The Essential Role of ATP in Cellular Function
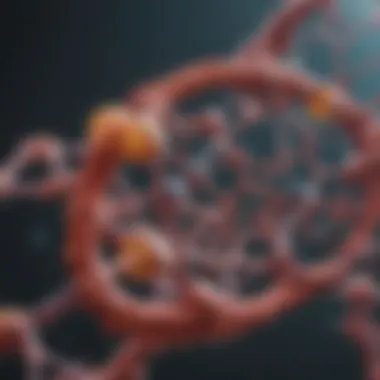
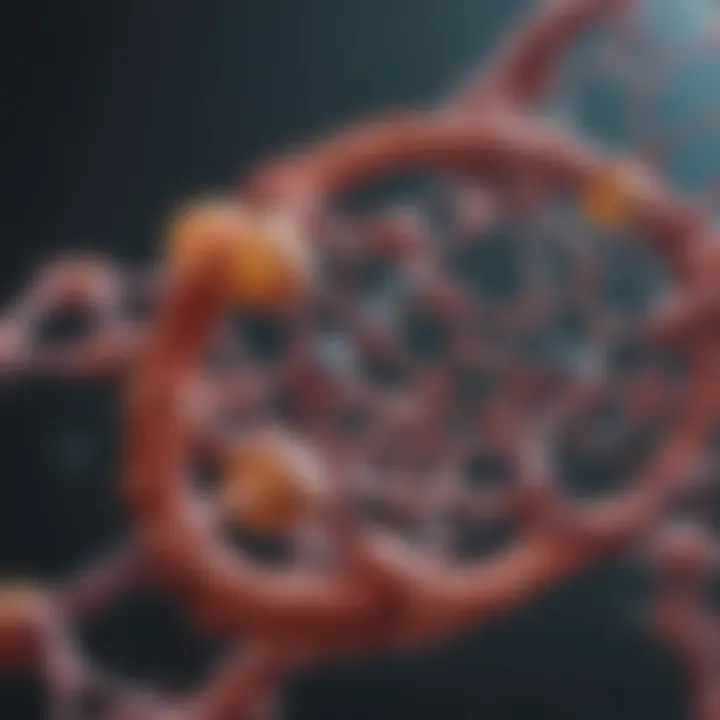
Intro
Adenosine triphosphate (ATP) is a critical molecule in biochemistry. It serves as the primary energy currency of cells, allowing for the storage and transfer of energy in biological systems. Understanding ATP's roles and mechanisms is vital for students and professionals alike, who aim to grasp the intricate workings of cellular processes. This exploration will dissect ATP's structure, function, and its importance in metabolism and disease.
ATP consists of three phosphate groups, ribose sugar, and adenine base. The high-energy bonds between the phosphate groups make ATP an efficient energy donor. When ATP breaks down into adenosine diphosphate (ADP) and inorganic phosphate, it releases energy that fuels numerous cellular activities. This transformation is not just a simple chemical reaction, but a key driver of life at the molecular level.
Furthermore, ATP plays essential roles in diverse metabolic pathways. It is integral in biosynthetic reactions, muscle contraction, and active transport across cell membranes. The balance of ATP synthesis and degradation is crucial for maintaining cellular health and function. Its production primarily occurs through cellular respiration and photophosphorylation.
This article aims to deeply analyze ATP's multifaceted roles and highlight recent advancements in ATP research. Insights into how ATP is linked to various physiological conditions will also be discussed, shedding light on its future implications in biotechnological applications.
Foreword to Adenosine Triphosphate
Adenosine triphosphate, commonly known as ATP, is often referred to as the energy currency of the cell. Understanding ATP is critical because it plays a fundamental role in almost all cellular processes. In this section, we will explore what ATP is, its chemical structure, and how its discovery has shaped our understanding of biochemistry.
