The Essential Functions of Cells in Human Health
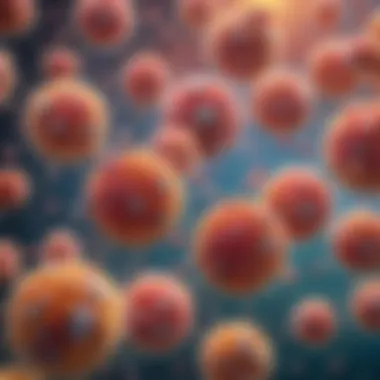
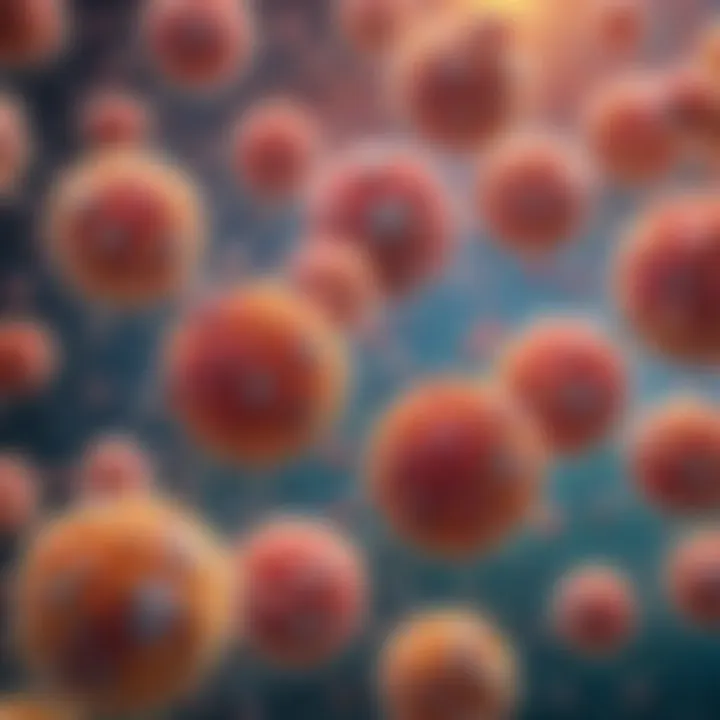
Intro
The human body is often seen as a complex machine, with each part playing a crucial role in maintaining overall function and balance. At the heart of this intricate system are cells, the fundamental units of life. These microscopic entities are far more than mere building blocks. They exhibit an astounding variety of types, each with specialized functions that sustain bodily processes and health.
In this article, we’ll explore the integral nature of cells in our physiology. From their structural characteristics to the fascinating roles they play in sustaining homeostasis, understanding cells illuminates much about health, disease, and life itself. As we delve deeper, we will uncover layers of cellular communication and pathways that highlight how vital these tiny forms of life are to human existence. Ideally, the insights offered will enhance the comprehension of cellular biology, helping students, researchers, educators, and professionals appreciate the significance of these microscopic marvels.
Here, we will start with a discussion of the methodology to uncover the intriguing world of cells.
Prelims to Cells
Cells are not just the basic units of life; they are the very essence of what makes organisms function, survive, and thrive. In the realm of human biology, understanding the integral role of cells is akin to unlocking the secrets of life itself. Their significance stretches far beyond individual existence; they form the foundation of our tissues, organs, and ultimately our entire physiology. This section aims to highlight the importance of cells, providing a lens through which we can appreciate their multifaceted contributions to health and disease.
Definition of Cells
A cell can be simply defined as the smallest structural and functional unit of an organism. These microscopic powerhouses are packed with machinery designed to carry out countless functions necessary for life. They come in various shapes and sizes, each tailored to meet specific needs of the organism. For instance, red blood cells are uniquely designed to transport oxygen, while muscle cells are structured to facilitate movement.
From an elementary perspective, every living thing—be it a bacterium or a blue whale—is built from cells. This universality underscores their critical role in biology, providing a compelling reason to delve deeper into their characteristics, varieties, and functionalities.
Historical Perspective
Historically, the journey into the world of cells dates back to the invention of the microscope in the 17th century. This groundbreaking tool allowed scientists to peer into the tiny world of cells, giving birth to cell biology as a field.
Key Discoveries
Among the pivotal discoveries in cell biology was the realization that all living organisms are composed of cells. This principle, known as the cell theory, was established in the mid-19th century. Scientists such as Theodor Schwann and Matthias Schleiden highlighted that not only plants but also animals are built of cells, marking a monumental shift in biological sciences.
These discoveries have paved the way for countless research avenues aimed at understanding cellular functions and their implications on health. The key characteristic of these findings lies in their ability to provide a unifying framework in biology. Understanding cells has allowed researchers to delve into more complex biological concepts, ultimately linking cellular form to function.
Advancements in Microscopy
The advancements in microscopy technology significantly contributed to cell biology. From the simple light microscope to sophisticated electron microscopes, each leap forward has enabled deeper insights into the cellular world. Electron microscopy, for instance, allowed scientists to visualize cell structures at a much higher resolution, revealing details such as organelles and cellular interactions.
