Gene Expression in Prokaryotes: Mechanisms and Regulation
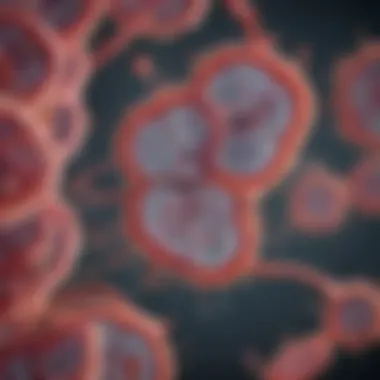
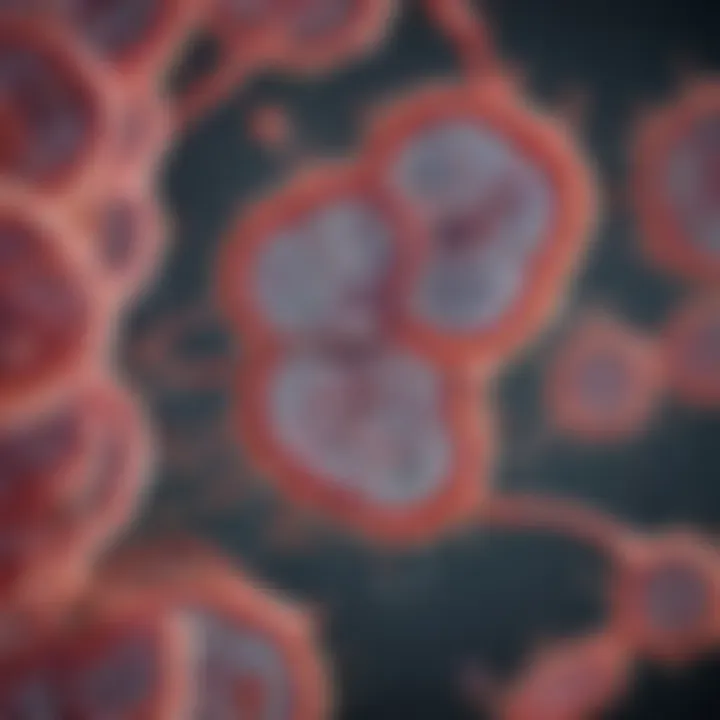
Intro
Gene expression in prokaryotes is a fundamental topic within molecular biology, pivotal for understanding how these organisms survive and thrive in diverse environments. Prokaryotes, which include bacteria and archaea, are characterized by their simplicity compared to eukaryotic counterparts. Yet, the mechanisms governing gene expression within prokaryotes reveal a complexity that is essential for regulating cellular activities.
This article explores two main processes: transcription and translation. In transcription, the DNA sequence of a gene is copied to produce RNA, which serves as a template for the synthesis of proteins through translation. Additionally, the regulation of these processes is critical. Regulatory elements, such as operons, play a significant role in ensuring that genes are expressed only when needed, responding to various environmental signals. With these considerations in mind, an in-depth examination of prokaryotic gene expression unveils the intricate dance of molecular interactions that sustain life.
Methodology
Understanding gene expression in prokaryotes involves a systematic study of existing literature and experimental techniques.
Study Design
The study design primarily focuses on observational methodologies, drawing from established research findings, laboratory practices, and theoretical frameworks. Various experiments have been conducted to elucidate the mechanisms of transcription and translation within prokaryotic cells.
A multitude of sources, including peer-reviewed articles, textbooks, and genetic databases, has been analyzed to compile a cohesive narrative regarding gene expression mechanisms. This literature provides a foundation for comprehending the processes at a molecular level.
Data Collection Techniques
Data collection primarily involves:
- Reviewing experimental results from primary research articles.
- Analyzing case studies of various prokaryotic organisms.
- Referencing databases like NCBI and UniProt for genetic information.
- Utilizing tools such as PCR (Polymerase Chain Reaction) and sequencing to explore gene regulation experimentally.
These data collection techniques ensure a comprehensive understanding of how prokaryotic cells manage gene expression.
Discussion
Interpretation of Results
The intricacies of gene expression in prokaryotes can substantially differ from those in eukaryotes. One significant finding is the organization of genes into operons. Operons allow for coordinated expression, providing efficient responses to environmental changes.
Another notable aspect is the role of transcription factors. These proteins regulate the initiation of transcription, tailoring the gene expression profile according to cellular demands. Prokaryotic cells can rapidly adjust their metabolic pathways in response to fluctuations in conditions, an adaptation often initiated via signal transduction pathways.
"In prokaryotes, a group of related genes can be expressed together, enhancing efficiency and speed of response to environmental changes."