Definition and Chemical Structure
ATP is a nucleotide composed of three key components: adenine, ribose, and three phosphate groups. The structure can be described simply. Adenine is a nitrogenous base, ribose is a five-carbon sugar, and the three phosphate groups are linked by high-energy bonds. This arrangement is crucial. When a cell needs energy, it hydrolyzes ATP, breaking off one of the phosphate groups. The energy released from this reaction fuels various biochemical reactions essential for life.
The chemical formula of ATP is C106N5O13P3. This indicates carbon, hydrogen, nitrogen, oxygen, and phosphorus atoms are involved, emphasizing the complexity of ATP's structural integrity.
Historical Background of ATP Discovery
The discovery of ATP dates back to the early 20th century. In 1929, the British scientist Sir Frederick Hopkins is credited with isolating ATP. However, it was not until the 1940s and 1950s that ATP's true significance in energy transfer was fully appreciated. During this period, chemists like Richard F. Heck and others contributed greatly to elucidating ATP’s role in metabolism and enzymatic reactions.
This growing body of research shifted scientific focus onto ATP, recognizing it as vital for cellular processes. Its discovery paved the way for further inquiries into cell metabolism, physiology, and biochemistry. Today, ATP is recognized not just in cellular energy transfer but also in several other biological functions, including signaling pathways and muscle contraction.
Biochemical Properties of ATP
Adenosine triphosphate (ATP) is vital for life, serving as the primary energy carrier in cells. Understanding its biochemical properties gives insight into its functions and impact on cellular processes. This section examines the molecular composition and physical properties of ATP, highlighting their implications for biology and health.
Molecular Composition of ATP
ATP's molecular structure consists of three primary components: adenosine, ribose, and triphosphate. The adenosine moiety includes adenine, a nitrogenous base, and ribose, a five-carbon sugar. The triphosphate tail contains three phosphate groups linked by high-energy bonds. These bonds are critical; when broken, they release energy used by cells for various functions. The specific arrangement of these components contributes to ATP's stability and utility in cellular processes.
To elaborate:
- Adenine facilitates the recognition of ATP by enzymes and receptors.
- Ribose supports structural integration within nucleotides, linking ATP to other nucleic acids like DNA and RNA.
- Phosphate groups are the source of energy during hydrolysis, where the terminal phosphate group is released, converting ATP into adenosine diphosphate (ADP).
This unique structure makes ATP an effective energy currency in living organisms. It's crucial in biochemical reactions, as cells utilize ATP to perform work.
Physical Properties and Stability
ATP's physical properties are essential for its role in biological systems. The molecule is relatively stable at physiological pH, but it has a tendency to hydrolyze, especially when exposed to water or certain enzymes. Stability is vital for ATP's storage and transport in cells.
Key physical properties include:
- Solubility: ATP is soluble in water, allowing it to circulate freely within the cell and reach various biochemical pathways. This solubility supports its functions in energy transfer and metabolic reactions.
- Reactivity: The high energy of phosphate bonds makes ATP reactive. This reactivity underpins its ability to participate in numerous biochemical reactions swiftly.
ATP’s stability under normal conditions and propensity for reactive breakdown allows cells to maintain energy levels effectively. The balance between stability and reactivity is crucial for sustaining life processes.
ATP’s high-energy bonds are the key to its role as an energy carrier, making it integral to cellular metabolism and function.
The Role of ATP in Cellular Energy Transfer
Adenosine triphosphate (ATP) serves as the primary energy currency in biological systems. Understanding the role of ATP in cellular energy transfer is essential for grasping how cells function and maintain life. ATP is involved in almost every cellular process, from muscle contraction to active transport across membranes. Its significance cannot be underestimated.