The unique feature of these advancements lies in their ability to provide unprecedented clarity and detail. This precision is particularly beneficial for fields like medicine and genetics, as understanding cellular structures can lead to breakthroughs in treating diseases at the cellular level. However, the complexity of operating advanced microscopy tools can be a barrier for some researchers, emphasizing the need for comprehensive training in this area.
In summary, the introduction of cells lays the groundwork for our understanding of human biology, leading us to comprehend the complex web woven by cellular interactions, their structure, and their functions. Diving into the minute details of cells enriches not only our scientific knowledge but also our approach to health and disease.
Types of Cells in the Human Body
Understanding the different types of cells in the human body is fundamental to grasping how our bodies function. Each type of cell has its unique role, and together they contribute to the complexity of biological processes and organ systems. By exploring this topic, one gains insights into not just the cells themselves, but also the significance of their interactions in maintaining health and facilitating life. This section will discuss how cells can be categorized primarily into eukaryotic and prokaryotic types, and delve deeper into somatic and germ cells, showcasing their importance in various bodily functions.
Eukaryotic and Prokaryotic Cells
The distinction between eukaryotic and prokaryotic cells serves as a cornerstone of cellular biology. Eukaryotic cells, characterized by a membrane-bound nucleus and organelles, comprise all complex living organisms, including humans. They are larger and more intricate, allowing for specialized functions and greater adaptability in multicellular settings. On the other hand, prokaryotic cells, which lack a true nucleus and membrane-bound organelles, are generally simpler and are typically found in bacteria and archaea. This simplicity allows them to thrive in various environments, making them essential in ecosystems and as part of the human microbiome.
Here are some key differences:
- Eukaryotic Cells:
- Prokaryotic Cells:
- Found in plants, animals, fungi, and protists
- Larger in size (10-100 micrometers)
- Contain specialized organelles like mitochondria and Golgi apparatus
- Complex cellular functions, multi-cellularity
- Represented mainly by bacteria
- Smaller size (0.1-5.0 micrometers)
- Lack membrane-bound organelles
- Simple cellular functions, unicellular existence
Knowing these distinctions emphasizes the evolutionary trajectory leading to complex life forms and the role of eukaryotic cells in supporting intricate systems like the human body.
Somatic Cells
Somatic cells are the building blocks of most tissues in the human body, excluding germ cells (sperm and egg cells). They play critical roles in growth, repair, and maintenance of bodily functions. Understanding somatic cells and their functions lays a foundation for comprehending broader biological processes. These cells are diploid, meaning they contain two sets of chromosomes.
Examples of Somatic Cells
Under the umbrella of somatic cells, we find various types including muscle cells, nerve cells, and epithelial cells, each adapted for distinct functions:
- Muscle Cells: Enable movement through contraction
- Nerve Cells (Neurons): Facilitate rapid communication throughout the body
- Epithelial Cells: Cover surfaces and line cavities, crucial for protection and absorption
These examples demonstrate the remarkable specialization of somatic cells, which makes them crucial components of the human anatomy, aiding in everything from physical movement to information transmission and nutrient absorption.
Functions of Somatic Cells
Somatic cells are essential for a multitude of bodily functions:
- Tissue Repair: Facilitate healing by replacing damaged cells
- Homeostasis: Regulate internal environments through metabolic activities
- Growth and Development: Support the organism's growth trajectory
This functionality underscores the critical role somatic cells play in sustaining overall health. Each type has unique features that allow for specialized roles within tissues, and their integrated activities make them indispensable to the organism's integrity.
Germ Cells
Germ cells hold a special status in biological processes as they are integral to reproduction. They are haploid, containing only one set of chromosomes, and their primary function is to enable genetic continuity by participating in the formation of gametes.
Role in Reproduction
The role of germ cells in reproduction cannot be overstated. They power sexual reproduction by combining genetic material from two parents to form offspring. This fusion occurs during fertilization, where a sperm cell meets an egg cell. Their unique characteristic of being haploid ensures that when they unite, the resulting zygote restores the diploid number of chromosomes, establishing a new organism. This generational leap in genetic variability fuels evolution.
Genetic Material Transmission
Germ cells are pivotal in the transmission of genetic material. The genetic code carried by these cells influences traits that may be passed on to future generations.