Limitations of the Study
While this analysis provides valuable insights, there are limitations to consider. Many experimental studies focus on model organisms like Escherichia coli. This can restrict generalizability to other prokaryotic species, as metabolic and regulatory mechanisms can vary greatly across different genera. Additionally, environmental factors used in laboratory settings may not always mimic natural habitats accurately, leading to potential discrepancies in gene expression under real-world conditions.
Future Research Directions
Future research could explore several areas, including:
- The interaction between prokaryotic and eukaryotic gene regulation.
- The effects of synthetic biology applications on prokaryotic gene expression.
- The impact of climate change on gene regulation mechanisms in prokaryotic populations.
Such investigations will deepen our understanding of adaptation in prokaryotes, clarifying how these organisms respond to ever-changing environments.
Preamble to Prokaryotic Gene Expression
Prokaryotic gene expression plays a crucial role in understanding cellular function and adaptation to environmental changes. As prokaryotes are the most abundant life forms on Earth, their mechanisms of gene expression are fundamental to both ecology and biotechnology. Prokaryotic organisms, which include bacteria and archaea, exhibit unique gene expression pathways compared to eukaryotes. This article aims to elucidate these pathways, examining not only the core processes of transcription and translation but also the regulatory elements that shape gene expression in these simpler organisms.
Defining Gene Expression
Gene expression refers to the process through which information from a gene is utilized to synthesize functional gene products, most commonly proteins. In prokaryotes, the expression involves two main steps: transcription and translation. Transcription is the initial phase, where the DNA sequence of a gene is copied into messenger RNA (mRNA). Subsequently, during translation, the mRNA is used as a template for synthesizing proteins. This flow of information from DNA to RNA to protein is often termed the central dogma of molecular biology.
In prokaryotes, this process is notably efficient. Both transcription and translation can occur simultaneously in the cytoplasm, a feature that distinguishes prokaryotic cells from eukaryotic ones, where these processes are separated by cellular compartments.
The Significance of Gene Expression in Prokaryotes
Understanding gene expression in prokaryotes is vital for several reasons:
- Adaptability: Gene expression allows prokaryotes to respond swiftly to environmental changes. For instance, when nutrients are scarce, certain genes are activated to facilitate metabolic adjustments.
- Efficiency: The streamlined processes of transcription and translation enable prokaryotes to reproduce rapidly. This efficiency is essential for survival in competitive environments.
- Biotechnological Applications: Insights into prokaryotic gene expression have major implications in biotechnology. For example, Escherichia coli is a model organism in genetic engineering, making it important to understand its gene regulation mechanisms.
Overview of Prokaryotic Cell Structure
Understanding prokaryotic cell structure is crucial to dissecting the mechanisms governing gene expression in these organisms. Prokaryotic cells, being the simplest form of cellular life, provide a foundation for comprehending the efficiency and compactness of biological processes. The framework of these cells directly influences their gene expression pathways, making it essential to grasp their structure when analyzing how genes are regulated and expressed.
Basic Features of Prokaryotic Cells
Prokaryotic cells present several defining features:
- Nucleus: Unlike eukaryotic cells, prokaryotes lack a membrane-bound nucleus. Their genetic material is organized in a nucleoid, a region within the cell that contains circular DNA.
- Organelles: Prokaryotes do not possess membrane-bound organelles. This absence simplifies their cellular machinery yet emphasizes the efficiency of their functions.
- Size: Typically smaller than eukaryotic cells, prokaryotes range from 0.1 to 5.0 micrometers in diameter. This size efficiency aids rapid growth and reproduction.
- Cell Wall: Most prokaryotic cells have a rigid cell wall, primarily made of peptidoglycan. This structure provides protection and maintains cell shape.
- Plasmids: Many prokaryotes contain plasmids, which are small, circular DNA molecules distinct from chromosomal DNA. Plasmids often carry genes that confer advantageous traits, such as antibiotic resistance.
Prokaryotic cell structure is not only fundamental in maintaining cellular integrity but is also integral to the immediate access to genetic information for transcription and translation processes.
