal role in modern biology. As the field evolves, enhancing the efficiency and reliability of this technique will foster continued discoveries in both medicine and science.
Comparative Techniques
In the realm of protein analysis, understanding the comparative techniques available is pivotal for researchers seeking the most effective method for their specific applications. Two of the most common methods, ELISA and Western Blotting, serve distinct yet sometimes overlapping purposes in protein detection. Mass spectrometry further complements these methods by providing detailed information on protein composition and structure. Recognizing the strengths and limitations of each technique can help guide researchers in choosing the ideal approach to fulfill their analytical goals.
ELISA vs. Western Blotting
Enzyme-Linked Immunosorbent Assay (ELISA) and Western Blotting both serve the purpose of detecting proteins, but they do so in different ways. ELISA is typically known for its high throughput and quantitative nature, allowing researchers to analyze multiple samples simultaneously, which can be a massive advantage in clinical settings. It entails the immobilization of antigens on a solid surface, typically a microplate, followed by the incubation with antibodies, resulting in a colorimetric response that reflects protein concentration.
On the other hand, Western Blotting provides a more in-depth qualitative analysis. It separates proteins based on their molecular weight using gel electrophoresis and then transfers them onto a membrane where specific antibodies target the proteins of interest. This method offers greater specificity, revealing important information about protein size and modifications, but at the cost of being more labor-intensive and time-consuming.
Some key differences include:
- Sensitivity: Western Blotting may detect lower abundances of proteins than ELISA because of the amplification techniques that can be applied.
- Quantification: ELISA provides a straightforward quantification, while Western Blotting often requires image analysis and normalization.
- Automation: ELISA is typically easier to automate, which is beneficial in high-throughput environments.
- Complexity: Western Blotting involves multiple steps, making it more complex than the straightforward procedure of ELISA.
Understanding these distinctions can significantly influence the choice of techniques in research projects or clinical diagnostics. Researchers must weigh these factors against their specific objectives to determine the most suitable approach.
Mass Spectrometry Comparison
Mass spectrometry (MS) offers a complementary avenue in protein analysis, standing apart yet often enhancing the capabilities of ELISA and Western Blotting. Unlike the aforementioned techniques, which primarily focus on protein detection and quantification, MS provides insights into the mass-to-charge ratio of ions, yielding information on the size, composition, and structure of proteins.
One of the notable advantages of mass spectrometry is its ability to analyze complex mixtures without the need for prior separation, a noteworthy feature when dealing with biological samples where proteins may exist in many different forms. Mass spectrometry excels in identifying post-translational modifications, a crucial factor in understanding protein function and interaction in cellular processes.
However, while mass spectrometry is incredibly powerful, the upfront costs and the requirement for specialized training can render it less accessible than ELISA or Western Blotting for some laboratories. Furthermore, sample preparation for mass spectrometry can also be time-consuming and intricate, making it necessary to consider this when planning experiments.
Here’s a brief rundown of the unique aspects of mass spectrometry compared to ELISA and Western Blotting:
- Analytical Depth: MS provides more in-depth analysis compared to the information obtained from ELISA and Western Blotting.
- Complexity of Sample Preparation: The preparation for MS is often more complex.
- Speed: With modern advances, MS can sometimes yield results faster than Western Blotting, depending on the setup.
- Cost: Mass spectrometers are significantly more expensive than typical ELISA kits or Western Blotting setups.
In summary, while ELISA and Western Blotting remain staples for protein detection, mass spectrometry enhances the toolbox available for researchers, enabling a broader understanding of proteins and their roles in biological processes. Understanding how each technique functions and what unique information it can provide is essential for informed decision-making in the field of molecular biology.
"In molecular research, the right method can be like finding a needle in a haystack. Choose wisely!"
As the landscape of protein analysis evolves, it becomes imperative for researchers to stay informed about comparative techniques, ensuring they harness the right method to meet their study's specific needs.
Future Directions in Western Blotting
The realm of Western blotting has seen substantial evolution since its inception. As researchers tread further into the realms of molecular biology, the direction this technique takes becomes increasingly vital. Two key aspects stand out when pondering the future of Western blotting: technological advances and potential improvements in protocols. Both areas promise to not only enhance the effectiveness of this technique but also expand its applications, which ultimately can lead to breakthroughs in fields like diagnostics and therapeutic development.
Technological Advances
In the backdrop of rapid technological innovations, Western blotting is on the brink of transformation. The incorporation of novel technologies is setting the stage for a more efficient and precise analysis of proteins. One notable advance is the integration of microfluidics into blots. Microfluidics allows researchers to manipulate small volumes of fluids, making sample handling much more efficient. This could facilitate high-throughput analyses, significantly reducing time and resources.