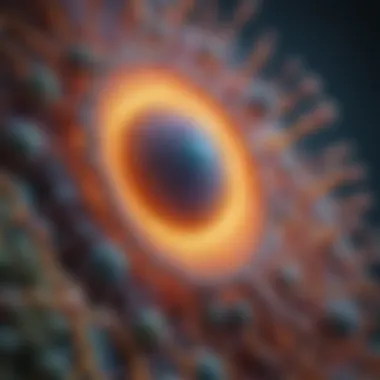
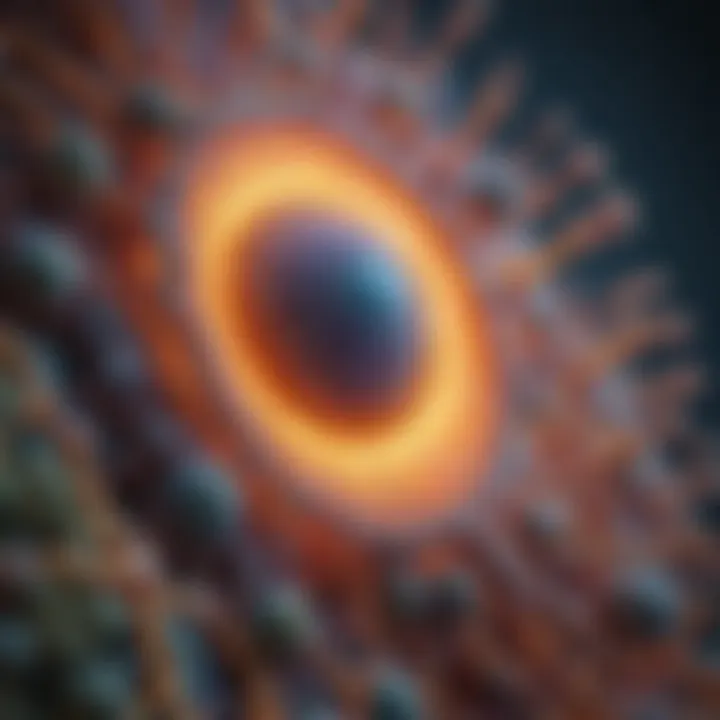
- Mitosis and Meiosis: Germ cells undergo meiosis, a specialized form of cell division that produces haploid gametes, ensuring genetic diversity through recombination and independent assortment.
This method of genetic transmission opens the door to the concept of inheritance, affecting everything from appearance to susceptibility to diseases, making germ cells instrumental in the continuity of species.
"Germ cells are not just carriers of genetic material; they are the architects of the biological lineage, ensuring diversity and adaptation in a changing world."
Structure of Cells
Understanding the structure of cells is pivotal, as it sets the groundwork for appreciating how they function within the human body. Just like a well-constructed building needs solid foundations and proper design, cells rely on their structure to execute their roles efficiently. The cell's architecture, particularly the composition of the cell membrane and the various organelles within the cytoplasm, plays a significant role in maintaining cellular integrity and facilitating communication among cells. This section will delve into these components, showcasing their importance in not just cell functionality but also in the broader context of human health.
Cell Membrane Composition
The cell membrane acts as the gatekeeper for the cell, controlling the entry and exit of substances.
Phospholipid Bilayer
The phospholipid bilayer is a fundamental aspect of the cell membrane. It consists of two layers of phospholipids, where the hydrophobic (water-repelling) tails face inward, shielded from the water, while the hydrophilic (water-attracting) heads face outward towards the aqueous environment. This arrangement creates a semi-permeable barrier.
A key characteristic of the phospholipid bilayer is its fluidity. This quality allows proteins and lipids to move freely within the layer, facilitating various cellular processes like signaling and nutrient transport. Its flexibility is beneficial because it enables cells to adapt to varying conditions without losing their essential functions.
However, this fluidity might also have disadvantages, such as making the membrane susceptible to certain toxins and pathogens that exploit these properties for entry into the cell. The dynamic nature of the bilayer highlights the delicate balance cells maintain in upholding their functions.
Membrane Proteins
Membrane proteins are crucial components embedded within the phospholipid bilayer. They fulfill a variety of roles including acting as receptors, channels, or enzymes that aid in the transport of substances across the membrane. A notable characteristic of these proteins is their specificity; they are designed to interact with specific molecules or ions, which enhances the cell's ability to respond to changes in the environment.
The advantageous feature of membrane proteins is that they can facilitate communication between cells by transmitting signals from the exterior to the interior, triggering necessary cellular responses. Despite their importance, one downside is that malfunctions or mutations in these proteins can lead to various diseases. Thus, their proper functioning is pivotal for maintaining overall homeostasis.
Cytoplasm and Organelles
The cytoplasm is the jelly-like substance that fills the cell, housing various organelles, each with specific functions. Understanding the roles of these organelles clarifies how cells perform their necessary tasks.
Function of Organelles
Organelles are specialized subunits within the cell, each performing distinct functions vital for the cell’s survival. For instance, mitochondria are known as the powerhouses of the cell, generating ATP through cellular respiration.
The key characteristic of organelles is compartmentalization. This ensures that various biochemical processes can occur simultaneously without interference, optimizing efficiency. Such organization is essential in the fast-paced environment of cellular activities, facilitating tasks such as synthesis, degradation, and storage of substances. However, organelles can be vulnerable to stressors like toxins and reactive oxygen species, which may impair their function and ultimately lead to cell damage.
Organelle Interactions
The interactions between organelles are equally important in understanding cellular dynamics. These interactions ensure that the various parts of the cell work in concert to achieve the overall cell goals. For example, ribosomes produce proteins that are then modified and packaged in the endoplasmic reticulum and Golgi apparatus.