Comparison Between Prokaryotic and Eukaryotic Cells
Comparing prokaryotic and eukaryotic cells illuminates significant differences that impact gene expression:
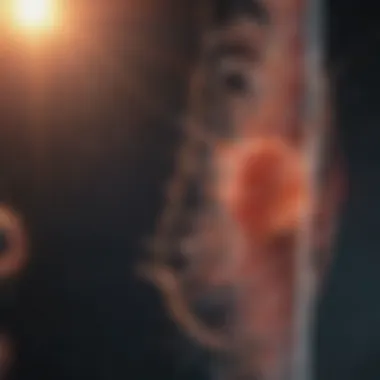
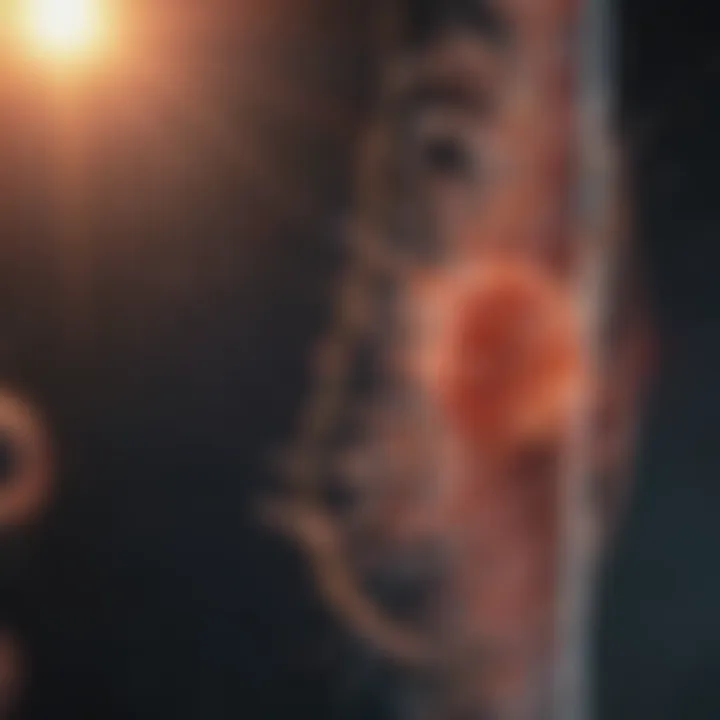
- Genomic Organization: Prokaryotic DNA is circular and free-floating, while eukaryotic cells have linear DNA encased within a nucleus.
- Gene Regulation: Prokaryotic gene expression takes place almost simultaneously at the transcription and translation levels in the cytoplasm, which stands in contrast to eukaryotic cells, where these processes are compartmentalized, involving additional steps such as mRNA splicing.
- Ribosome Size: Prokaryotic ribosomes (70S) are smaller than eukaryotic ones (80S), leading to differences in protein synthesis mechanisms.
The simplicity of prokaryotic cells results in faster responses to environmental changes, giving them a survival advantage in fluctuating conditions.
In summary, the distinction between prokaryotic and eukaryotic cells emphasizes the adaptive nature of prokaryotes. Their structural characteristics enable efficient gene expression and responsiveness to their environment. This knowledge lays the groundwork for deeper discussions on gene expression mechanisms in prokaryotes.
Cellular Machinery for Gene Expression
Gene expression in prokaryotes relies heavily on cellular machinery that is integral to the transcription and translation processes. This section explores the essential components and their roles in ensuring effective gene expression. Understanding this machinery sheds light on how bacteria and archaea manage to respond rapidly to changes in their environment, making it critical for both basic biological research and applied sciences.
Role of DNA in Prokaryotic Gene Expression
The information necessary for protein synthesis is encoded in the prokaryotic DNA. Unlike eukaryotic cells, prokaryotic organisms possess a single, circular chromosome, which is compact and organized differently.
The DNA in prokaryotes not only provides a template for transcription but also directly facilitates regulatory functions. The presence of promoter sequences and regulatory regions allows for the control of gene expression.
One of the key elements found in prokaryotic DNA is the operon, a cluster of genes under the control of a single promoter. The coordinated expression of these genes allows prokaryotes to efficiently respond to environmental changes. For example, when lactose is present, the lac operon is activated to produce enzymes necessary for its metabolism.
RNA Polymerase: The Key Enzyme
RNA polymerase is arguably the most critical enzyme in gene expression. In prokaryotes, a single type of RNA polymerase is responsible for the transcription of all types of RNA, including mRNA, tRNA, and rRNA.
This enzyme initiates the process by binding to the promoter of the gene, unwinding the DNA strands, and synthesizing a complementary RNA strand.
The process of initiation is highly regulated by various factors such as sigma factors, which assist in recognizing specific promoter sequences to ensure transcription begins at the right location. An efficient transcription process enhances the prokaryote's ability to quickly adapt to new situations.
Ribosomes in Protein Synthesis
Ribosomes play a vital role in the translation of mRNA into proteins. In prokaryotes, ribosomes are made of ribosomal RNA (rRNA) and proteins. Notably, they are significantly smaller than those in eukaryotic cells.
The ribosome reads the mRNA sequence in sets of three nucleotides, known as codons, each of which specifies a particular amino acid. The synthesis of proteins occurs in three main stages: initiation, elongation, and termination.
During initiation, the small ribosomal subunit binds to the mRNA and scans for the start codon. Once found, the large subunit joins, forming the complete ribosome ready for translation. Elongation involves the addition of amino acids, while termination occurs when a stop codon is reached, releasing the newly synthesized polypeptide.
The efficient function of ribosomes is essential not only for protein production but also for the overall growth and replication of prokaryotic cells, which underscores their importance in cellular machinery for gene expression.
