Exploring the Intricacies of Microscale: An In-depth Examination
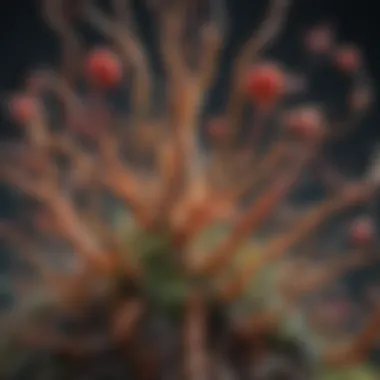
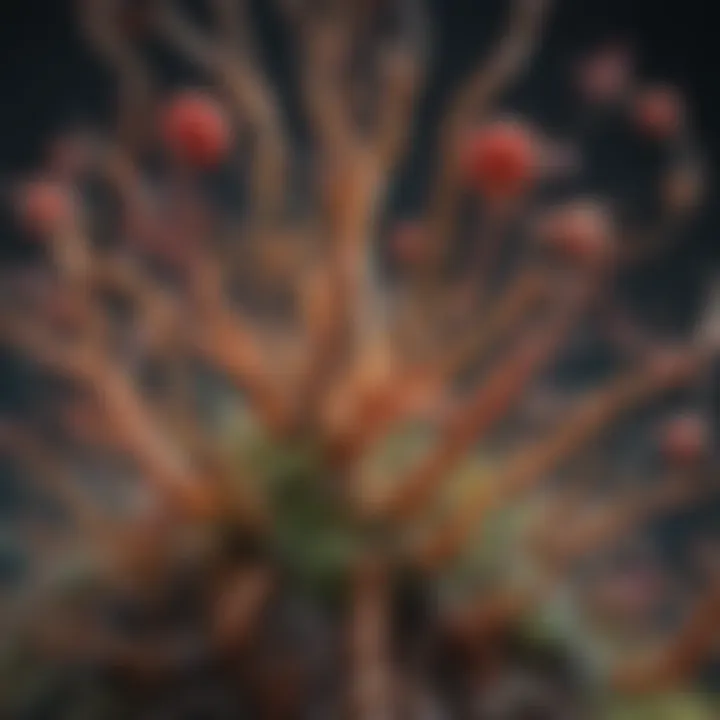
Intro
Microscale phenomena have garnered substantial attention across various scientific disciplines. Understanding this concept is not just about dissecting tiny structures or particles; it is about the nuanced implications these scales have in advancing research and technology. In biology, physics, chemistry, and earth sciences, microscale phenomena demonstrate unique behaviors that differ significantly from macroscopic observations. The relevance of the microscale lies in its capabilities to influence innovations in technology, medicine, and environmental science. This article offers a thorough overview of the methodologies employed in microscale research, alongside a discussion about findings, challenges, and future research directions in this pivotalarea of study.
Methodology
The study of microscale phenomena requires a systematic approach to understand the complexity involved effectively. Below are key aspects of the methodology adopted in these examinations.
Study Design
A well-defined study design helps to structure the investigation regarding microscale phenomena. It may involve:
- Experimental setups that utilize controlled environments to observe behaviors at the microscale.
- Quantitative analysis to measure variables accurately.
- Comparative studies that assess microscale phenomena in various contexts, highlighting differences and similarities.
Data Collection Techniques
Data collection in microscale studies is crucial for deriving valid conclusions. Common techniques include:
- Microscopy techniques, such as scanning electron microscopy (SEM) or atomic force microscopy (AFM), which provide high-resolution images of microscopic structures.
- Spectroscopic methods, including Fourier-transform infrared spectroscopy (FTIR), to analyze chemical properties at the microscale.
- Nanotechnology-based instruments like nanomanipulators, which facilitate the precise handling of nanoscale materials.
Discussion
Following the presentation of the methods, the findings from microscale studies warrant careful interpretation.
Interpretation of Results
The interpretation revolves around understanding how microscale phenomena contribute to broader scientific questions. For instance, the behavior of microorganisms in varying environments reveals insights regarding ecosystem dynamics and health implications.
Limitations of the Study
Despite advancements in microscale research, certain limitations persist.
- Technological constraints may hinder the resolution or accuracy of measurements.
- Sample variability can affect reproducibility of results.
- Environmental factors that are hard to control can introduce variability in findings.