One notable feature of organelle interactions is their dependency on the cytoskeletal network, which aids in the transport of materials within the cell. This network ensures that organelles are positioned correctly and maintain their functionalities. A downside to these interactions might be that if one organelle fails, it can disrupt the entire cellular processes, leading to broader implications for cellular health.
The intricate structure and interactions of cells underscore their importance in maintaining health and facilitating life processes.
Cell Functionality
Understanding cell functionality is like peering into the engine room of a sophisticated machine. Cells don't just exist; they perform a myriad of functions that are essential for the smooth operation of our body. This section aims to unpack the vital roles cells play in energy production and protein synthesis, highlighting how these processes contribute to overall health and vitality.
Energy Production
Cellular Respiration
Cellular respiration stands at the forefront of energy production. This intricate series of metabolic reactions allows cells to convert nutrients into usable energy. Essentially, it's the body's way of turning what we consume into fuel for our biological processes. The key characteristic that makes cellular respiration so crucial is its efficiency; it taps into glucose and oxygen, breaking them down to harvest energy in the form of adenosine triphosphate (ATP).
This process is both fantastic and complex, involving various stages, including Glycolysis, the Krebs Cycle, and the Electron Transport Chain. Each of these stages contributes uniquely. For example, Glycolysis occurs in the cell’s cytoplasm and initiates the breakdown of glucose, providing a quick source of energy, while the Krebs Cycle and Electron Transport Chain happen within the mitochondria, where the bulk of ATP is produced. A unique feature of cellular respiration is the oxidative phosphorylation stage, which creates a large output of ATP, thereby playing a significant role in meeting the energy demands of the body.
However, while efficient, cellular respiration does have its drawbacks. Oxygen is a necessity, and in the absence of it, cells can't produce energy in this manner; they resort to less efficient anaerobic processes instead.
ATP Generation
Now, speaking of ATP generation, it's the crown jewel of energy production. ATP, or adenosine triphosphate, serves as the primary energy currency within cells. Without ATP, not much gets done; think of it as the power supply that drives every cellular function.
The key characteristic of ATP that solidifies its significance in this article is its versatility. ATP provides energy for various cellular processes, from muscle contraction to nerve impulse transmission. What's particularly fascinating is how quickly cells regenerate ATP. Under normal conditions, ATP is produced and consumed continuously through cellular respiration, allowing for a constant energy supply.
A unique feature of ATP generation is the enzyme ATP synthase, which catalyzes the formation of ATP during oxidative phosphorylation. This enzyme is a marvel of nature, converting the energy from a proton gradient generated during respiration into the chemical bond of ATP. The disadvantage, though, is that ATP cannot be stored in high amounts. Once synthesized, it's often used rapidly, meaning organisms must continually produce it, which ties back to effective cellular respiration.
Protein Synthesis
Role of Ribosomes
Moving on to another essential aspect of cell functionality; protein synthesis is truly the lifeblood of any cell. At the heart of this process are ribosomes. These cellular machineries are responsible for translating genetic information into proteins. They can be found either floating freely in the cytoplasm or bound to the endoplasmic reticulum, where they play a collaborative role in synthesizing proteins destined for secretion or for use within cellular membranes.
A key characteristic of ribosomes is their composition, which consists of ribosomal RNA (rRNA) and proteins. This structural combination allows them to carry out the crucial task of assembling amino acids into polypeptide chains based on mRNA templates. This precise function is why ribosomes earn a special mention in discussions about cellular functionality.
Their unique feature lies in their ability to carry out many cycles of translation, producing proteins in large quantities. However, ribosomes can also be a vulnerability point in a cell, as they can be affected by drugs that inhibit protein synthesis, potentially leading to cell dysfunction.
Transcription and Translation Process
Finally, let’s delve into the transcription and translation process. This is a two-step mechanism that starts with transcription, where DNA is transcribed into messenger RNA (mRNA). This mRNA then travels from the nucleus to the ribosomes, where translation occurs. The importance of this process cannot be overstated, as it translates the genetic code into functional proteins that are vital for cellular structure and activities.
The key characteristic of the transcription and translation process is its sequential nature. First, the genetic information is copied into mRNA, then decoded to create proteins. This systematic approach ensures that cells produce the necessary proteins in a regulated manner, crucial for maintaining homeostasis.
A unique feature of this whole operation is the phenomenon of alternative splicing during transcription. It allows a single gene to code for multiple proteins, enhancing the diversity of proteins that can be produced by a cell. However, missteps in transcription or translation can lead to dysfunctional proteins, which may contribute to diseases, highlighting the need for precise regulation.