Transcription in Prokaryotes
Transcription is an essential process in gene expression in prokaryotes, forming the first step in translating genetic information stored in DNA into functional proteins. This process is critical because it dictates how genetic information is used to produce proteins that carry out various cellular functions. Understanding the transcription mechanisms helps researchers understand various cellular processes and responses in prokaryotic organisms.
During transcription, the DNA molecule serves as a template for synthesizing messenger RNA (mRNA). This mRNA then acts as an intermediary, transporting the genetic code to ribosomes, where proteins are synthesized. The significance of transcription is not solely limited to protein production; it also plays roles in regulating gene expression and responding to environmental changes.
"The process of transcription ensures that cells can respond effectively to their environment by producing necessary proteins."
Understanding the steps in transcription, including initiation, elongation, and termination, is crucial for comprehending how prokaryotes manage gene expression.
Initiation of Transcription
Initiation is the starting point of the transcription process. This phase involves several key players, including RNA polymerase, a crucial enzyme that catalyzes the formation of RNA from a DNA template. Initiation occurs when RNA polymerase binds to a specific region known as the promoter, located upstream of the gene being expressed.
Key Elements of Initiation:
- RNA polymerase holoenzyme: The more complex form of RNA polymerase that includes a sigma factor, which assists in recognizing the promoter regions.
- Promoters: Specific DNA sequences that signal the start site for RNA synthesis. Each promoter has unique sequences, which can vary in strength and affinity for RNA polymerase.
Once RNA polymerase binds to the promoter, it unwinds the DNA helix, allowing access to the template strand of DNA. This preparation is critical for moving into the next phase of elongation.
Elongation: Synthesizing RNA
Once initiation is complete, the elongation phase begins. During elongation, RNA polymerase advances along the DNA template, synthesizing a complementary mRNA strand. The primary direction of RNA synthesis is 5’ to 3’, meaning nucleotides are added to the growing RNA strand at the 3’ end.
Considerations During Elongation:
- Nucleotide triphosphates: These are the building blocks of RNA, which include adenine (A), guanine (G), cytosine (C), and uracil (U). They are incorporated by RNA polymerase as it synthesizes RNA.
- Transcription bubble: The unwound portion of the DNA where RNA is synthesized, typically 17 base pairs in length, maintains the integrity of the DNA strands.
- Proofreading: RNA polymerase possesses the ability to proofread and correct mistakes, although this capability is not as extensive as that in DNA polymerases during DNA replication.
This phase continues until a termination signal is encountered, which leads to the final stage of transcription.
Termination of Transcription
Termination marks the end of the transcription process. In prokaryotes, two mechanisms drive transcription termination: intrinsic and rho-dependent termination.
- Intrinsic termination: This occurs when the RNA strand forms a hairpin loop structure due to complementary sequences within the RNA. The formation of this loop disrupts the interaction with RNA polymerase, leading to the release of the mRNA.
- Rho-dependent termination: This mechanism involves a protein called Rho, which binds to the mRNA and moves towards the RNA polymerase. When Rho catches up, it causes the dissociation of the RNA polymerase from the DNA, ending transcription.
Significance of Termination:
Proper termination is crucial to ensure that the mRNA is accurately synthesized and released, ready for translation. This is especially vital in prokaryotes, where translation can begin even before transcription is fully completed.
Organisms must regulate these processes to adapt to changing environments and maintain homeostasis. Overall, understanding transcription in prokaryotes provides crucial insights into how these organisms operate at a molecular level.
Translation in Prokaryotes
Translation is a critical process in prokaryotic gene expression, responsible for synthesizing proteins based on the instructions encoded in mRNA. This cellular activity forms the bridge between the genetic information stored in DNA and functional proteins, which serve as the workhorses of cellular activities. Understanding translation in prokaryotes is essential because it helps explain how organisms without a nucleus produce proteins rapidly, adapting efficiently to varying environmental conditions.