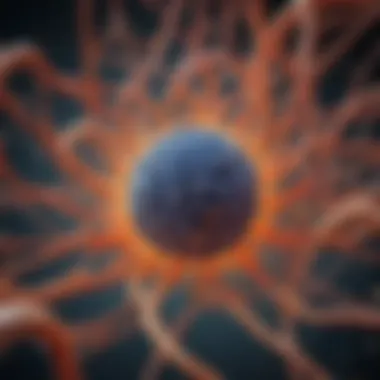
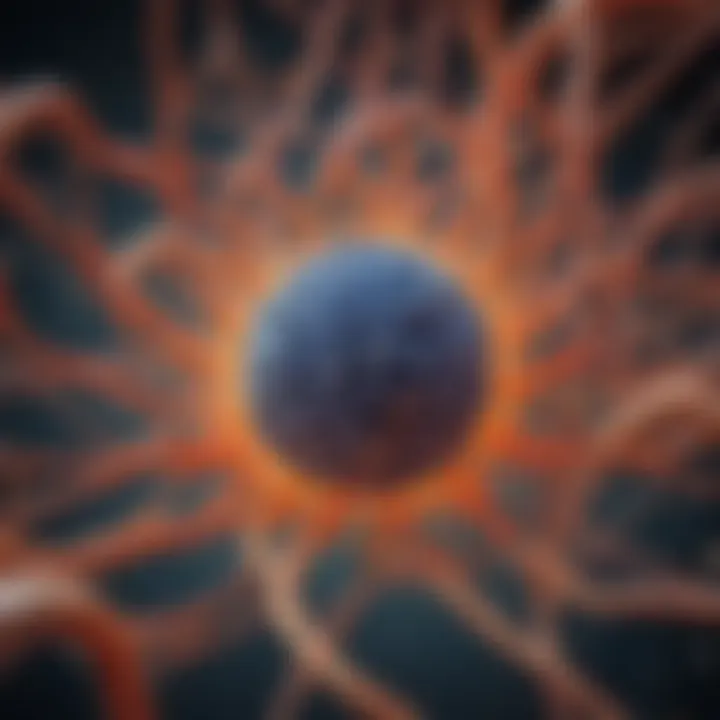
The mechanisms by which ATP releases energy are intricate and vital. ATP molecules contain three phosphate groups. The bonds between these phosphate groups, particularly the high-energy bond between the second and third phosphates, are crucial. When ATP is hydrolyzed, it releases energy that can be utilized for various cellular activities. This process is a cornerstone of cellular respiration, as it provides the necessary energy for sustaining life.
Mechanisms of Energy Release
ATP hydrolysis is the primary mechanism through which energy is released. When ATP reacts with water, it undergoes a reaction that breaks down the molecule into adenosine diphosphate (ADP) and an inorganic phosphate (Pi). This reaction releases energy because the products are more stable and have lower free energy than ATP.
- Reaction:
ATP + O → ADP + Pi + Energy
Energy from ATP can be harnessed in two primary ways:
- Direct Work: Energy is used directly for various cellular reactions like biosynthesis.
- Coupled Reactions: ATP can drive endergonic reactions through coupling. For instance, ATP facilitates the synthesis of glucose from smaller substrates, which would not occur spontaneously. The energy release from ATP hydrolysis is used to drive these reactions forward.
ATP can also be regenerated from ADP through the addition of a phosphate group, usually during cellular respiration processes, allowing cells to maintain a continuous energy supply.
ATP and Cellular Work
ATP is essential for various forms of cellular work. These include:
- Mechanical Work: This is seen in muscle contraction. Myosin heads in muscle fibers use ATP to change shape and generate force.
- Transport Work: ATP provides energy for the active transport of molecules across membranes. For example, the sodium-potassium pump uses ATP to move sodium out of and potassium into the cell against their concentration gradients.
- Chemical Work: ATP is crucial for driving necessary chemical reactions, such as the synthesis of macromolecules like proteins and nucleic acids.
The efficiency of ATP in transferring energy makes it an ideal energy carrier. All cellular machinery relies on ATP to convert energy from nutrients into usable forms, ensuring that biological processes can function properly. Its role is fundamental for life as we know it, and fluctuations in ATP levels can lead to critical consequences for cellular function.
"ATP is the universal energy currency of the cell, playing a key role in facilitating biological reactions essential for life."
In summary, ATP's performance as an energy carrier illustrates its central role in energy transfer processes within cells. Through mechanisms of energy release and its contributions to various types of cellular work, ATP is indispensable for maintaining the functions of living organisms.
