Exploring Illumina Sequencing by Synthesis

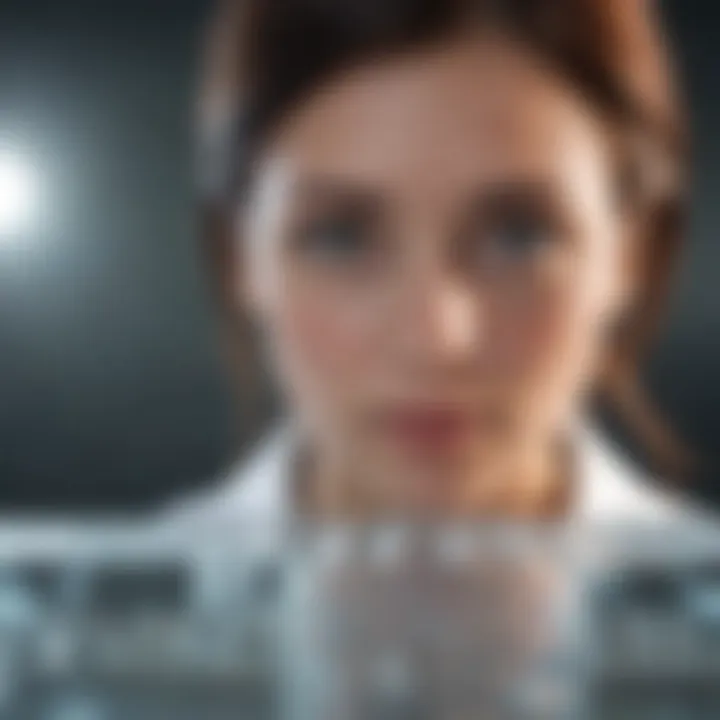
Intro
Illumina sequencing by synthesis is one of the most transformative technologies in the field of genomics. It has redefined how scientists investigate biological systems, offering immense advantages over traditional methods. Not only does it provide high-throughput capabilities, but it also yields accurate and reliable data, making it an indispensable tool in both research and clinical settings.
To fully appreciate the impact of this technology, one must delve into its underlying principles and mechanics. This exploration goes beyond mere technicality; it paints a broader picture of the advancements in genetics and their implications for future studies. Understanding Illumina sequencing is crucial for students, educators, professionals, and researchers as they navigate the complexities of modern science.
Methodology
Study Design
The design of studies utilizing Illumina sequencing often revolves around a clear set of objectives. Whether it’s seeking to identify genetic variations associated with diseases or exploring microbial diversity in environmental samples, the foundational aspect is to ensure that the sequence data acquired aligns with the overarching research question. Protocols must be meticulously crafted to facilitate effective data capture while taking into account factors like sample type, sequencing depth, and read length.
For example, a study investigating a specific genetic disorder may start with a cohort of affected individuals, alongside a control group of healthy subjects. Samples might be collected through blood draws or biopsies, where each sample undergoes an integrated process of library preparation, sequencing, and subsequently analysis.
Data Collection Techniques
Once the study design is in place, data collection techniques come into play. Illumina sequencing employs a unique approach to collect genetic information, primarily through the synthesis of complementary strands of DNA. This involves several key steps:
- Library Preparation: Here, the target DNA is fragmented, and adapters are ligated, preparing it for sequencing.
- Cluster Generation: Each DNA fragment is clonally amplified on a flow cell, forming dense clusters that are readily accessible for sequencing.
- Sequencing by Synthesis: As nucleotide bases are added to the growing strands, fluorescent signals are captured, revealing the sequence in real-time.
The entire process is automated and highly efficient, enabling the sequencing of multiple samples simultaneously. By utilizing these advanced collection techniques, researchers can gather a treasure trove of genomic data that fuels discoveries across a multitude of biological fields.
Discussion
Interpretation of Results
The interpretation of sequencing results is a critical phase that often demands as much attention as the collection of the data itself. Each dataset can unveil unexpected insights, from identifying novel genetic markers to uncovering variations that contribute to disease susceptibility. It is important for researchers to discern not only the significance of their findings but also the breadth of their implications within the wider scientific context.
In practice, the analysis might involve bioinformatic tools and algorithms designed to compare sequences against known databases, enabling the identification of single nucleotide polymorphisms (SNPs) or structural variations that could influence phenotypic traits.
Limitations of the Study
Despite its strengths, Illumina sequencing is not without limitations. One notable drawback is its reliance on the quality of the initial DNA sample. If the DNA is degraded or contaminated, it could lead to inaccuracies in the results. Additionally, the cost of sequencing can be prohibitive for wide-scale studies, especially in lower-resourced settings. The technology also faces challenges in certain applications, like repetitive regions of the genome, where obtaining accurate reads can be problematic.