Initiation of Translation
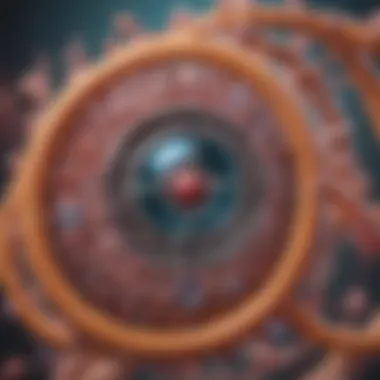
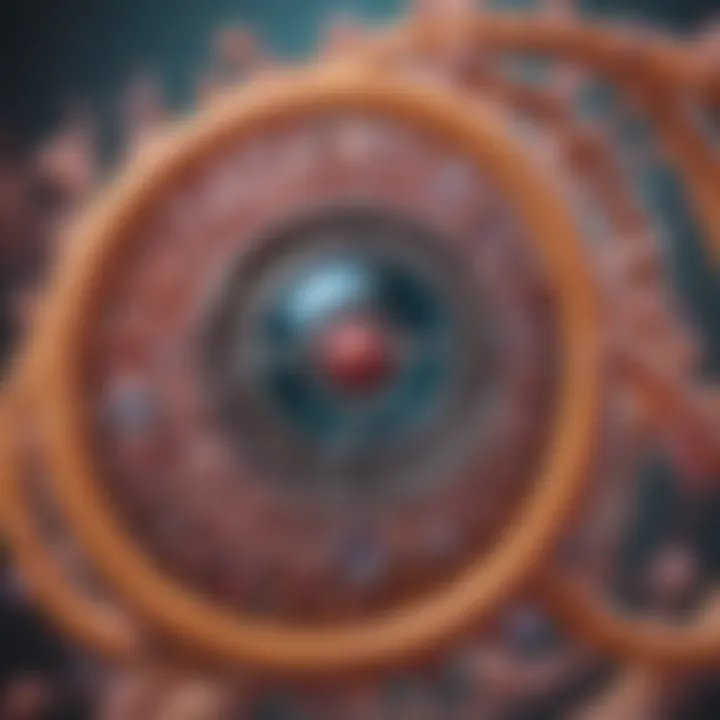
The initiation phase of translation marks the beginning of protein synthesis. It involves several key components: the ribosome, mRNA, and transfer RNAs (tRNAs). First, the small subunit of the ribosome binds to the mRNA molecule. This complex scans the mRNA to find the start codon, typically August (AUG), which signifies the place where translation begins. The initiator tRNA, carrying methionine, recognizes this AUG codon and pairs with it. Subsequently, the large ribosomal subunit joins this assembly, forming a complete ribosome ready for protein synthesis.
Elongation in Protein Formation
Once initiation is successfully completed, elongation can commence. This phase is characterized by the sequential addition of amino acids to the growing polypeptide chain. tRNAs, which transport specific amino acids, enter the ribosome at the A site, pairing with codons on the mRNA. The ribosome facilitates the formation of peptide bonds between adjacent amino acids, triggering a chain reaction as the ribosome moves along the mRNA template. Each cycle of elongation adds one amino acid to the chain, continuing until a stop codon is reached.
Termination of Translation
Termination occurs when the ribosome encounters a stop codon in the mRNA sequence. This codon does not correspond to any amino acid. Instead, it attracts release factors that prompt the ribosome to disassemble, releasing the newly synthesized polypeptide. The release factors cause the completed protein to detach from the tRNA and the ribosomal complex. As a result, the ribosome dissociates into its subunits, becoming available for the next round of translation.
Translation in prokaryotes is a swift and efficient process, allowing rapid response to environmental changes. This efficiency is crucial for survival in fluctuating conditions.
Regulatory Mechanisms in Gene Expression
Gene expression is not merely a linear process of transcription and translation; it is intricately regulated at multiple levels in prokaryotes. The mechanisms that govern this regulation are crucial for cellular function and adaptability. A deep understanding of regulatory mechanisms highlights how prokaryotic cells respond to environmental changes, ensuring the expression of genes required for survival, replication, and function.
At the core of gene regulation in prokaryotes are operons, which serve as the fundamental units. These operons enable coordination in the transcription of genes that share a common function, allowing prokaryotes to efficiently manage their metabolic activities. The regulatory elements associated with operons modulate gene expression in response to varying conditions, which is vital for adaptation.
Understanding the factors influencing gene expression is equally important. These factors can be internal, such as genetic mutations or feedback mechanisms, or external, like availability of nutrients or stressors in the environment. This versatility in regulation ensures that prokaryotes can thrive in diverse and often challenging environments.
Operons: The Fundamental Units
Operons are clusters of genes that are regulated together, and they express proteins with related functions. A well-known operon is the lac operon, which manages the metabolism of lactose in Escherichia coli. The operon is composed of structural genes, a promoter, and an operator.
Key features of operons include:
- Promoter Region: This region binds RNA polymerase, initiating transcription.
- Operator: A segment that can block RNA polymerase if a repressor protein is bound, controlling transcription.
- Structural Genes: These genes encode proteins with a common function, often implicated in metabolic pathways.
The elegance of operons allows simultaneous regulation of multiple genes, enhancing metabolic efficiency. This organization minimizes energy expenditure and coordinates metabolic responses effectively.
Factors Influencing Gene Expression
Several factors critically shape gene expression in prokaryotes. These can broadly be categorized into two groups: genetic regulatory elements and environmental conditions.
- Genetic Regulatory Elements:
- Environmental Conditions:
- Repressors: Proteins that bind to operators to inhibit transcription.
- Activators: Proteins that enhance transcription by facilitating the binding of RNA polymerase to the promoter.
- Enhancer Elements: These regions aid in stabilizing transcription factors that promote gene expression.