Cell Communication
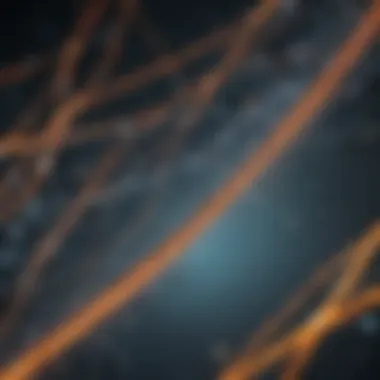
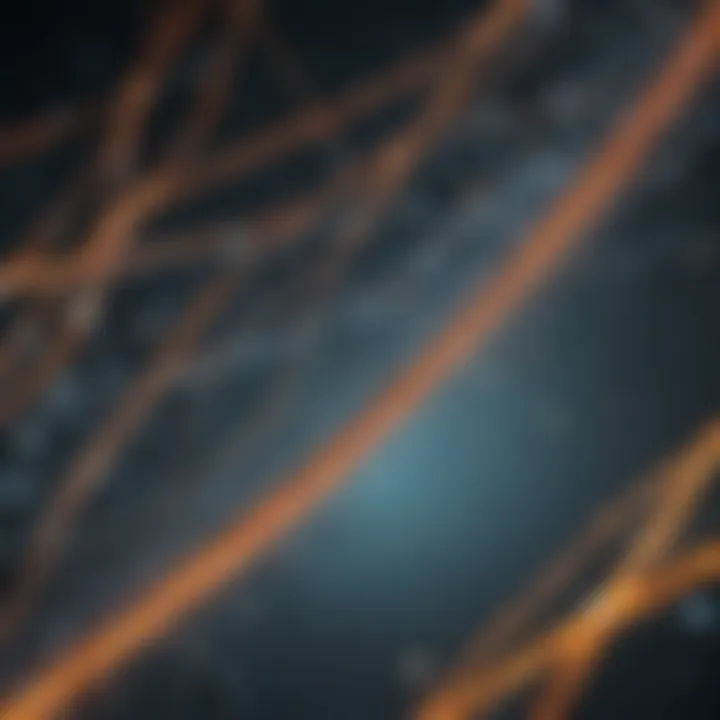
Cell communication is like the orchestra conductor of the human body. It's a finely tuned process that ensures all the different cell types work together harmoniously, maintaining the balance and functionality necessary for life. This concept encompasses numerous signaling mechanisms that dictate how cells interact, respond, and adapt to both internal stimuli and external environments. Understanding cell communication sheds light on how biological systems manage complex tasks and cope with challenges, making it crucial for students and professionals alike.
Cell Signaling Pathways
Hormonal Signals
Hormonal signals are the long-range messengers of the endocrine system. They travel through the bloodstream, delivering messages that influence growth, metabolism, and even mood. One key characteristic of hormonal signals is their ability to activate distant target cells, making them a powerful tool for coordinating bodily functions from a distance. Their beauty lies in their specificity; each hormone binds to particular receptors on target cells, triggering a cascade of responses tailored to the needs of the organism.
However, while hormonal signals are essential, they have a unique feature: the time lag in their action. Unlike neurotransmitters that work within milliseconds, hormonal responses may take minutes or even hours to manifest. This delayed response can be advantageous, allowing the body to maintain prolonged changes such as those seen during stress or growth phases.
Yet, this is not without its caveats. The dysregulation of hormonal signals can lead to various health issues, such as diabetes or thyroid disorders, where the communication process becomes faulty, highlighting the delicate balance required for optimal function.
Neurotransmitters
Neurotransmitters, on the other hand, are the swift messengers that communicate across synapses, the gaps between neurons. These molecules enable rapid responses to stimuli, crucial for functions such as reflexes and emotional reactions. Their ability to deliver messages in split seconds makes them particularly advantageous for processes that require immediacy, like responding to danger.
One notable feature of neurotransmitters is their incredible diversity. Various types of neurotransmitters exist, including dopamine, serotonin, and acetylcholine, each serving unique roles in nervous system function. However, this diversity can also pose a challenge, as imbalances or dysfunction in neurotransmitter systems are linked to a plethora of disorders, such as depression or schizophrenia.
Intercellular Communication
Gap Junctions
Gap junctions are specialized structures that facilitate direct communication between adjacent cells. These tiny channels allow for the passage of ions and small molecules, enabling cells to synchronize their activity. This direct form of communication is critical in tissues like the heart, where simultaneous contraction is essential for pumping blood effectively.
A striking advantage of gap junctions is their efficiency. By allowing cells to share resources and signals directly, they bypass the need for longer signaling pathways, ensuring a quick response. Additionally, their presence in various tissues illustrates their importance in maintaining metabolic couplings and coordinated activity.
However, gap junctions can also be a double-edged sword. In certain conditions, such as injury or disease, their closure can prevent communication and consequently impair tissue function. Thus, while they play a pivotal role, they need to function seamlessly to avoid unfavorable outcomes.
Chemical Signals
Chemical signals encompass a vast group of molecules including hormones, neurotransmitters, and other signaling compounds that orchestrate a symphony of cellular interactions. The hallmark of chemical signaling is its versatility; these signals can be employed for various purposes, from long-range hormonal communication to localized synaptic transmission.
One of the compelling aspects of chemical signals is the ability to convey intricate messages using combinations of signaling pathways. The sheer diversity of chemicals involved means that cells can respond to a multitude of signals simultaneously, enhancing their ability to adapt. However, this complexity can also lead to complications. For instance, a malfunction in the signaling pathway can disrupt normal processes, often resulting in diseases like cancer, where cell signaling is grossly aberrant.