Future Research Directions
Looking ahead, the future of Illumina sequencing is rife with potential. Advances in technology are continually on the rise, paving the way for more cost-effective and faster sequencing solutions. This not only makes extensive studies more feasible but also pushes the boundaries of personalized medicine.
Additionally, new applications are emerging, such as metagenomics, where researchers can explore microbial communities in various environments. There's also a growing interest in linking genomic data with phenotypic information, enhancing our understanding of how genetics mesh with environmental factors in shaping health and disease.
"Illumina sequencing by synthesis represents not just a step forward in technology, but a leap towards understanding the genetic basis of life itself."
In summary, as tools and methodologies evolve, so too does the landscape of research possibilities. Embracing these advancements allows for deeper exploration and ultimately greater discoveries in the complex interplay of genetics, health, and nature.
Prologue to Sequencing Technologies
Sequencing technologies play a pivotal role in our understanding of genetics and genomics. By decoding the base pair sequences of DNA, researchers can delve into the complex underpinnings of life, which lays the groundwork for various scientific and medical advancements. In today's fast-paced world, the ability to efficiently sequence DNA is not just a luxury but a necessity. Accurate sequencing mechanisms can lead to breakthroughs in personalized medicine, cancer research, and even evolutionary biology, opening doors to new horizons in these fields.
The importance of this topic cannot be overstated; it underpins numerous innovations in biological research. With the emergence of high-throughput sequencing technologies, like Illumina’s methods, the exploration of vast genetic landscapes has become accessible, facilitating studies that were once thought impossible. These advancements not only streamline the research process but also lead to far less resource consumption compared to older methods.
Defining DNA Sequencing
At its core, DNA sequencing is the process of determining the exact sequence of nucleotides within a DNA molecule. This is akin to reading a book where each nucleotide acts as a letter. With advancements in technology, sequencing has evolved significantly from the methodologies of the past. Earlier techniques were labor-intensive and time-consuming, but the advent of automated systems has revolutionized this field. Techniques like Sanger sequencing paved the way for next-generation sequencing (NGS) methods, which allow for the rapid sequencing of entire genomes, thereby providing insights into genetic variations across populations.
In simple terms, being able to decipher a DNA sequence holds immense potential to answer fundamental questions about biology, disease mechanisms, and evolutionary patterns. Understanding differences in these sequences can aid in pinpointing genetic disorders, which is particularly significant in a clinical context.
Chronology of Sequencing Innovations
The journey of DNA sequencing has been marked by several key milestones. Initially, the Sanger method emerged in the late 1970s, enabling scientists to sequence small fragments of DNA with reasonable accuracy. Over time, the need for speed and scale became apparent. This led to the development of various techniques in the following decades:
- 2000: The Human Genome Project was completed, marking a significant achievement in sequencing technology. It set the stage for the future of genomics by providing a reference sequence.
- 2007: Towering leaps were made with the introduction of next-generation sequencing platforms, fundamentally reshaping the landscape. These methods could generate millions of sequences simultaneously, vastly outpacing the capabilities of traditional methods.
- 2010: Continued enhancements in sequencing chemistry and bioinformatics tools improved accuracy and reduced costs dramatically. It allowed labs all over the world to harness sequencing for diverse applications.
- 2020 onwards: Further developments, such as long-read sequencing technologies, promise to resolve complex genomic areas that short-read technologies struggle with. Examples like Pacific Biosciences and Oxford Nanopore Technologies are leading the way in this area.
In summary, the evolution of sequencing technologies represents not just a technical progression but a shift in how we approach biological research and medical diagnostics. Each breakthrough lays a critical foundation for ongoing innovation and discovery.
The Basics of Illumina Sequencing by Synthesis
Illumina sequencing by synthesis has established itself as a cornerstone in genomic research. A firm grasp on the basics of this technology is essential for researchers, clinicians, and educators aiming to utilize its vast capabilities. Understanding the underlying principles and components provides clarity into how this method transforms biological insights and equips further scientific exploration. Moreover, the significance of this topic lies not just in learning about the mechanics, but in appreciating its immense contribution to fields like personalized medicine, evolutionary biology, and beyond.
Core Principles of Sequencing by Synthesis
Illumina sequencing by synthesis revolves around a few fundamental concepts that set it apart from other methodologies. At its heart, this technique relies on the incorporation of fluorescently labeled nucleotides into a growing DNA strand, allowing for real-time monitoring of DNA synthesis. Each nucleotide emits a distinct signal when incorporated, which is captured through optical imaging.