- Nutrient Availability: Lack or surplus of certain nutrients can trigger or inhibit gene expression.
- Stress Factors: Changes in temperature, pH, or osmotic pressure can lead to the expression of stress response genes.
- Quorum Sensing: This process allows bacteria to assess their population density and adjust gene expression accordingly, often influencing virulence factors.
The interplay of these factors forms a complex network that ultimately ensures the proper expression of genes. As research continues, the ongoing discovery regarding these mechanisms offers insights into both fundamental biology and potential biotechnological applications.
Environmental Influence on Gene Expression
Understanding how environmental factors influence gene expression is crucial for comprehending the flexibility and adaptability of prokaryotic organisms. Prokaryotes, including bacteria, can rapidly respond to changes in their surroundings by altering their gene expression profiles. This ability enhances survival under fluctuating conditions. For example, changes in nutrient availability can prompt metabolic shifts through the regulation of specific operons. Thus, the regulation of gene expression in response to environmental changes is essential for their growth and reproduction.
Responding to Environmental Changes
Prokaryotic organisms possess various mechanisms to sense and respond to environmental changes. When conditions change, these microorganisms can modulate gene expression swiftly. For instance, a sudden increase in temperature may trigger heat shock proteins, which help in protecting cellular integrity. Notably, prokaryotes utilize signal transduction pathways to relay information from the environment to gene expression machinery.
Key points regarding this response include:
- Signal Detection: Prokaryotes have receptors that detect changes in the external environment, feeding this information into the signaling pathways.
- Regulatory Proteins: These proteins can activate or repress target genes based on conditions. They act as molecular switches.
- Metabolic Adaptation: When faced with low oxygen concentrations, bacteria can deactivate aerobic respiration genes, switching to anaerobic pathways instead.
This ability to adjust gene expression according to environmental variables ensures that prokaryotes can survive and thrive in diverse habitats, from extreme heat to deep-sea environments.
Quorum Sensing in Prokaryotes
Quorum sensing is a sophisticated communication method employed by prokaryotes to detect cell density through the release and detection of signaling molecules known as autoinducers. This system enables bacteria to coordinate group behaviors, such as biofilm formation, virulence factor production, or bioluminescence, depending on the population density.
Key aspects of quorum sensing include:
- Autoinducers: These signaling molecules evolve among the bacteria, increasing in concentration with cell density. When a threshold concentration is reached, they trigger an array of genetic responses.
- Coordinated Behavior: By sensing others in their vicinity, bacteria can act in unison, which is more effective in attacks, such as during infection.
- Variations among Species: Different species utilize distinct signaling molecules tailored to their specific environmental contexts.
"Quorum sensing exemplifies the interconnectedness of microbial communities, underscoring the importance of gene expression regulation in collective behavior."
In summary, environmental conditions play an instrumental role in the regulation of gene expression in prokaryotes. Their ability to react to changes in the environment and communicate through systems like quorum sensing showcases their evolutionary adaptability.
The Role of Gene Regulation in Adaptation
Gene regulation is fundamental for prokaryotes as it directly impacts their ability to adapt to changing environmental conditions. Prokaryotic organisms, typically single-celled bacteria, face constant fluctuations in their surroundings, from nutrient availability to temperature variations. The ability to swiftly regulate gene expression allows these organisms to survive and thrive in diverse environments.
When prokaryotes experience new stresses, such as limited nutrients or toxic substances, they must adjust their metabolic pathways accordingly. Gene regulation serves as an adaptive mechanism, enabling cells to activate or deactivate specific genes based on external cues. This ensures efficient energy use and survival during unfavorable conditions.
The significance of understanding gene regulation cannot be overstated. It elucidates how prokaryotes have evolved strategies to maintain homeostasis. The adaptation mechanisms employed by these microorganisms can provide insights into evolutionary biology and the resilience of life forms.