Synthesis of ATP
The synthesis of adenosine triphosphate (ATP) is a critical aspect of cellular metabolism and energy transfer. Energy is essential for all cellular processes, and ATP serves as the primary energy currency in biological systems. Understanding how ATP is synthesized provides deep insights into cellular mechanics and overall metabolic function.
Substrate-Level Phosphorylation
Substrate-level phosphorylation is one of the two main processes through which ATP is generated within cells. This method occurs in the cytoplasm during glycolysis and within the mitochondria during the Krebs cycle.
During glycolysis, glucose is broken down into pyruvate, resulting in a net gain of two ATP molecules per glucose molecule. This reaction does not require oxygen, which makes it crucial during anaerobic conditions. The enzymes involved facilitate the transfer of a phosphate group from a substrate directly to adenosine diphosphate (ADP), forming ATP.
In the Krebs cycle, substrate-level phosphorylation occurs through the conversion of succinyl-CoA to succinate. Here, a phosphate group is transferred to ADP, again yielding ATP. This method of ATP synthesis is efficient and does not depend on electron transport.
Oxidative Phosphorylation in Cellular Respiration
Oxidative phosphorylation is the second primary pathway for ATP synthesis and primarily occurs in the mitochondria. This process is crucial for efficient ATP production, especially in aerobic organisms. It involves the electron transport chain and chemiosmosis.
In oxidative phosphorylation, electrons are transferred through protein complexes within the inner mitochondrial membrane. These electrons come from NADH and FAD, which are produced during earlier metabolic pathways. As electrons move through the chain, they release energy. This energy pumps protons out of the mitochondrial matrix, creating a proton gradient across the inner membrane.
The return flow of protons into the matrix through ATP synthase drives the conversion of ADP and inorganic phosphate into ATP, producing a significant amount of energy. This method yields approximately 28 to 30 ATP molecules per glucose molecule, highlighting its efficiency compared to substrate-level phosphorylation.
Overall, both substrate-level and oxidative phosphorylation are essential for synthesizing ATP in cells. Each method contributes to the total ATP output, supporting various cellular activities that require energy. Understanding these synthesis pathways underlines the complexity and efficiency of cellular energy management.
Degradation of ATP
The degradation of adenosine triphosphate (ATP) is a vital process that impacts cellular activities. ATP plays a central role as an energy currency in various biochemical reactions. However, it must also be broken down to regulate cellular function carefully. Understanding this process reveals insights into cellular metabolism, energy flow, and even points towards implications for diseases.
Hydrolysis of ATP
ATP hydrolysis is the main pathway through which ATP is degraded. This process involves the reaction of ATP with water, resulting in the release of energy. The reaction leads to the formation of adenosine diphosphate (ADP) and an inorganic phosphate molecule (Pi). The reaction can be represented as:
[ \textATP + \textH_2\textO \rightarrow \textADP + \textPi + \textEnergy ]
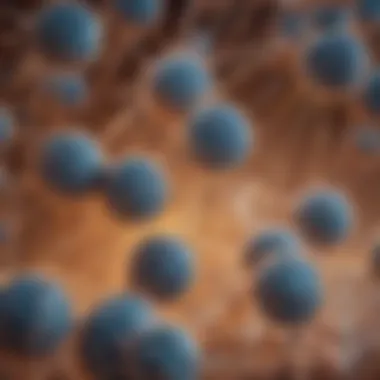
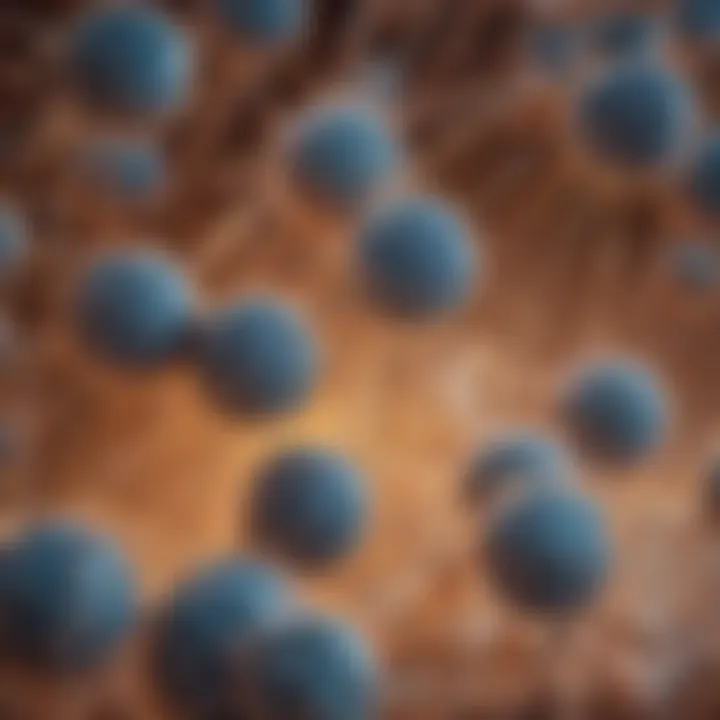