Future Research Directions
Future research in the microscale domain promises to unveil new frontiers. Areas ripe for exploration include:
- Interdisciplinary collaborations to tackle complex problems involving multiple scientific fields.
- Development of novel materials at the nanoscale, with applications in medicine and electronics.
- Enhanced computational models to predict behaviors of microscopic systems under varied conditions.
The microscale realm is not just a field of study; it is a critical aspect of modern science that shapes our understanding of nature.
Through rigorous methodologies and insightful discussion, researchers can continue to illuminate the intricacies of the microscale, contributing to technological advancements and deeper comprehension of fundamental science.
Understanding Microscale in Science
The study of microscale is increasingly relevant in various scientific fields. It allows researchers to explore phenomena that occur at minuscule dimensions, often leading to new insights and advancements. Understanding microscale serves not only to deepen scientific knowledge but also to bridge gaps across disciplines such as biology, chemistry, physics, and earth sciences. By examining processes on this scale, we can uncover underlying mechanisms that often go unnoticed in larger systems. This overview outlines key aspects, benefits, and considerations of microscale research.
Defining Microscale
Microscale refers to dimensions that can range from a few micrometers to nanometers. This scale is critical for understanding biological systems like cells and microorganisms, as well as chemical reactions influenced by particle size. In simple terms, microscale encompasses the tiny details that make a big difference in various scientific phenomena. Researchers utilize precise tools and methods to manipulate and observe matter at this scale, enhancing our ability to interrogate the fundamental principles of science. This definition serves as a foundation for the discussion of microscale's broader implications in various fields.
Historical Context
Historically, the study of microscale phenomena began with early microscopy, which allowed scientists to observe cells and other minuscule structures for the first time. This field has advanced significantly over centuries, evolving from simple light microscopes to intricate electron microscopy and scanning probes. With these innovations, understanding microscale interactions has progressed rapidly. For instance, in the 19th century, the identification of cells as the basic unit of life opened doors to modern biology. In chemistry, insights gained from studying reactions at the molecular and atomic levels have transformed our understanding of materials and compounds. Recognizing these historical milestones helps contextualize the advancement of microscale research today.
Current Importance
In contemporary science, the study of microscale is vital for several reasons. First, it facilitates the development of new technologies like nanotechnology, which has applications in medicine, electronics, and materials science. Understanding microscale interactions leads to innovations such as targeted drug delivery systems and advanced materials with unique properties. Additionally, research at this scale contributes to addressing global challenges, such as environmental sustainability and health care. The relevance of microscale cannot be understated; it acts as a crucial link that connects numerous scientific disciplines and fosters interdisciplinary collaboration.
The significance of microscale research extends beyond scientific curiosity. It plays a crucial role in advancing technology and improving quality of life through innovative solutions.
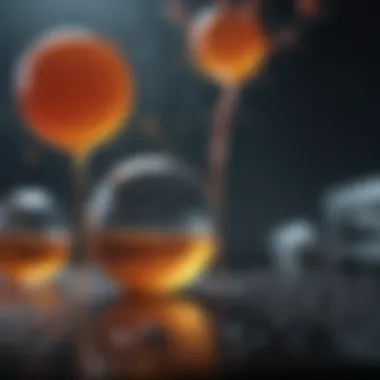
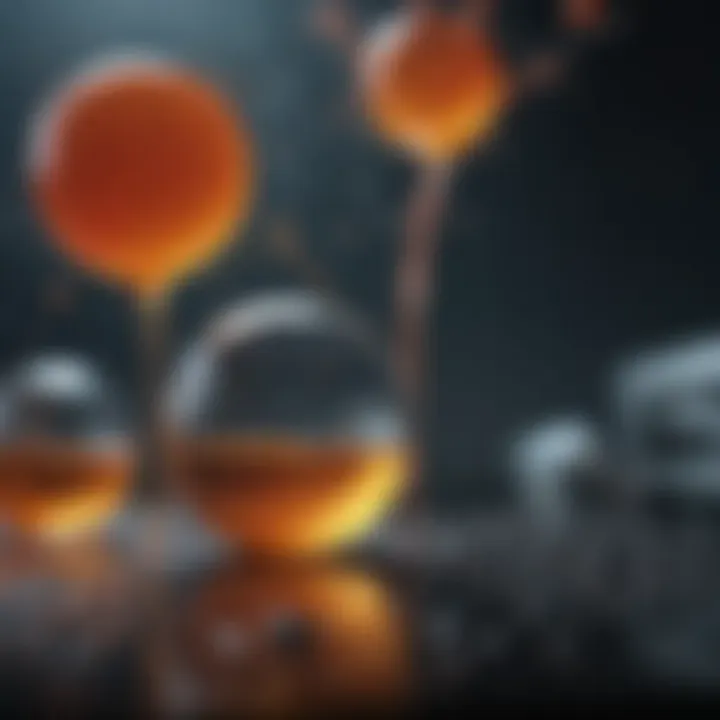
As new methodologies emerge, continued exploration at the microscale will likely yield further breakthroughs that may alter our understanding of various scientific disciplines.