Adaptation Mechanisms
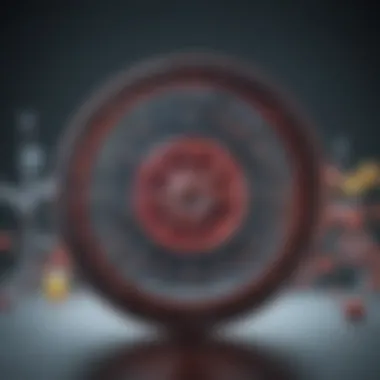
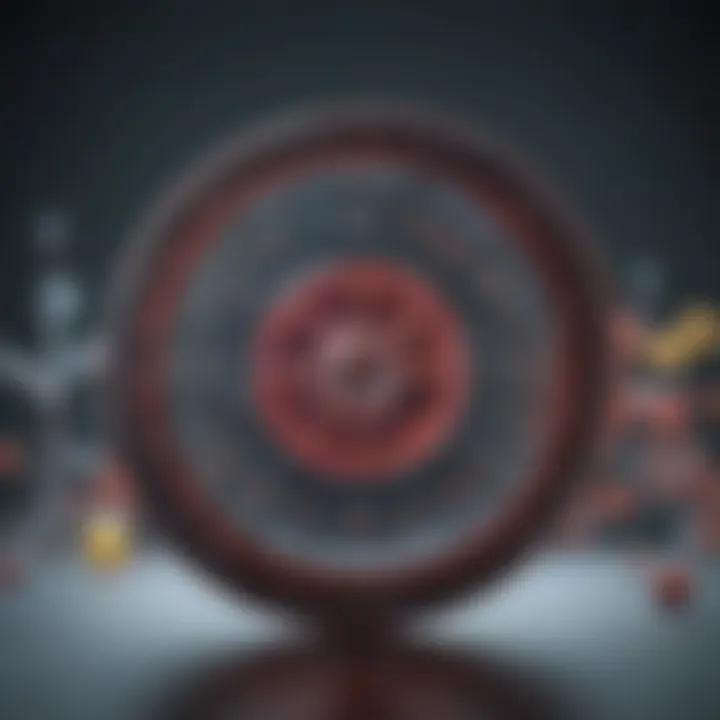
Prokaryotes employ several mechanisms for adaptation through gene regulation. Key among these is the use of operons, which allow bacteria to coordinate the expression of multiple genes that function together in a pathway. For example, the lac operon in Escherichia coli regulates the metabolism of lactose. When lactose is present, specific genes are expressed to utilize this sugar effectively.
Other adaptation mechanisms include:
- Transcriptional Regulation: Proteins known as transcription factors bind to DNA at specific sites, activating or repressing the transcription of target genes. This fast response is crucial in fluctuating environments.
- Post-Transcriptional Modifications: The stability of RNA molecules can influence how much protein is ultimately produced. Bacteria can modify RNA molecules to respond to immediate environmental conditions.
- Signal Transduction Pathways: These pathways allow cells to sense environmental changes, triggering cascades that ultimately lead to gene expression changes. This is essential in processes like quorum sensing, where bacteria communicate and synchronize behavior based on population density.
These mechanisms enable rapid responses and finely tuned control over gene expression, ensuring prokaryotes can adjust to their surroundings effectively.
Importance of Regulating Gene Expression
The importance of regulating gene expression in prokaryotes extends beyond mere survival; it is pivotal for complex behaviors and ecological interactions. For instance, by regulating genes responsible for virulence, pathogenic bacteria can enhance their survival in host environments, manipulating their host's immune response. This aspect highlights how gene regulation contributes to a bacterium's overall fitness and evolutionary success.
Moreover, the study of gene expression regulation in prokaryotes has broader implications for biotechnology and medicine. Advances in genetic engineering, which capitalize on our understanding of these regulatory mechanisms, open pathways for developing new antibiotics and treatments for diseases. The insights gained from investigating gene regulation in prokaryotes may also influence synthetic biology, where engineered bacteria could perform specified tasks like bioremediation or biofuel production efficiently.
Experimental Techniques in Studying Gene Expression
Understanding how gene expression operates is critical for advancing science. Experimental techniques that investigate these processes provide insight into cellular functions. This section covers methods essential for studying prokaryotic gene expression, focusing on the importance of accurate measurement and analysis.
Studying gene expression requires careful and precise methods. Each technique has its own benefits and limitations. Accurate results can help in understanding gene regulation in prokaryotes. Research on these techniques can lead to improved applications in biotechnology and medicine.
Techniques for Analyzing Transcription
Analyzing transcription in prokaryotes is a fundamental step in studying gene expression. Several key techniques are commonly used:
- RT-PCR (Reverse Transcription Polymerase Chain Reaction): This technique converts RNA into cDNA, allowing researchers to quantify mRNA levels. It provides insights into gene expression patterns, which can change under various conditions.
- Northern Blotting: This method detects specific RNA molecules using gel electrophoresis followed by membrane transfer. It allows for the analysis of mRNA size and expression levels. This can give an understanding of which genes are transcribed and under what conditions.
- RNA Sequencing (RNA-Seq): This modern method provides a comprehensive view of the transcriptome. It allows researchers to analyze all RNA species present in a sample. This high-throughput technique gives a full picture of gene expression and is powerful for comparing different conditions or treatments.
These techniques help elucidate how genes are expressed, revealing their roles and how they adapt to the environment.
Methods for Examining Translation Processes
Translation is the next step in gene expression. There are methods to study how proteins are synthesized in prokaryotic cells:
- Polysome Profiling: This technique separates ribosomes engaged in translation. It allows researchers to analyze mRNA associated with multiple ribosomes. This helps determine how efficiently a transcript is being translated into protein.