The hydrolysis of ATP is exergonic. This means it releases energy that can be harnessed for cellular activities. The energy released during this process is fundamental for muscle contraction, movement of ions across membranes, and the synthesis of macromolecules. This fundamental reaction underscores ATP's dual role: as both a provider of energy and a substrate for further reactions.
Roles of ATPase Enzymes
ATPase enzymes play a significant role in the degradation of ATP. These enzymes catalyze the hydrolysis of ATP to ADP and Pi. Different types of ATPases exist, serving various functions in cells. Here are some of the key roles they perform:
- Energy Transfer: ATPases enable the energy from ATP to be transferred to substrates, facilitating essential cellular processes.
- Ion Transport: Certain ATPases are involved in pumping ions across cell membranes, crucial for maintaining cellular homeostasis and electrical gradients. An example includes the sodium-potassium pump, which maintains cellular ion concentrations.
- Muscle Contraction: Myosin ATPase activity is essential for muscle contraction. The hydrolysis of ATP provides the energy required for muscle fibers to contract.
The reaction rates of ATPases may vary depending on the cellular environment and the specific needs of the cell. Their activity is tightly regulated to ensure appropriate energy supply.
"The regulated breakdown of ATP ensures that cells not only harness but also precisely control energy use within cellular processes."
In summary, the degradation of ATP, particularly via hydrolysis and the action of ATPase enzymes, is fundamental for sustaining life at the cellular level. Understanding these processes contributes to our knowledge of metabolism, energy dynamics, and overall cellular health.
ATP in Metabolic Pathways
Adenosine triphosphate (ATP) plays a central role in metabolic pathways, serving as a critical energy currency in biological systems. Understanding ATP’s involvement in these pathways provides insight into how living organisms manage energy and process substrates for various functions. This section will elucidate ATP's significance in two major metabolic processes: glycolysis and the Krebs cycle.
ATP's Role in Glycolysis
Glycolysis is a fundamental metabolic pathway that converts glucose into pyruvate, producing energy in the form of ATP. The process occurs in the cytoplasm and can be divided into two phases: the energy investment phase and the energy payoff phase. During the initial stages of glycolysis, two ATP molecules are consumed to phosphorylate glucose and its derivatives, making it more reactive. This is crucial because it prepares glucose for subsequent breakdown.
As the pathway progresses, energy is released, leading to the production of four ATP molecules during the energy payoff phase. The net gain of ATP, therefore, is two molecules per glucose molecule decomposed. This transformation is essential for cells because it provides immediate energy supply for cellular functions and initiates further oxidative pathways.
ATP, in this context, highlights the interplay between light and dark reactions in photosynthesis, especially in plant cells, where sugars created from light energy might enter glycolysis for energy production. The balance of ATP produced and utilized in glycolysis is vital for maintaining cellular metabolism and ensuring adequate energy supply for diverse biological processes.