Microscale in Biology
Microscale in biology plays a vital role in understanding the complex interactions and mechanisms that govern life. This domain focuses on the minute structures and processes that, while often invisible to the naked eye, are essential for the functioning of biological systems. By exploring cellular elements, microbial interactions, and research techniques, we can grasp the vast significance of microscale phenomena in biology. These aspects not only advance scientific knowledge but also provide insights into health, disease, and ecological balance.
Cellular Structures
At the core of microscale biology are cellular structures. Cells are the fundamental units of life. They comprise various organelles, each performing unique functions vital for the cell’s overall health. For instance, mitochondria are known as the powerhouses of the cell because they produce ATP, the energy currency of the cell.
Understanding the organization and function of these cellular components is crucial. Researchers can use techniques like fluorescence microscopy to visualize proteins within the cell. This method enables the observation of cellular processes in real-time, revealing how organelles interact and adapt to changing conditions. Such studies help in investigating not only normal cellular functions but also identifying the alterations in cancerous cells or during infections.
Microbial Interactions
Microbial interactions represent another important aspect of microscale biology. The relationship between microorganisms and their environments is complex. Microbes play a vital role in nutrient cycling, decomposition, and even human health. For example, the human gut microbiome consists of trillions of bacteria that assist in digestion and influence immune responses.
Research in this area often focuses on the dynamics between species. This can involve studying how different microbes compete or cooperate in various environments, from soil ecosystems to the human body. By understanding these interactions, scientists can develop strategies to combat pathogenic microbes or enhance beneficial ones. This exploration highlights the significance of microbial communities in maintaining ecological balance and human health.
Microscale Research Techniques
Microscale research techniques are essential for investigating the previously mentioned components of biology. These methods have evolved significantly, offering increasingly precise tools for scientists. Techniques such as microfluidics take advantage of the small scale to manipulate fluids in channels thinner than a human hair.
This approach allows for the study of biological samples with minute quantities, significantly reducing resource use while increasing efficiency. Also, techniques like CRISPR for gene editing enable researchers to modify genetic materials at the microscale, opening new avenues for genetic research and therapy.
To summarize, microscale innovations in research are expanding our understanding and capabilities. Such advancements promise better solutions in fields like medicine, agriculture, and environmental science.
The microscale world is teeming with interactions and mechanisms that form the foundation of life itself. Every detail contributes to the bigger picture of biological systems and their functions.
In essence, understanding microscale in biology provides substantial insights while also yielding practical implications for many scientific disciplines.
Microscale in Chemistry
The realm of microscale chemistry holds considerable importance in advancing our understanding of chemical processes at a minute scale. This area focuses on phenomena that occur at the nanoscale and microscale levels, which significantly differ in behavior compared to larger scales. Not only does this field provide insights into molecular interactions, but it also enhances the efficiency of chemical reactions, incorporating sustainable practices and innovative technologies.
As we delve into microscale chemistry, we uncover various elements that contribute to its richness and depth. The integration of microscale techniques leads to higher precision in experiments, minimized reactant use, and a more manageable analysis of chemical behavior.
Nanoscale Materials and Their Properties
Nanoscale materials, often defined as those having dimensions between 1 to 100 nanometers, exhibit unique properties that differ fundamentally from their bulk counterparts. These differences arise due to a higher surface area-to-volume ratio and quantum effects that become pronounced at such small scales. Common examples include gold nanoparticles and carbon nanotubes.
The unique characteristics of these materials lead to extraordinary applications, particularly in electronics, medicine, and environmental science. For instance, gold nanoparticles can be used in targeted drug delivery systems, while carbon nanotubes offer robust alternatives in constructing lightweight and high-strength materials.