This sequential addition of labeled nucleotides forms a crucial feedback loop, where the process of reading and building DNA occurs in tandem. It allows for massively parallel sequencing, paving the way for high-throughput applications and rapid data acquisition. The ability to sequence millions of fragments simultaneously turns Illumina into a powerhouse in the realm of genomics.

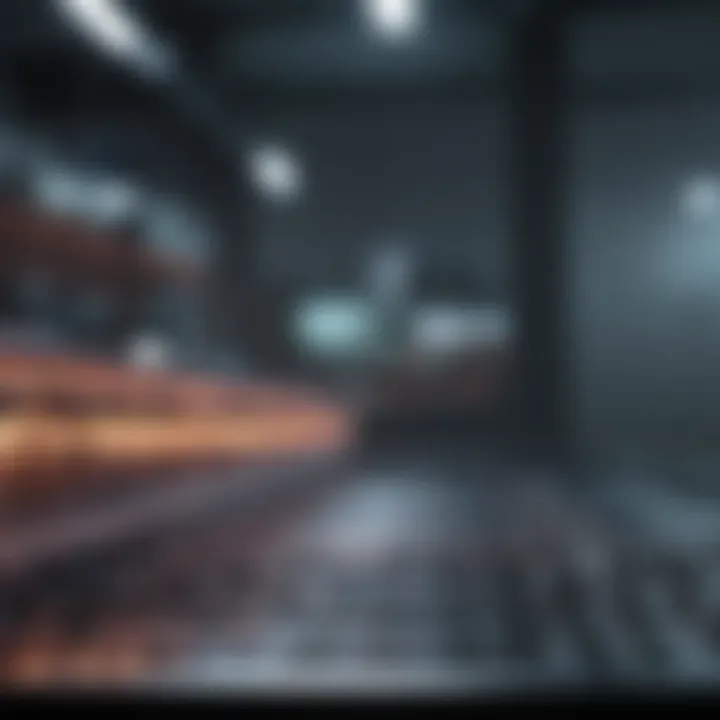
Essential Components and Their Functions
Understanding the essential components that make up the Illumina sequencing apparatus is just as important as grasping its principles. Each component has a unique role that supports the overall workflow, ensuring optimal performance and reliability in sequencing.
Flow Cell
The flow cell serves as the foundational element where the sequencing takes place. This glass slide, often coated with oligonucleotides, allows for the attachment and amplification of DNA fragments. One of the key characteristics of a flow cell is its design that facilitates the efficient capture and clustering of these fragments. The microfluidic channels within it optimize fluid flow, ensuring that reagents are effectively delivered.
A notable advantage of using flow cells in this method is their ability to support massively parallel sequencing, which leads to higher throughput. However, one limitation can arise from clogging or uneven distribution of DNA samples during preparation. Addressing these challenges can enhance the overall fidelity of sequencing runs.
Reagents for Sequencing
Reagents are the unsung heroes of the sequencing process, providing the necessary tools for synthesizing and detecting DNA. The primary reagents include the nucleotide mix, polymerase enzyme, and buffer solutions.
The defining characteristic of these reagents is their ability to facilitate accurate incorporation and signal generation during synthesis. These high-quality reagents ensure that the sequencing runs yield consistent and reliable data. Despite their importance, care must be taken to manage reagent shelf life and storage conditions, as degradation can skew results.
Imaging Systems
Closing the loop in the Illumina sequencing process is the imaging system. This sophisticated setup captures the fluorescent signals generated by the nucleotides as they are added to the growing DNA chain. One key feature of imaging systems is their high-resolution capabilities, which allow for precise identification of nucleotide incorporation.
These systems are integral to achieving high accuracy in sequencing results. However, real-time imaging can introduce complexity, as fluctuating light conditions may affect signal detection. Researchers must keep in mind that maintaining optimal imaging conditions is crucial to preserving the quality of the resulting data.
"Understanding these components and their functions is crucial for anyone looking to utilize Illumina sequencing effectively in their research and applications."
In summary, the basics of Illumina sequencing by synthesis encompass a range of principles and components that work harmoniously. These factors not only contribute to the technology's popularity but also underscore its significance across varied applications in scientific inquiry.
Operational Workflow of Illumina Sequencing
The operational workflow of Illumina sequencing is a cornerstone of the technology that not only defines its efficiency but also highlights its significance in genomic research. Each step in this intricate process plays a crucial role, from preparing the DNA samples to capturing the final data that researchers will analyze. Understanding these steps provides insights into how Illumina sequencing has revolutionized the way we read genomic information.