ATP in the Krebs Cycle
The Krebs cycle, also known as the citric acid cycle or tricarboxylic acid (TCA) cycle, is a crucial metabolic pathway that takes place in the mitochondria of eukaryotic cells. This cycle is integral to aerobic respiration, where acetyl-CoA, derived from carbohydrates, fats, and proteins, is oxidized to produce energy. In the cycle, ATP generation occurs indirectly through the synthesis of electron carriers such as NADH and FAD. These electron carriers transport electrons to the electron transport chain, leading to the ultimate production of ATP via oxidative phosphorylation.
During one full turn of the Krebs cycle, three NADH and one FAD are produced, along with one GTP, which can be readily converted to ATP. Although the direct ATP yield from the Krebs cycle is modest, its significance lies in supporting the electron transport chain, where the majority of ATP synthesis occurs—showcasing the efficiency of cellular respiration.
In summary, ATP serves as a linchpin linking glycolysis and the Krebs cycle, ensuring the smooth transition between energy extraction from substrates and energy transfer to cellular functions. The effective function of these metabolic pathways is paramount for cellular homeostasis and overall organismal health.
ATP is more than an energy substrate; it is a critical connector in cellular metabolism, illustrating the dynamic relationship between different organelles and pathways.
Physiological Significance of ATP
Adenosine triphosphate (ATP) is often described as the energy currency of the cell. Its physiological importance goes well beyond this simplified view. ATP is critical for a range of cellular processes. Understanding its significance provides insight into several mechanisms of life.
ATP plays a vital role in muscle contraction. When muscles contract, they require energy to shorten and produce force. This energy comes from ATP. The process begins when ATP is hydrolyzed into adenosine diphosphate (ADP) and phosphate, releasing energy. The myosin heads in muscle fibers use this energy to pivot and pull actin filaments during contraction.
Key points regarding ATP’s role in muscle contraction include:
- The immediate source of energy for muscle cells.
- The vital function of ATP in connecting biochemical energy transfer to mechanical work in muscles.
- The rapid turnover of ATP during sustained muscle activity, demonstrating its importance in maintaining muscle performance.
Given this, ATP not only serves as a fuel but also facilitates coordination between biochemical activities and muscular movements.
"ATP is critical for sustaining the energy demands of muscle tissues, illustrating the intricate connection between biochemistry and physiology."
Additionally, ATP is critical in neurotransmission. Neurons rely on ATP to maintain the sodium-potassium pump. This pump is essential for creating the membrane potential necessary for propagation of nerve impulses.
In neurotransmission, ATP has several roles:
- Sustaining membrane potential: By ensuring the correct ion concentrations across the membrane, it allows neurons to fire effectively.
- Modulating signaling pathways: ATP acts as a signaling molecule in glial cells, influencing neurotransmitter release and uptake.
- Facilitating vesicle fusion: ATP is required for the release of neurotransmitters from synaptic vesicles.