- Western Blotting: This is used to detect and quantify specific proteins in a sample. By measuring the presence and abundance of proteins, researchers can infer translational activity and how it correlates with transcription.
- Fluorescence Tagging: This modern approach uses fluorescent proteins tagged to specific proteins of interest. It provides insights into protein localization and dynamics within living cells.
These methods are critical for understanding how prokaryotic cells respond at a translational level to various stimuli or stress conditions.
To sum up, experimental techniques in gene expression are vital for advancing our understanding of molecular biology.
The insights gained from these methods can lead to groundbreaking discovery in genetics and deeper understanding of cellular machinery.
Current Research Trends in Prokaryotic Gene Expression
Research in prokaryotic gene expression is a vibrant field that continues to evolve. Understanding how these microorganisms regulate their genes is fundamental to biotechnology, medicine, and environmental science. Current trends emphasize integrating advanced techniques, like CRISPR-Cas systems, in gene regulation studies. These trends not only enhance scientific knowledge but also enable innovations in gene editing and microbial engineering.
Novel Discoveries and Breakthroughs
In recent years, several notable discoveries have emerged in the study of prokaryotic gene expression. One significant breakthrough is the elucidation of non-coding RNAs (ncRNAs) roles in gene regulation. ncRNAs have been found to help fine-tune the expression of genes, influencing cellular responses to stress and other environmental factors. Another area of discovery includes the mechanisms of transcription factors interacting with regulatory sequences. Studies using Escherichia coli have shown how these factors manage gene activation and repression under varying conditions.
Furthermore, advancements in single-cell transcriptomics provide insights into gene expression variability among individual bacterial cells. This technique helps scientists observe how different cells within a population can express the same genes differently, leading to a deeper understanding of cellular function and adaptability.
"Understanding gene expression in real-time offers unprecedented insights into bacterial behavior and evolution."
Future Directions in Research
The future of research into prokaryotic gene expression is poised to be equally exciting. One promising direction involves the application of synthetic biology to construct artificial gene circuits. These circuits can mimic natural gene regulation processes or respond predictably to environmental changes. This approach holds massive potential for developing bio-based applications, such as bioremediation and sustainable manufacturing practices.
Another area forecast for growth is the exploration of interspecies relationships, particularly how gene expression is influenced by community dynamics in microbiomes. Understanding how gene regulation varies in different ecological contexts could lead to new strategies for managing bacterial communities in health and environmental settings.
Moreover, the use of machine learning and artificial intelligence should not be underestimated. By applying these technologies to large datasets, researchers can uncover patterns in gene expression that may not be discernible through traditional methods.
Culmination
Understanding gene expression in prokaryotes is critical for both fundamental biology and applied research. This article synthesized core mechanisms, such as transcription and translation, highlighting their unique processes compared to eukaryotic gene expression. By delving into regulatory elements, both internal and environmental influences, we can appreciate the sophisticated means by which prokaryotes adapt and thrive in various conditions.
The importance of recognizing operons as essential units in prokaryotic gene expression cannot be overstated. The coordination of multiple genes under a single regulatory mechanism illustrates the efficiency of prokaryotic systems. This coordination is vital for survival, allowing for rapid responses to environmental changes.
Through this exploration, we not only reaffirmed the basic principles governing gene expression but also underscored the significance of regulatory mechanisms in maintaining homeostasis and facilitating adaptation. The themes of adaptability and functional regulation are critical concepts to grasp, especially in the context of evolving bacterial populations.
"The capacity of prokaryotic organisms to fine-tune gene expression is a significant factor in their survival and evolutionary success."
Recap of Gene Expression Principles
In summary, gene expression in prokaryotes operates through well-defined mechanisms and regulatory systems. Key principles include:
- Transcription and Translation: These processes occur simultaneously in prokaryotes, allowing for rapid protein synthesis.
- Operons: Groups of genes are regulated together, often responding to environmental signals.
- RNA Polymerase: The critical enzyme responsible for synthesizing RNA strands from DNA templates.
- Ribosomes: They facilitate the translation of mRNA into proteins, essential for cellular function.
The interplay between these elements forms a robust network that enables prokaryotic organisms to adapt quickly to their surroundings, reinforcing their ecological niche.
Implications for Future Research
The study of prokaryotic gene expression holds various implications for future research directions. Understanding mechanisms at play opens new avenues in:
- Synthetic Biology: Designers of synthetic circuits can leverage knowledge of prokaryotic systems to create more efficient biological systems.
- Medical Research: Insights into bacterial gene regulation can inform antibiotic development strategies targeting specific regulatory functions.
- Environmental Science: Examining how prokaryotes respond to environmental stress, like temperature changes or nutrient availability, can help develop bioremediation strategies.
As research expands in these domains, it will be crucial to continue exploring the complexities of gene expression in prokaryotes, not only to enhance our technological capabilities but also to address global challenges in health and environmental sustainability.