Sample Preparation and Library Construction
Before sequencing can occur, effective sample preparation is essential. The quality of the nucleic acid material significantly impacts the overall outcome of the sequencing process. Initially, the target DNA is fragmented into smaller pieces, often using physical or enzymatic methods. This fragmentation ensures the DNA can be handled more easily and fits into the sequencing apparatus seamlessly.
Once fragmented, the process of library construction begins. Unique adapter sequences are ligated to the ends of these fragments, which serve two precise purposes: they allow for amplification during the sequencing process and facilitate the attachment of these DNA fragments to the flow cell. This step is critical for ensuring that the sequences can be amplified in a way that generates a robust dataset to work with. The library preparation can be time-consuming, but its meticulous nature ensures that the final sequencing results are of high quality.
Cluster Generation on Flow Cells
After the library has been constructed, the next logical step is loading the DNA onto the flow cell. This is where the magic begins! The flow cell is coated with oligonucleotides complementary to the adapter sequences on the DNA fragments. When the DNA library is introduced, each fragment adheres to the surface of the flow cell, forming individual clusters.
The beauty of cluster generation lies in its exponential amplification. Each bound fragment acts as a template for PCR amplification, yielding thousands of copies in a defined area. These densely populated clusters are essential because they enable the detection of distinct signals during the sequencing process, amplifying the signal strength and improving the accuracy of the captured data.
Sequencing Steps Explained
Once the clusters are generated, the sequencing process can commence. This section breaks down the core sequencing steps, detailing how nucleotides are incorporated, how fluorescent signals are imaged, and the role of algorithms in base calling.
Incorporation of Nucleotides
The incorporation of nucleotides is a critical aspect of the sequencing process. During each sequencing cycle, a single fluorescently labeled nucleotide is introduced to the reaction mix. The key characteristic of this step is that it enables the determination of the DNA sequence based on the flow of information from one nucleotide to another.
This method is widely adopted because it is efficient and highly precise. Each nucleotide carries a specific fluorescent dye, which is attached to a blocking group that prevents any further extension of the growing DNA strand until the dye is imaged. This step ensures that only one nucleotide is incorporated at a given time, thus maintaining accuracy in sequence determination. The removal of the blocking group after imaging allows for the next cycle to begin.
Imaging of Fluorescent Signals
Following the incorporation of nucleotides, the next major phase is the imaging of fluorescent signals. This involves capturing the emitted light from the clusters after each nucleotide incorporation, which is crucial for identifying which nucleotide was added at every step.
The unique feature of this imaging process is its capability to gather high-resolution images at a rapid pace, literally taking snapshots of a billion clusters in mere moments. This sheer volume of data contributes significantly to the effectiveness of Illumina sequencing, allowing it to achieve a high throughput. However, the complexity of the imaging system also means that proper calibration and maintenance are mandatory for reliable results.
Base Calling Algorithms
Finally, the cycle concludes with the application of base calling algorithms. After imaging, the data gathered must be translated into readable sequences of nucleotides. Base calling is an algorithmic process where the intensity of each pixel corresponding to a cluster is analyzed to determine which nucleotide was incorporated.
The beneficial characteristic of base calling algorithms is their ability to handle vast amounts of data effectively, converting raw images into a structured format that researchers can analyze. Modern algorithms employ machine learning to improve accuracy and reduce error rates, focusing on distinguishing true signals from background noise. Even though these algorithms are robust, challenges still exist in the interpretation of complex genomic signals, reminding researchers that ongoing refinement is essential.
"The operational workflow's precision ensures that the rich data generated can lead to new discoveries in genetics and medicine."
Understanding the operational workflow of Illumina sequencing by synthesis underscores its impact on many fields. Each step, from sample preparation to data analysis, takes place in a systematic manner to guarantee that the results are not only accurate but also reliable and actionable. This thorough methodology is at the heart of the technology's widespread adoption and success in modern genomic studies.
Advantages of Illumina Sequencing
The landscape of genomics has been reshaped by the advent of Illumina sequencing by synthesis, bringing with it a host of advantages that enhance both research capabilities and clinical applications. This section breaks down the key benefits of this technology, providing an insight into its pivotal role in advancing scientific understanding.