Understanding cell communication provides insights into the delicate processes that sustain life and can reveal potential therapeutic avenues for addressing ailments related to dysfunctional signaling.
Cell Cycle and Division
The cell cycle and division are pivotal processes that enable the growth and maintenance of multicellular organisms. Understanding this intricate cycle not only sheds light on how cells replicate but also underscores the importance of regulation in cellular functions. The balance between cell proliferation and programmed cell death is crucial for homeostasis in the body. In this regard, any disruption in these processes can lead to significant health issues, including cancer.
Phases of the Cell Cycle
The cell cycle is divided into several distinct phases that allow for systematic division and reproduction of cells. Each phase plays a unique role in the overall lifecycle of a cell.
Interphase
Interphase comprises the majority of the cell's lifecycle, accounting for about 90% of the time. It’s where growth and preparation occur before cell division takes place. During this phase, a cell undergoes several critical processes: it grows in size, duplicates its DNA, and synthesizes the necessary proteins and organelles required for mitosis or meiosis.
A key characteristic of Interphase is its subdivided nature, encompassing G1 (Gap 1), S (Synthesis), and G2 (Gap 2) phases.
- G1 Phase: The cell increases in size and synthesizes mRNA and proteins necessary for DNA replication.
- S Phase: The cell replicates its DNA, ensuring each new cell will inherit a complete set.
- G2 Phase: The cell continues to grow and prepares for mitosis by producing proteins and organelles needed for the next phase.
What makes Interphase particularly beneficial is its focus on preparation; without a thorough and effective Interphase, subsequent stages of division may not proceed correctly, leading to potential errors. However, a downside is that it can be viewed as a lengthy process, potentially creating challenges in rapidly dividing tissues.
Mitosis and Meiosis
Mitosis and meiosis are crucial processes within the cell cycle that result in cell division, yet they serve very different purposes. Mitosis is the process of somatic cell division, resulting in two genetically identical daughter cells, crucial for growth, repair, and development.
On the other hand, meiosis is specialized for the production of gametes—sperms and eggs—halving the chromosome number to ensure diversity through sexual reproduction. One of the key characteristics of mitosis is that it involves a single division, while meiosis consists of two sequential divisions, leading to a reduction in chromosome number.
- Mitosis is beneficial as it allows for quick replication of cells, crucial for healing and growth. However, every now and then, errors during mitosis can lead to conditions like cancer.
- Meiosis, notably, introduces genetic variation through mechanisms like crossing over. Yet, its complexity means it is more prone to errors that can result in genetic abnormalities.
Regulation of the Cell Cycle
Regulating the cell cycle is essential for maintaining normal cellular function. Dysregulation can lead to uncontrolled cell growth, which is a hallmark of cancer.
Cyclins and CDKs
Cyclins and cyclin-dependent kinases (CDKs) are core players in the regulation of the cell cycle. Cyclins are proteins whose levels fluctuate throughout the cells' lifecycle, while CDKs are the enzymes that, when activated by cyclins, can phosphorylate other proteins to progress the cell cycle.
The key feature of Cyclins and CDKs lies in their ability to ensure the timing of cell cycle transitions is precise.
- Their benefit is that they create checkpoints needed to prevent erroneous divisions and maintain cellular integrity. However, if cyclin or CDK function is impaired, it can lead to unchecked progression and possibly tumorigenesis.
Checkpoint Mechanisms
Checkpoint mechanisms act as regulatory points in the cell cycle, ensuring that each phase has been properly completed before moving on to the next phase. They monitor DNA integrity, cell size, and whether DNA replication has occurred accurately.
A key characteristic of these checkpoints is their ability to halt the cycle if any discrepancies are found, allowing for repair or apoptosis.
- The primary advantage of checkpoint mechanisms is that they serve as guards against mutations and control the cell division process. While beneficial, a fault in such mechanisms can prevent cells from repairing themselves and may inadvertently lead to the proliferation of damaged cells.
In summary, the cell cycle and its regulation are fundamental to organismal health, influencing growth, repair, and reproduction. Understanding these processes provides insight into cellular biology and the potential for targeted therapies in conditions arising from cell cycle dysregulation.