Moreover, enhanced imaging technologies like digital imaging systems are ushering in a new era, enabling more accurate quantification and detection of proteins. Compared to traditional methods, digital imaging can minimize errors associated with manual interpretations. A growing interest in mass spectrometry, when used in conjunction with Western blots, holds promise too. This combination can yield deeper insights into protein characterization, providing valuable information about post-translational modifications that would otherwise be missed.
Another frontier worth noting is the rise of multiplexing techniques. Advanced multiplexing can simultaneously analyze multiple proteins in a single sample, allowing for a comprehensive profiling of protein expression levels. This not only saves on reagents but also improves the quality of data by reducing variability.
"Embracing technological advances in Western blotting means moving toward a future where precision and efficiency become standard rather than exceptions."
Potential Improvements in Protocols
While advancements in technology are exciting, the core protocols behind Western blotting need continuous refinement too. One area of improvement lies in the blocking step. Traditional blocking agents can sometimes lead to background noise that hampers the specificity of the results. Newer agents that are less complex could be developed, providing researchers with cleaner results and clearer insights.
Moreover, the incubation times and temperatures for antibodies can also be optimized. There's a growing recognition that adjusting these parameters can significantly impact signal intensity and background. For example, exploring the effects of longer incubation periods at lower temperatures might allow for a more sensitive detection while reducing background interference.
Additionally, as understanding of protein-protein interactions deepens, protocols could be refined to investigate more complex biological networks. Techniques integrating co-immunoprecipitation with Western blotting could be standardized, allowing researchers to not just study individual proteins, but to understand their roles within larger protein complexes.
A focus on automation could also streamline workflows. Automated systems for sample handling, gel loading, and even data analysis would mitigate human error, contributing to more reproducible results and less variance across experiments.
In summary, as we look toward the future of Western blotting, it's clear that a combination of cutting-edge technologies and refined protocols will lead to a more adaptable and powerful research tool. The ongoing refinement not only holds the promise for enhanced accuracy but also addresses challenges faced in current methodologies, paving the way for a richer understanding of protein biology.
The End
In the realm of molecular biology, the conclusion serves as a crucial junction where all threads of the narrative weave together, reinforcing the significance of the Western blotting technique. The insights gleaned throughout this article highlight not only the technical intricacies involved in each phase of the process but also the profound implications these methodologies hold for research and clinical applications.
Recap of Key Points
To summarize, here are the vital takeaways from our extensive examination:
- Introduction to Western Blotting: We explored its historical roots and current relevance as a robust method for protein analysis.
- Principle of Western Blotting: Understanding the fundamentals, particularly the roles of proteins and antibodies, is imperative to grasp how this technique operates effectively.
- Sample Preparation Techniques: Properly preparing samples is foundational for accurate results. We discussed various sample types and lysis methods.
- Gel Electrophoresis Process: This process is crucial for separating proteins based on molecular weight, with details on different gels and loading techniques.
- Detection Methods: Chemiluminescence, fluorescence, and colorimetric methods provide diverse options for visualizing proteins.
- Common Challenges: Identifying non-specific binding and sensitivity issues allows researchers to refine their protocols.
- Best Practices: Optimizing experiments and validation are essential for reliable outcomes.
- Quantification and Data Analysis: Image analysis software is vital in interpreting the results accurately.
- Applications of Western Blotting: The versatility of this technique in both clinical diagnostics and various research applications underscores its value.
- Comparative Techniques: Understanding the strengths and weaknesses of related methods like ELISA and mass spectrometry can guide effective research design.
- Future Directions: The necessity for innovation is clear, as we discussed technological advancements and potential improvements in protocols.
The Significance of Continued Research
The ongoing quest for knowledge in the field of Western blotting cannot be understated. Each discovery adds layers to our understanding of biochemical processes and enables us to confront health challenges head-on. As research evolves, new methodologies improve specificity and sensitivity, addressing current limitations.
More significantly, as new techniques emerge and older ones get refined, the implications for diagnostic practices and therapeutic approaches become evident. For scientists, this means staying ahead of the curve, ensuring they are equipped with the latest tools for analyzing proteins in their endeavors.
Continued exploration in this area has the power to unlock new pathways for understanding disease mechanisms and developing targeted treatments. As researchers delve deeper into protein dynamics, the scope of Western blotting will likely expand, leading to breakthroughs that are not only academically intriguing but also hold potential to change lives.