High Throughput Capabilities
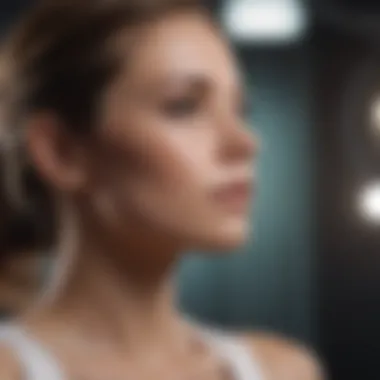
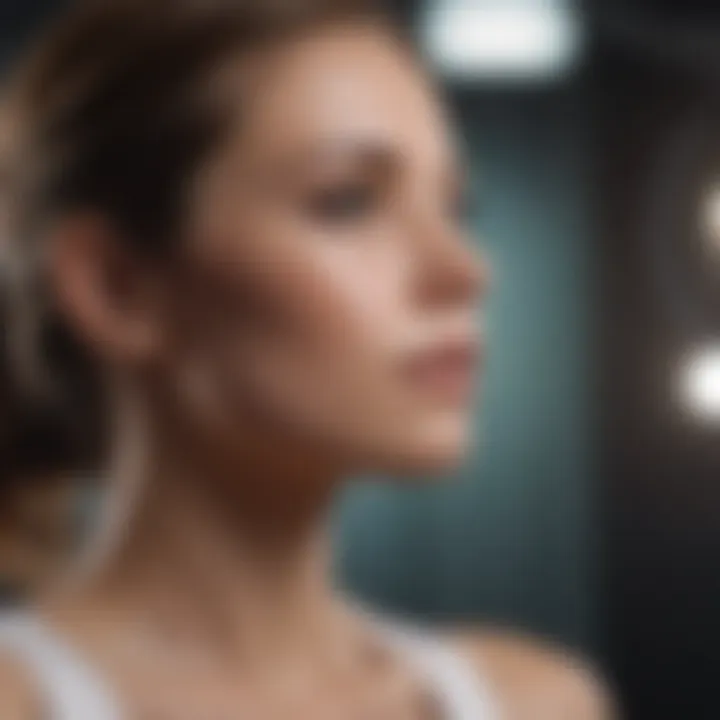
One of the standout features of Illumina sequencing is its high throughput capabilities. This allows for the simultaneous processing of millions of DNA fragments, significantly accelerating the rate of discovery. Researchers can generate vast amounts of data in a single run, a feat that traditional methods simply cannot match.
- Scalability: As the demand for genomic data grows, Illumina's platform can easily scale up to meet these needs, making it suitable for both small and large studies.
- Multiplexing: Researchers can utilize barcoding to sequence multiple samples in one workflow. This not only maximizes resource usage but also provides a comprehensive view of the genetic landscape in a cost-efficient manner.
The ability to conduct large-scale experiments with such efficiency has transformed how scientists approach complex genomic questions, enabling studies that were previously unimaginable.
Cost-Effectiveness Compared to Other Methods
In the realm of genomic sequencing, the cost is more than just a number; it influences research viability and the ability to conduct large-scale population studies. Compared to other sequencing methods, Illumina offers a cost-effective solution without compromising quality.
- Affordability: The per-sample cost in Illumina sequencing has drastically reduced over the years, making it accessible for a broader range of research institutions and laboratories.
- Economic Efficiency: With the ability to analyze multiple samples simultaneously, research teams see savings in both reagents and time.
This economic advantage opens doors for diverse applications, from environmental genomics to precision medicine, where budget constraints often limit research endeavors.
Accuracy and Error Rates
Illumina sequencing shines in terms of accuracy, a non-negotiable feature in genomic research. With its sophisticated error correction algorithms and robust protocols, it stands out among competitors.
- Robust Error Correction: The technology employs several layers of control to minimize the chances of errors in the sequencing process, ensuring the reliability of the data generated.
- High Fidelity: Studies have shown that the error rates in Illumina sequencing are significantly lower than those of other high-throughput systems, giving researchers confidence in their findings.
"The accuracy of Illumina sequencing not only enhances trust in data but also facilitates further explorations within genomic research, which can lead to groundbreaking discoveries."
In sum, the advantages of Illumina sequencing are manifold. Its high throughput capabilities, cost-effective nature, and exceptional accuracy position it as a cornerstone technology in genomics, fostering innovations and driving discoveries across various disciplines.
Applications of Illumina Sequencing
The applications of Illumina sequencing are vast and varied, underscoring its significance in genomics. This technology not only enables researchers to delve deep into the genetic blueprints of organisms, but it also facilitates clinical diagnostics. Each application reaffirms Illumina's position as a cornerstone technology in the modern scientific landscape.
While genomic research has seen the lion's share of application, sectors such as clinical diagnostics and transcriptomics are also experiencing a sea change thanks to Illumina sequencing. Understanding these applications sheds light on how Illumina technology not only supports current research but also paves the way for future investigations.