Cellular Adaptation
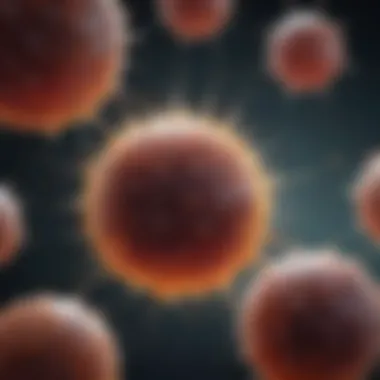
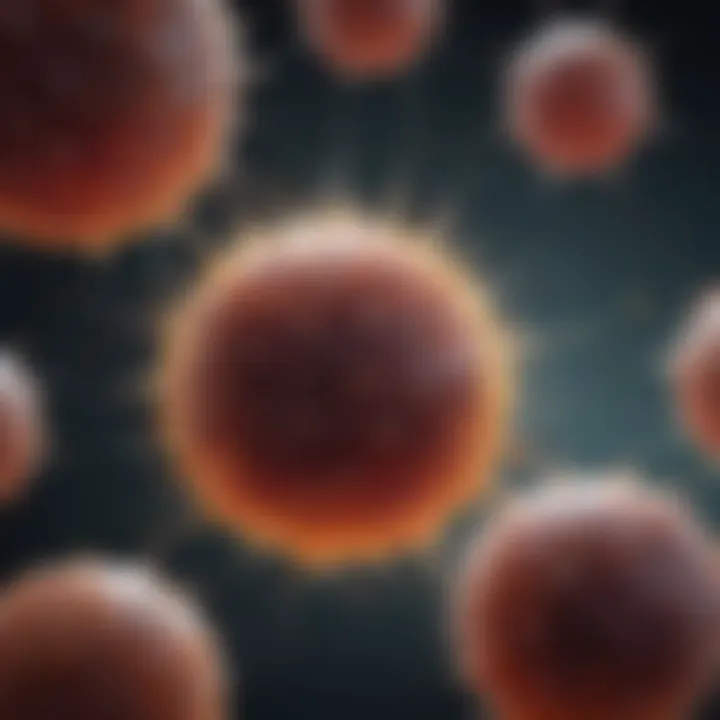
Cellular adaptation refers to the way cells adjust to various changes in their environment. This adaptability is crucial, as it allows cells to cope with stressors and maintain homeostasis, a balance within the body. Understanding how cells adapt is essential for comprehending their overall function and the implications for health and disease. Adaptations can be physiological, occurring in response to daily stresses, or pathological, which arise due to detrimental factors affecting cell functionality.
Physiological Adaptations
Physiological adaptations are the everyday adjustments that cells make to better serve the body’s needs. These changes are vital for survival, allowing organisms to thrive in diverse conditions. Let's take a closer look at these adaptations.
Response to Stress
When cells face stress—be it from limited resources, toxins, or physical damage—they must respond swiftly. The response to stress is characterized by a variety of mechanisms that enable cells to either repair damage or adjust their functions accordingly. For instance, when faced with oxidative stress, cells may ramp up the production of antioxidants to neutralize harmful free radicals. This adaptability is beneficial as it helps stave off cell death and maintain function. It acts like a safety net, allowing systems to remain operational in adverse situations.
While the unique feature of the response to stress is its dynamic nature, it can also have consequences—such as prolonged stress leading to maladaptation, which might cause more significant damage. Balancing act is key here; cells need to be responsive but should not overreact to stressors.
Metabolic Adjustments
Metabolic adjustments are another important physiological response of cells. These alterations can involve changes in energy production pathways or shifts in resource allocation. For example, muscle cells increase their reliance on anaerobic metabolism during intense exercise, even though this process is less efficient than aerobic metabolism. This shift is crucial for sustaining activity, ensuring the muscles get the energy they need in the short term.
The highlight of metabolic adjustments is their immediacy in addressing energy demands. However, such reliance on less efficient pathways can lead to an accumulation of metabolic byproducts that may affect cellular health in the long run. Hence, while metabolic adjustments are critical to functionality, they require careful regulation to prevent adverse effects.
Pathological Changes
As much as cells can adapt positively, there are also times when adaptations turn pathological, leading to dysfunction and disease. Pathological changes often signify a cellular response gone wrong, usually triggered by extreme or persistent stressors.
Cell Damage Mechanisms
Cell damage mechanisms reveal how stressors can lead to injury. Factors like toxins, radiation, or infection can inflict harm on cellular structures, leading to malfunctions. The body responds with repair mechanisms; however, these can become overwhelmed, resulting in apoptosis, or programmed cell death. One key characteristic of these mechanisms is the activation of stress response pathways, which, when not regulated, can escalate from temporary stress responses to full-blown damage.
Understanding cell damage mechanisms is beneficial as it can guide treatment strategies aimed at minimizing injury and promoting repair. On the downside, there is often a delay in these adaptive responses, which might lead to irreversible changes in cells.
Aging and Senescence
Aging and senescence encompass the gradual decline in cellular function over time. As cells divide and replicate, they experience cumulative damage from environmental factors, leading to a slowdown in their ability to respond and adapt. The hallmark of senescence is the loss of proliferative capacity, which means that aged cells can no longer divide and function optimally. This characteristic manifests in visible aging in an organism and correlates with increased susceptibility to diseases.
Aging and senescence highlight the trade-off between adaptive capacity and longevity—where adaptations to stress initially serve to prolong life, they can eventually lead to cellular exhaustion. It presents an interesting paradox that underscores the delicate balance cells maintain to sustain life while avoiding damage from wear and tear.
"Cells show us how life is not merely about enduring, but about adapting—every challenge met is part of staying alive."
Cellular Impact on Health
Cells serve as the foundation for all biological structures and functions in the human body. Their proper functioning is paramount for maintaining health and well-being. This section dives into how the behavior and characteristics of cells can directly affect overall health, particularly through their roles in diseases such as cancer and autoimmune disorders.