Key Properties of Nanoscale Materials:
- Increased reactivity
- Enhanced strength without added weight
- Size-dependent optical properties
- Greater electrical conductivity in specific instances
Exploring nanoscale materials reveals both the potential and challenges of utilizing them effectively in real-world applications. Understanding these properties is paramount for advancing research efforts in various fields.
Chemical Reactions on a Microscale
Exploring chemical reactions at the microscale provides interesting insights into reaction mechanisms and dynamics. Microscale environments create conditions under which reactions can behave differently compared to larger volumes. This change in scale often enhances reaction rates and efficiency, enabling rapid experimentation and analysis.
Techniques like microfluidics enable the precise manipulation of small volumes of liquids. For example, a device may channel microdrops of reactants that induce a reaction in a controlled manner. This scaling down minimizes waste and can lead to significant reductions in cost and time.
In summary, advantages of microscale reactions include:
- Increased control over reaction conditions
- Enhanced safety due to smaller volumes
- Better scalability for industrial applications
The study of microscale chemical reactions allows researchers to derive fundamental principles that can inform larger-scale processes.
Applications of Microscale Chemistry

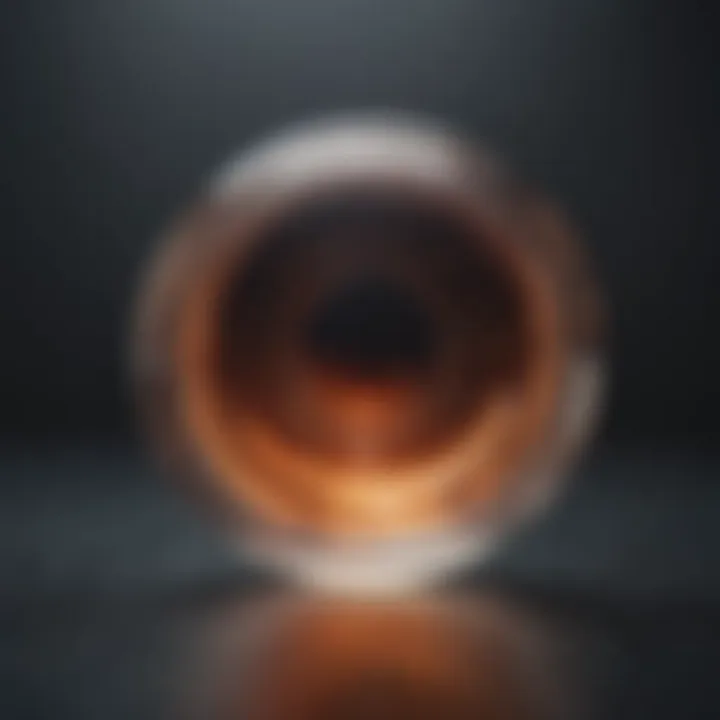
The applications of microscale chemistry are diverse and impactful. In pharmaceuticals, for instance, microscale techniques are used for drug formulation and analysis, allowing for the development of personalized medicine. These methods ensure that the right dosage is delivered effectively to patients, particularly those who may require tailored treatments based on their specific conditions.
In environmental science, microscale chemistry contributes to understanding pollutions and developing remediation strategies. Researchers can analyze subtle changes in soil or water composition at a microscale, providing crucial data for assessing environmental health.
Additional Applications include:
- Development of sensors for detecting chemical presence at low concentrations
- Synthesis of nanomaterials for various industrial applications
- Improvement in energy storage technologies, such as batteries and supercapacitors
By harnessing the power of microscale chemistry, industries can achieve innovative solutions that address contemporary challenges. The benefits span across various domains, making it a cornerstone of modern scientific inquiry.
Understanding the intricacies of microscale chemistry not only enhances scientific knowledge; it also drives technological innovation and sustainability in multiple sectors.
Microscale in Physics
Microscale phenomena in physics provide critical insights into the fundamental aspects of matter and energy. It delves into the structures and behaviors that are not visible to the naked eye but have significant implications on broader physical theories. Understanding this domain can lead to innovations in technology and materials science. The intersection of physics with microscale studies unravels new materials and techniques that can enhance various applications from electronic devices to medical technologies.