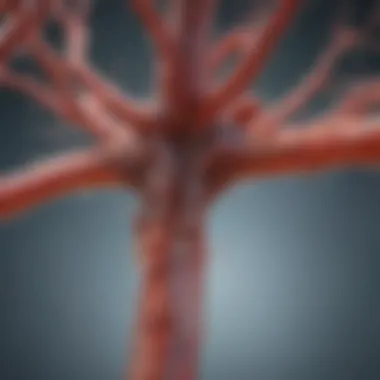
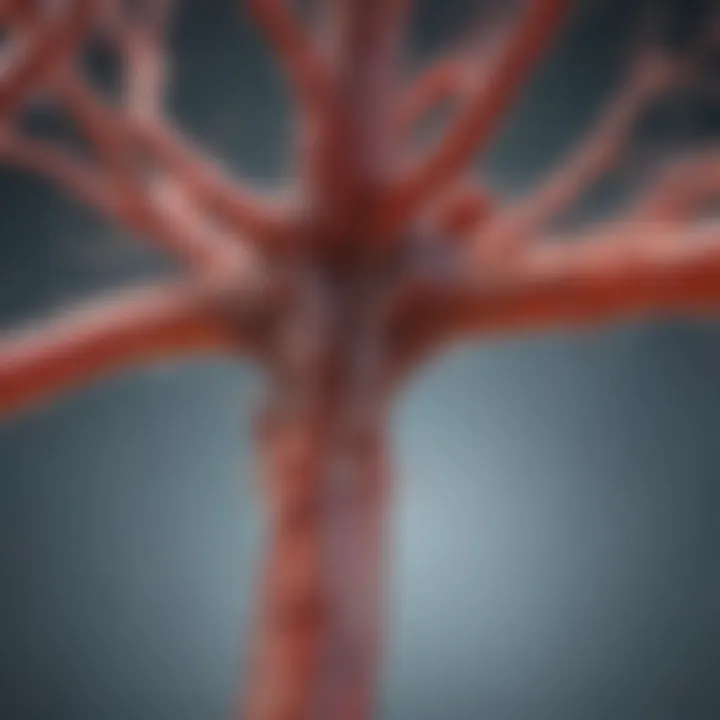
Thus, ATP's physiological impact is evident in muscle contraction and neurotransmission. Its roles in energy transfer across multiple cellular systems signify its foundational importance in life processes. Through ongoing research into ATP, more insights could emerge that illuminate its contribution to health and disease.
Challenges in ATP Research
Research focused on adenosine triphosphate (ATP) presents unique challenges that are critical to understand. ATP is fundamental in cellular function, and its proper measurement and understanding in various contexts directly impact not only biochemical studies but also therapeutic applications.
Measuring ATP Levels in Cells
Accurate measurement of ATP levels in cells is one of the prominent challenges in ATP research. Cells maintain ATP concentrations at relatively stable levels. However, factors such as cell type, metabolic state, and the external environment can affect these concentrations. Techniques for measuring ATP levels vary in effectiveness and sensitivity. Common methods include bioluminescence assays and high-performance liquid chromatography (HPLC).
- Bioluminescence assays use luciferase, an enzyme that catalyzes the conversion of luciferin to produce light in the presence of ATP. This method is sensitive but can be affected by factors like light exposure and the presence of other nucleotides.
- HPLC allows for the separation and quantification of ATP and other nucleotides. While precise, it often requires complex preparation and may not be suitable for measuring ATP levels in live cells.
The variability among methods creates complexity in studying ATP dynamics. As a result, it remains challenging to correlate ATP levels and cellular function accurately. Because of the importance of precise measurements, it necessitates constant refinement of techniques.
Understanding ATP's Role in Disease
Assessing the role of ATP in various diseases adds another layer of complexity. ATP is involved in numerous biochemical pathways that intersect with various disease processes. Diseases such as cancer, neurodegenerative disorders, and metabolic syndromes have all exhibited altered ATP dynamics.
In cancer, for instance, tumor growth is associated with increased ATP production. This enhanced energy supply aids in supporting rapid cellular proliferation. However, this phenomenon also leads to a higher demand for ATP, making it essential to understand how ATP metabolism changes in such contexts.
Neurodegenerative diseases, including Alzheimer's and Parkinson's, often see disruptions in ATP synthesis. This disruption can trigger cellular death pathways, indicating the significance of ATP in maintaining neuronal health. Thus, research into ATP's roles in these diseases could lead to therapeutic strategies aimed at restoring normal ATP levels or improving ATP utilization.
Understanding these intricate mechanisms requires a multi-faceted approach, combining biochemistry, molecular biology, and clinical insights. This interdisciplinary nature complicates the study but is necessary for unraveling ATP's contributions to both health and disease.
"The study of ATP is not merely about understanding a molecule; it's about deciphering the myriad processes that underlie life itself."
Biotechnological Applications of ATP
Adenosine triphosphate (ATP) plays a significant role in biotechnological applications. Its importance is rooted in its function as an energy source and a signaling molecule. The unique properties of ATP make it vital in various research and industrial processes. Understanding these applications can lead to enhanced techniques and methods in biotechnology.