Applications in Genomic Research
Whole Genome Sequencing
Whole genome sequencing (WGS) is a powerful application of Illumina sequencing that literally reads the entire genetic code of an organism. This comprehensive approach allows researchers to unravel complex questions about genetic variations, evolutionary biology, and species identity.
A distinguishing characteristic of WGS is its ability to detect single nucleotide polymorphisms (SNPs) and structural variations comprehensively. This feature makes it invaluable for population genetics studies, enabling scientists to pinpoint genetic adaptations that help organisms thrive in specific environments.
WGS is a favorable choice due to its thoroughness, but it's not without drawbacks. The sheer volume of data produced necessitates robust computational resources and extensive bioinformatics expertise to analyze and interpret. Nevertheless, the information yielded often outweighs the challenges posed.
Targeted Sequencing
In contrast to WGS, targeted sequencing hones in on specific genomic regions, usually associated with particular traits or diseases. This application allows researchers to obtain high-resolution data on genes of interest. By focusing on a smaller subset of the genome, researchers can efficiently generate more data while conserving resources.
This method's key trait is its cost-effectiveness, making it particularly attractive for large-scale studies, such as clinical trials or familial studies of genetic disorders. By concentrating on relevant genomic regions, targeted sequencing often results in faster turnaround times and valuable insights, though it may miss broader genomic context.
Exome Sequencing
Exome sequencing is another crucial approach that focuses on the exome, the portion of the genome necessary for coding proteins. Given that less than two percent of the genome constitutes exons, this method provides a streamlined way of identifying genetic variations influencing protein function.
The key characteristic here is that exome sequencing can often highlight mutations that lead to diseases, particularly rare genetic disorders. It represents a cost-effective means of gaining significant data about clinically relevant genes, balancing depth with breadth.
However, the limitation of exome sequencing lies in its inability to capture regulatory regions or non-coding elements of the genome. This can sometimes lead to incomplete understandings of underlying genetic factors, a concern that researchers must keep in mind.
Role in Clinical Diagnostics
Cancer Genomics
Illumina sequencing plays a crucial role in cancer genomics, allowing for a deeper understanding of tumor evolution and heterogeneity. By identifying mutations associated with various forms of cancer, it offers potential pathways for personalized therapies and targeted treatments.
A notable aspect of cancer genomics is that it provides a landscape of the tumor's genetic alterations, which can help oncologists tailor treatment plans specific to the molecular profile of a patient’s tumor. This approach increases the likelihood of therapeutic success but requires robust follow-up studies to validate findings.
Genetic Disorders
In the realm of genetic disorders, Illumina sequencing offers a lifeline for diagnostics, particularly in understanding hereditary conditions. The ability to detect mutations in coding regions allows specialists to elucidate the genetic underpinnings of many diseases.
The paramount feature of genetic disorder diagnostics using Illumina technology is the swift identification of pathogenic variants. This timely knowledge can guide clinical decisions, impacting treatment strategies and family planning. Yet, interpreting the identified genetic anomalies requires skilled expertise and often leads to perplexing discussions surrounding variants of uncertain significance.
Implications in Transcriptomics
Both Illumina sequencing and its capabilities in transcriptomics are transforming our understanding of gene expression. Through technologies like RNA-seq, transcription profiles can be documented in real time, providing insights into what genes are active under certain conditions.
This application lays a foundation for investigating complex and multi-faceted biological processes, opening avenues for studying gene regulatory networks and differential expression in various tissues or conditions.

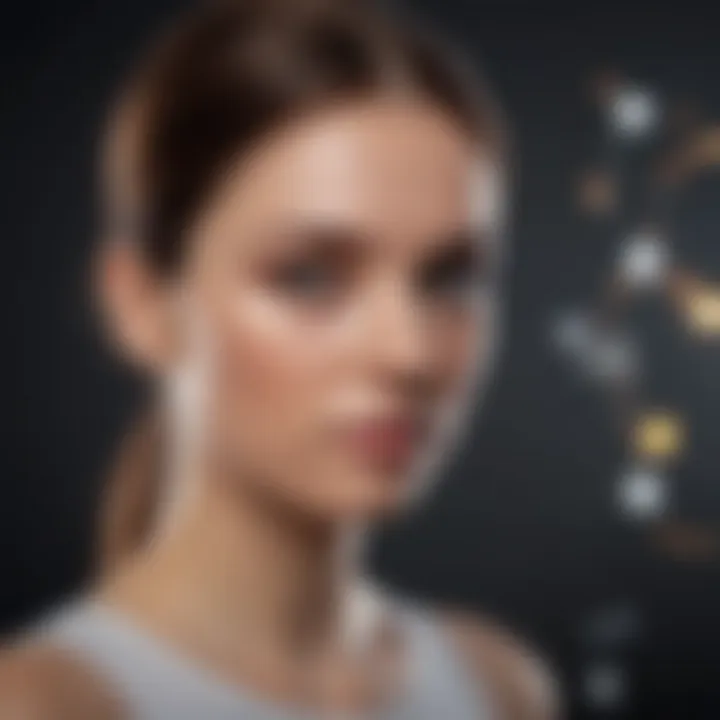
In summary, the applications of Illumina sequencing not only drive scientific inquiry but forge critical connections across diverse disciplines, marking a transformative era in genomics.