Quantum Mechanics and Microscale
Quantum mechanics is pivotal in exploring microscale phenomena. At this level, particles behave in ways that far exceed classical predictions. For instance, the dual nature of particles—acting as both particles and waves—introduces concepts such as superposition and entanglement. These quantum effects are essential for the development of quantum computing and quantum cryptography, which have vast implications for processing information and securing data.
Researchers are increasingly intrigued by the behavior of particles at the nanoscale, where the principles of quantum mechanics are significantly less observable. As systems become smaller, classical mechanics starts to break down, leading to surprising results like quantum tunneling and the Casimir effect. This indicates the fascinating relationship between size and physical properties, marking the microscale as a frontier for theoretical and experimental physics.
Nanotechnology Innovations
Nanotechnology represents one of the most exciting outcomes of microscale physics. By manipulating matter at the nanoscale, researchers create materials with unique properties that deviate from their larger counterparts.
For example, carbon nanotubes exhibit extraordinary strength and electrical conductivity, leading to developments in electronics, energy storage, and nanomedicine. Techniques such as lithography and chemical vapor deposition enable precision fabrication of structures at the nanoscale.
In nanotechnology, innovations are not just limited to physical properties. The ability to design materials with specific optical characteristics has led to advancements in displays and sensors. Moreover, the manipulation of biological molecules at the nanoscale is paving the way for targeted drug delivery systems in healthcare.
Microscale Measurement Techniques
Accurate measurement at the microscale presents unique challenges due to the very small sizes involved. Traditional measurement techniques are often inadequate. Thus, scientists have developed specialized techniques to quantify microscale phenomena effectively.
- Atomic Force Microscopy (AFM): AFM allows researchers to image surfaces at the atomic level. It employs a mechanical probe to monitor surface forces, revealing topographical features with high precision.
- Scanning Electron Microscopy (SEM): SEM produces detailed images of surfaces by scanning them with a focused beam of electrons. This technique is quintessential for studying materials and their properties at the microscale.
- X-ray Crystallography: This method is essential to understand molecular structures and interactions in the microscale realm. It provides insights into the arrangement of atoms in crystalline solids.
These techniques not only enhance our understanding of microscale physics but also play a vital role in interdisciplinary research. They facilitate the connection between physics, chemistry, biology, and materials science, extending our grasp of phenomena at a fundamental level.
Microscale in Earth Sciences
Microscale phenomena in Earth sciences play a crucial role in understanding complex geological and environmental processes. The examination of these processes at a microscale allows scientists to unravel detailed interactions that are often lost when viewed at broader scales. Recognizing the fine details enhances our comprehension of natural systems and informs our management of resources and environments.
Microscale Geological Processes
At the microscale, geological processes can reveal intricate relationships between minerals, fluids, and biological entities. For instance, studies conducted using scanning electron microscopy have provided insights into crystal structures and their formations. This level of detail is vital when assessing mineral properties, weathering processes, and sedimentary mechanisms. Every small change in these processes can have major implications for larger geological phenomena, such as soil stability, erosion rates, and the availability of earth resources.
"Understanding the microscale can illuminate the pathways through which geologic processes happen, linking them to larger explanations of Earth's evolution."
Soil Composition at the Microscale
Soil is a complex medium composed of minerals, organic matter, liquids, and gases, where microscale analysis can reveal vast information regarding its composition and health. Various techniques, such as X-ray diffraction and micro-CT scanning, are employed to identify soil mineralogy and pore structuring. These analyses help illustrate how different soil particles interact with each other and with water, influencing soil fertility and plant growth. Moreover, the microscale composition of soil can inform land use practices and sustainable agriculture approaches.
Environmental Impact Studies
In environmental impact studies, microscale insights are imperative for assessing pollution and its effects on ecosystems. High-resolution analytical techniques can detect contaminants at low concentrations, which is significant for both remediation efforts and policy formulation. The fate of pollutants, such as heavy metals or pesticides, can be better understood by observing their interactions at the microscale. Ultimately, this detailed understanding informs environmental management efforts and regulatory measures, ensuring that conservation efforts are effective and scientific.
Challenges in Microscale Research
The study of microscale phenomena holds immense significance in various scientific fields. However, it also presents unique challenges that can impede progress. Understanding these challenges is vital for researchers aiming to push the boundaries of knowledge and innovation.