ATP in Biochemical Assays
Biochemical assays often utilize ATP as a reference point. Many assays measure ATP levels to assess cell viability or metabolic activity. For example, the luciferase assay is a widely used technique that quantitatively measures ATP levels in cells. The reaction involves the enzyme luciferase, which catalyzes the conversion of luciferin, releasing light in the presence of ATP. This light output can be detected and correlated to the amount of ATP present in the sample. This method is highly sensitive and allows for real-time monitoring of cellular processes.
"ATP quantification is essential in determining cellular health, metabolism, and activity levels of microorganisms."
In addition to luciferase, other assays use ATP in enzyme-linked methods or for calibrating responses. The reliable detection of ATP concentrations helps in drug development, toxicology, and environmental studies. Certain ATP-based biosensors are being developed to monitor ATP in living systems, enhancing our understanding of cellular dynamics.
Potential Therapeutic Uses of ATP
ATP's therapeutic potential is an area of growing interest. One area is its use in regenerative medicine. Recent studies indicate that ATP can promote tissue repair and regeneration. Direct administration of ATP has shown promise in wound healing due to its role in increasing cellular energy and signaling pathways that facilitate recovery.
Furthermore, ATP can act as a signaling molecule in the nervous system. It has been suggested to have neuroprotective effects. Research indicates that ATP can improve outcomes in conditions like stroke or neurodegenerative diseases. By modulating neuronal activity, ATP may help to preserve or restore function, warranting further exploration.
Moreover, ATP is being researched for its application in drug delivery systems. Encapsulating ATP within nanoparticles can provide controlled release mechanisms in targeted therapies. This could maximize therapeutic effects while minimizing side effects, raising its potential as a treatment strategy in various diseases.
The exploration of ATP in biotechnological frameworks highlights its significance beyond traditional roles, paving the way for innovative applications that enhance medical science and biotechnology.
End and Future Directions
The exploration of adenosine triphosphate (ATP) emphasizes its critical role in countless cellular functions. The research surrounding ATP is not merely academic; it holds practical implications for health, biotechnology, and understanding metabolic disorders. In this conclusion, the vital elements and future directions in ATP research become clearer.
ATP serves as the primary energy currency in cells, facilitating various biochemical reactions necessary for life. The insight gained from studying ATP's mechanisms can lead to breakthroughs in medicine, particularly in diseases where energy metabolism is disrupted. For instance, conditions like diabetes or muscular dystrophies could be better understood by delving deeper into ATP dynamics. Moreover, ongoing advancements in biotechnology are creating new avenues for ATP applications. The potential therapeutic uses mentioned earlier could revolutionize treatment approaches.
Summary of Key Concepts
- Energy Transfer: ATP is integral for energy transfer within cells, providing the necessary fuel for metabolic processes.
- Synthesis and Degradation: Understanding how ATP is synthesized and degraded clarifies its role in maintaining cellular energy balance.
- Physiological Relevance: From muscle contraction to neurotransmission, ATP’s functions are diverse and essential.
- Challenges in Research: Measuring ATP levels and understanding its implications in diseases are ongoing challenges.
- Biotechnological Applications: The versatility of ATP in biochemical assays and potential therapies showcases its significance in research and clinical settings.
Implications for Future Research
Future investigations into ATP should continue to focus on its multifaceted roles. Some specific areas for future research include:
- Investigating ATP Levels in Disease: There is a need for refined techniques to measure ATP concentrations in various disease states to correlate them with disease severity.
- ATP as a Therapeutic Agent: Exploring pharmacological approaches that utilize ATP could open new frontiers in treatment strategies.
- Role in Aging: Research can also delve into how ATP metabolism changes with age and its impact on age-related diseases.
- Engineering ATP-Dependent Systems: In biotechnology, creating synthetic pathways that harness ATP could lead to innovative applications in energy storage and conversion.