Challenges and Limitations of Illumina Sequencing
Illumina sequencing by synthesis has transformed the field of genomics, enabling researchers to decode genetic information with remarkable speed and accuracy. However, like any technology, it’s not without its hurdles and weaknesses. Understanding these challenges is vital because they inform the development of strategies to overcome them, ensuring that the technology can deliver results effectively.
Handling of Complex Genomic Regions
When it comes to sequencing complex genomic regions, Illumina technology faces a particular set of difficulties. These regions often contain repetitive sequences, structural variants, or high GC content that can interfere with accurate read mapping. Repeats can lead to misalignment, and in some cases, the sequence may not be represented adequately in the output data. Moreover, regions with high GC content can exhibit reduced efficiency in sequencing reactions, potentially leading to biased coverage. In essence, while Illumina excels at sequencing the less complex parts of the genome, it stumbles when dealing with the intricate landscapes of complex genomic regions.
Limitations in Read Length
One of the notable limitations of Illumina sequencing is its relatively short read lengths in comparison to other technologies. The standard reads, typically around 150 to 300 base pairs, can pose significant challenges in comprehending long repetitive sequences or structural complexities. When analyzing larger genomes or those with vast regions of homology, it becomes tricky to piece together the data accurately from shorter reads. Longer read sequencings, like those produced by Pacific Biosciences or Oxford Nanopore, can provide better continuity in assembly, particularly for complex genomic regions, but they come with their own trade-offs in terms of throughput and cost.
Data Interpretation and Analysis Issues
The rich datasets generated by Illumina sequencing bring their own challenges regarding interpretation. The sheer volume of data can overwhelm standard analysis tools, requiring sophisticated computational power and software to make sense of it all. Furthermore, distinguishing between true variants and sequencing artifacts is not always straightforward. Algorithms used in base calling and variant calling can vary, each with their strengths and weaknesses. This variability might lead to different interpretations of the same dataset, creating potential discrepancies in findings. As a result, researchers must be equipped with not only the technology to sequence but also the know-how to analyze and interpret the data effectively.
"Data is the new oil, but only if we know how to refine it."
While Illumina sequencing has advanced our understanding of genomics substantially, it is crucial to acknowledge and address these challenges. As the field of genomics evolves, so too must the technologies, ensuring they remain robust under a range of biological scenarios.
Future Directions in Sequencing Technologies
Sequencing technologies continue to evolve at a breakneck pace. This section sheds light on the emerging trends, innovations, and challenges that shape the future of sequencing, particularly regarding Illumina sequencing by synthesis. As scientific demands grow, the ability to produce accurate and comprehensive genomic data becomes essential. Understanding these future directions not only enhances the application of genomic medicine but also broadens the horizons of biological research.
Advancements in Read Length and Accuracy
Improving read length while maintaining accuracy is paramount in the race to decode DNA effectively. Longer reads significantly enhance the assembly of complex genomes, particularly in species with highly repetitive sequences. Researchers are actively working on optimizing sequencing methods to push beyond the limitations seen in traditional short-read sequencing. As the technology progresses, the hope is to achieve longer reads that still exhibit the high accuracy that Illumina is known for.
- Longer reads allow for more comprehensive genomic mapping.
- They help mitigate issues related to repetitive regions often problematic in genome assemblies.
Advancements here will mean that not only will we sequence genomes with better fidelity, but the analyses will reveal more about structural variations within the genome.
Integration of AI in Data Analysis
Artificial Intelligence is becoming woven into the fabric of genomic data analysis. The sheer volume of data generated through sequencing brings about challenges in interpretation and management. Integrating AI-driven solutions can streamline data processing, providing a more robust framework for analysis. Machine learning algorithms can identify patterns and potential anomalies in genomic data.