Technical Limitations
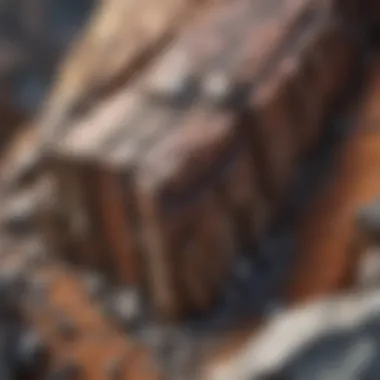
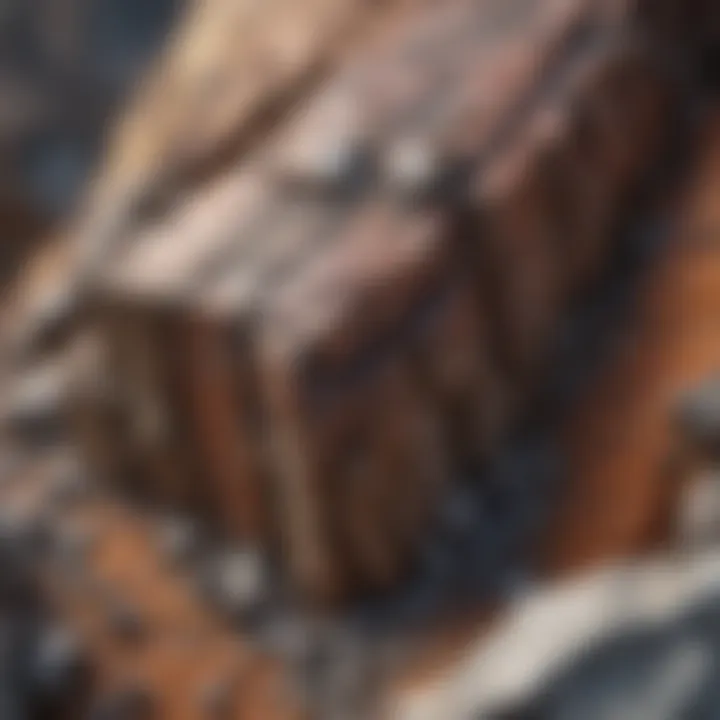
Microscale research often faces technical limitations. These can involve the precision of instruments and the capabilities of current technologies. For instance, measuring features at a microscale may require advanced microscopy techniques, such as atomic force microscopy or scanning electron microscopy. Each method has its own resolution limits and may introduce artifacts or errors during data collection.
Furthermore, fabrication challenges arise when creating microscale structures. Traditional manufacturing techniques may not achieve the desired precision at smaller scales, leading to discrepancies between intended and actual designs. This can hinder the reproducibility of experiments, a critical aspect of scientific research.
Some key technical limitations include:
- Resolution constraints: Many techniques struggle to visualize structures smaller than a certain threshold, limiting the types of materials that can be studied.
- Material constraints: Some materials do not behave predictably at microscale, yielding different properties than at a larger scale.
Data Acquisition Difficulties
Data acquisition at the microscale can be particularly challenging. Collecting meaningful data often requires sophisticated experimental setups and careful calibration of equipment. Researchers must navigate the complexities of sampling methods and data processing to ensure statistical significance.
Another layer of difficulty arises from the sheer volume of data generated during microscale experiments. The large datasets can overwhelm researchers, making it hard to identify relevant patterns or anomalies. This complexity necessitates thorough data management practices and advanced analytical techniques, such as machine learning, to interpret results effectively.
Considerations for data acquisition difficulties include:
- Sampling bias: Ensuring a representative sample is essential but can be difficult to achieve at the microscale.
- Data integration: Combining data from different sources or techniques can pose challenges, impacting analysis and conclusions drawn from research.
"Microscale research not only requires an understanding of the phenomena being studied but also demands innovative approaches to overcome its inherent challenges."
Moving forward, these challenges must be thoughtfully addressed to optimize microscale research and unlock its vast potential in advancing science and technology.
Future Directions in Microscale Studies
The field of microscale studies is evolving rapidly, reflecting advancements in technology and an increasing understanding of complex systems. Future directions hold promise for significant breakthroughs across various scientific disciplines. Researchers must remain adaptive to these changes and embrace interdisciplinary collaboration. Such cooperation can unleash new potentials in microscale research, such as improved materials and enhanced understanding of biological systems.