The health of an indivisual is closely linked to cellular health. When cells perform their functions optimally, they help to sustain the body, resist toxins, and heal injuries. However, when they go awry, the repercussions can be severe, leading to conditions that can alter or even end lives.
"The greatest threat to our health is the cell’s inability to communicate and function as intended."
Understanding these relationships between cell behavior and health outcomes offers insight into potential treatments and preventive measures.
Cancer Development
Mutations and Tumor Formation
Mutations in cellular DNA can lead to tumor formation, a crucial aspect of cancer development. These mutations can arise from various sources such as environmental factors, lifestyle choices, and even random errors during cell division. The key characteristic here is that not all mutations are harmful; in fact, some can be benign. However, specific mutations can disrupt normal cell function, leading to uncontrolled growth. This element makes it central to the discussion on cancer.
A palpable advantage of understanding the role of mutations is that it empowers researchers to target specific pathways in cancer treatment. For instance, targeted therapies can be developed to inhibit the functions of mutated proteins, potentially slowing or halting tumor progression. But there’s a double-edged sword to consider; while targeted therapy can be effective, it also may result in treatment resistance over time.
Metastasis
Metastasis is the process by which cancer spreads from its original site to other parts of the body. This aspect is exceedingly important in the overall cancer narrative. The key characteristic of metastasis is how deceptive it can be; a localized tumor might not raise immediate alarm, yet the potential for metastasis can pose a significant threat. It showcases cancer's ability to evolve and adapt, complicating treatment options considerably.
The unique feature of metastasis lies in its ability to utilize the body’s own systems to promote disease spread, such as the lymphatic system or bloodstream. However, while metastasis is often viewed as purely detrimental, studying this process has led to the development of new therapeutic approaches aimed at preventing the spread of cancer.
Autoimmune Disorders
Immune Response and Cells
The immune response is fundamentally about how cells in the body interact to defend against perceived threats. In autoimmune disorders, however, this finely-tuned system misfires, causing immune cells to attack the very tissues they normally protect. This characteristic presents a complex picture in the context of health, as it reveals how the immune system's cellular behavior can lead to harmful effects.
An essential element to consider is that while the immune response is a protective mechanism, its malfunction due to cells attacking the body can create profound health issues. This duality poses challenges in therapeutic interventions, as treatments must suppress the unwanted immune response without compromising overall immune function.
Examples of Autoimmune Diseases
A variety of autoimmune diseases highlight the complexities of immune responses gone awry. Conditions such as rheumatoid arthritis, lupus, and type 1 diabetes illustrate how cellular interactions can lead to debilitating health issues. Their key characteristic is the common thread of misdirected immune cells causing inflammation and damage in various organs.
In examining these diseases, one can see the unique feature of multiple. For example, rheumatoid arthritis can result in joint damage, whereas lupus can affect the skin, kidneys, and heart. While they may appear distinct, understanding the cellular bases for these diseases fosters a more holistic approach to treatment, allowing for tailored therapies that target specific cell types involved in the pathogenesis of each condition.
Ending
The integral role of cells in the human body cannot be overstated. This article has illuminated the myriad of ways cells contribute not only to individual health but also to the overall functioning of bodily systems. For students, researchers, educators, and professionals, understanding these concepts is essential in framing the complex narrative of cellular biology. By delving into the diversity of cell types, their structures, and functions, it becomes clear that every cell is a cog in the machine that sustains life.
Summary of Key Points
- Cell Types: Cells are categorized primarily into eukaryotic and prokaryotic, with somatic and germ cells playing unique roles in physiological processes.
- Cell Structure and Function: The intricate organization within cells, from membranes to organelles, underpins their roles in energy production and protein synthesis.
- Cell Communication: Cell signaling pathways and intercellular interactions are vital for maintaining homeostasis and responding to environmental changes.
- Cell Cycle and Adaptation: Understanding how cells adapt to stresses, undergo division, and respond to damage is fundamental in contexts such as cancer biology and aging.
- Health Implications: The article outlined how cellular behavior significantly influences disease processes like cancer development and autoimmune disorders.
Future Directions in Cell Research
Potential Therapeutic Approaches
Potential therapeutic approaches in cellular biology are gaining traction for their promise in treating a variety of conditions. Techniques like gene therapy aim to correct genetic disorders by directly modifying cellular components. This approach has gained considerable attention because it addresses the root cause of diseases rather than just symptoms. A key feature of these therapies is their ability to potentially result in long-lasting effects, restoring function at the cellular level. However, challenges remain, such as ensuring precise delivery of the therapeutic agents without causing unintended consequences.
Technological Advancements
Advancements in technology are reshaping the landscape of cellular research. Tools such as CRISPR-Cas9 enable precise editing of DNA within cells, facilitating groundbreaking research in genetic engineering. The central feature of these advancements is their ability to provide scientists with unprecedented control over cellular mechanisms, allowing for the development of targeted therapies. Nonetheless, the ethical considerations and potential for misuse necessitate careful scrutiny to ensure the responsible use of these technologies.
"In the realm of cellular biology, the possibilities are as vast as the complexities within each cell."