"AI in genomic research acts as a lighthouse in the fog of data complexity, guiding scientists to key insights."
This integration equips researchers with powerful tools to better understand genetic variants and their implications in diseases, potentially paving the way for tailored treatment strategies. The future will likely see AI becoming an indispensable ally in genomic studies, pushing the boundaries of what is currently achievable.
Emerging Alternative Sequencing Techniques
While Illumina sequencing stands as a powerful tool in genomics, various alternative technologies are making their mark. These new methods can complement or offer solutions where Illumina may face limitations, especially in specific research contexts.
Nanopore Sequencing
Nanopore sequencing is an innovative approach that provides real-time results with an ease that is often compared favorably with traditional methods. With its ability to sequence long fragments of DNA, it addresses some of the challenges linked to complex genomic regions. Its key characteristic is the ability to read DNA strands directly as they pass through a nanopore, providing insights into both the sequence and structure of the DNA molecules.
One unique feature of nanopore sequencing is its portability, allowing field-based genomic studies that were previously impractical. However, it does have some disadvantages, such as a generally higher error rate compared to Illumina. Despite this, its rapid developments make it a notable player in future sequencing technologies, particularly where time and flexibility are critical.
Single-Molecule Real-Time Sequencing
Single-Molecule Real-Time Sequencing (SMRT) captures the essence of sequencing in real-time, offering deep insights into the genetic material with minimal biases. A key characteristic of SMRT is its capacity to observe nucleotide incorporation as it happens, rather than after the fact. This real-time approach can be crucial for dynamic processes where timing and accuracy are essential.
The unique feature of SMRT is its ability to generate long reads while simultaneously providing information about the methylation of the bases. This ability enhances understanding of epigenetic modifications in genomes. However, challenges remain regarding throughput and the comprehensive analysis of data produced, which may not be as robust as that generated by Illumina technologies.
Culmination
Illumina Sequencing by Synthesis has carved out its niche as a cornerstone in the realm of genomics. Understanding its significance requires looking beyond the mechanics to appreciate the vast implications it has on both scientific research and medical diagnostics. By integrating sophisticated technology with streamlined processes, it grants researchers an unparalleled ability to decipher the complex code of life itself.
Summary of Key Insights
When one analyzes the journey illuminated throughout this article, several key insights emerge:
- Core Technology: The principles of sequencing by synthesis, particularly its reliance on fluorescently labeled nucleotides, revolutionized how scientists read genetic sequences.
- Operational Excellence: From sample preparation to data analysis, each step in the Illumina workflow is finely tuned for efficiency. This allows for high-throughput sequencing that can process vast amounts of data in a fraction of the time required by older technologies.
- Versatile Applications: The applications extend far and wide—from genomic research, probing the intricacies of whole genomes, to clinical diagnostics, where it aids in understanding cancer genomics and genetic disorders. Additionally, its role in transcriptomics delineates the interplay between genes and their expression.
- Future Outlook: Advancements on the horizon indicate a more interconnected future for sequencing technologies, especially with the integration of artificial intelligence in data interpretation and analysis.
Through this lens, the value of Illumina sequencing is unmistakable. It’s not merely about reading DNA; it’s fundamentally about unlocking the secrets that lie within.
The Impact of Illumina Sequencing on Modern Science
Illumina sequencing stands as a testament to how a single technology can reshape entire fields of study. Its impact can be summarized through several critical developments:
- Accelerating Discovery: With its rapid output, researchers can now generate whole-genome sequences that would have taken years, if not decades, to compile manually. This acceleration leads to faster discoveries in evolutionary biology, genetics, and beyond.
- Driving Personalized Medicine: In the medical realm, tailored treatments based on individual genetic profiles are quickly becoming a reality. The precision of Illumina sequencing enables clinicians to identify genetic mutations responsible for diseases, allowing for customized therapeutic approaches.
- Enhancing Collaborative Research: The availability of extensive genomic data has fostered a collaborative spirit among researchers across disciplines. Large consortiums like the 1000 Genomes Project have arisen, relying on Illumina sequencing to generate an extensive catalog of human genetic variation.
- Shaping Public Health Initiatives: With the swift identification of pathogens during outbreaks, Illumina sequencing has enhanced the capabilities of public health organizations to respond effectively to emerging infectious diseases.
The holistic influence of Illumina sequencing remains profound, not just in accelerating our scientific inquiry but also in guiding our future directions in health, ecology, and human biology.
As we continue to unravel the complexities of life at a molecular level, Illumina sequencing will no doubt remain at the forefront, guiding our understanding and keeping curiosity alive in the scientific community.