Emerging Technologies
Emerging technologies will play a pivotal role in shaping the future of microscale studies. Innovations like advanced microscopes, micro-manipulation techniques, and nanofabrication methods are already changing how scientists observe and interact with materials and biological entities at small scales. For instance:
- Atomic Force Microscopy (AFM) enables scientists to obtain high-resolution images of surfaces at atomic levels, enhancing our understanding of material properties.
- Microfluidics allows precise control and manipulation of tiny volumes of fluids, which is crucial for applications in chemistry and biology.
- 3D Printing at microscale offers unprecedented design flexibility in creating complex structures and devices.
These technologies not only expand the toolkit available to researchers but also open new avenues for exploration, enabling the potential for cross-disciplinary applications.
Emerging technologies are revolutionizing the way we study and understand microscale phenomena. The boundaries of research that can be achieved are continually pushed, allowing complex systems to be analyzed with unmatched precision.
Interdisciplinary Approaches
Interdisciplinary approaches are vital for advancing microscale studies. By converging knowledge from different fields, researchers can develop holistic solutions to intricate problems. For example:
- Combining biology and chemistry can lead to the design of better drug delivery systems that operate at the microscale, enhancing the efficacy of treatments.
- Physics and engineering disciplines can contribute insights into the behavior of materials at the nanoscale, fostering innovations in nanotechnology.
- Collaborative research initiatives are crucial for creating sensors that integrate biology and material science, improving environmental monitoring techniques.
Furthermore, universities and research institutions are increasingly recognizing the value of fostering interdisciplinary research groups. This shift promotes an environment where various scientific perspectives intersect, resulting in comprehensive approaches to understanding health, technology, and the environment.
In summary, the future of microscale studies is bright, driven by emerging technologies and interdisciplinary collaboration. As scientists continue to investigate microscale phenomena, the possibilities seem boundless, promising enhanced insights and applications that can profoundly impact society.
Ending
The conclusion of this article serves as a critical synthesis of the complexities associated with microscale phenomena across various scientific disciplines. It emphasizes the overarching significance of microscale research and its multifaceted implications on modern science and technology. As shown throughout the article, the microscale is not merely a niche subject; rather, it underpins much of our understanding in biology, chemistry, physics, and earth sciences.
In particular, this section reinforces the following key elements:
- Interdisciplinary Impact: The integration of knowledge across different fields fosters a richer understanding of microscale processes. This interdisciplinary approach can lead to innovative solutions for complex problems, merging insights from various perspectives.
- Technological Advancements: The continuous evolution of technological tools enhances our ability to explore and manipulate microscale phenomena. Techniques such as atomic force microscopy and microfluidics have transformed research methodologies, making it possible to study subjects at unprecedented resolutions.
- Future Research Directions: The future of microscale research looks promising, with emerging technologies and interconnections between fields paving the way for new discoveries. Researchers are now better equipped to tackle pressing challenges in health, environmental science, and materials development.
When considering the presence of microscale research in various applications, one must recognize its role in addressing global challenges. For example, understanding microbial interactions at the microscale can aid in combating antibiotic resistance, while advancements in nanoscale materials can lead to more sustainable industrial practices.
Overall, the conclusion elegantly encapsulates the vital themes explored throughout the article. It serves as a call to action for scientists, educators, and students alike to engage with and further investigate the vast potentials and implications of microscale phenomena. As research expands, the microscale will continue to play a crucial role in shaping the scientific landscape and addressing the complexities of the world around us.
"Understanding microscale processes is not merely an academic pursuit; it is essential for the advancement of science and technology, impacting countless aspects of our lives."
Summation of Key Points
The final reflections lead us to a summation of the key points discussed in the article:
- Defining Microscale: Understanding the microscale is fundamental to all scientific disciplines.
- Cross-disciplinary Applications: Research at the microscale benefits from the synthesis of knowledge across diverse fields.
- Innovative Techniques: New technology has revolutionized our research capabilities, allowing for complex analyses that were once impossible.
- Environmental and Health Impacts: Microscale research contributes significantly to environmental sustainability and health advancements.
- Future Prospects: The ongoing development in this field presents numerous avenues for exploration and research.
The importance of fostering an interest and deepening our understanding of microscale phenomena is paramount. The accumulation of this knowledge not only serves academic purposes but can also lead to real-world applications that benefit society as a whole. The journey through the intricacies of microscale is just beginning, and it beckons us forward into uncharted territories of scientific inquiry.