CsI Scintillators: Impact on Science and Innovation
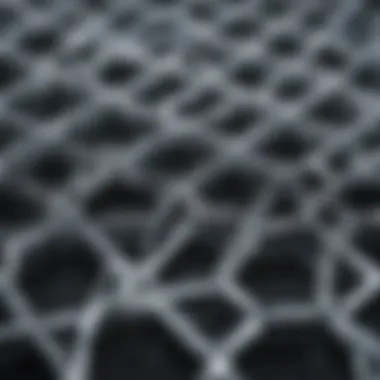
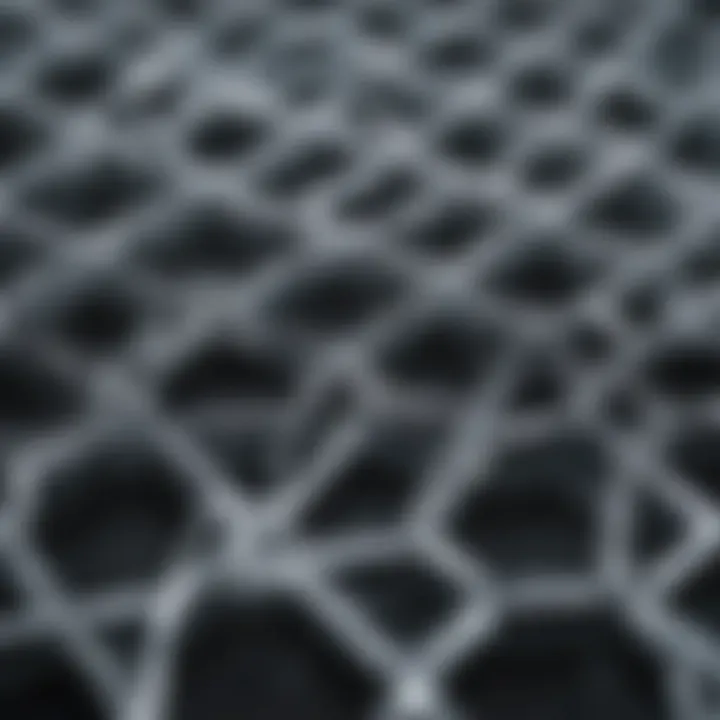
Intro
In the realm of modern science, materials play a pivotal role in shaping our understanding of the universe. Among these materials, cesium iodide (CsI) scintillators stand out for their remarkable properties and wide-ranging applications. These scintillators, which convert high-energy radiation into visible light, have become cornerstone elements in fields such as radiation detection, medical imaging, and experimental physics. Their versatility is immense, making them ideal for a variety of uses—from monitoring radiation levels in nuclear facilities to enhancing the clarity of images in medical diagnostics.
This article aims to delve into the intricate workings and diverse applications of CsI scintillators, shedding light on their structure and operational principles. By exploring both historical context and recent advancements in scintillator technology, we can adequately gauge the significance of cesium iodide in contemporary research and its implications for future innovations.
The discussion will be centered around key topics including the essential characteristics of CsI, how they function within various scientific paradigms, and noteworthy progress in the field that pushes boundaries forward. Ultimately, we seek to furnish a comprehensive understanding that caters to students, researchers, educators, and professionals alike, presenting both foundational knowledge and cutting-edge insights that are crucial in today's scientific landscape.
Now, let’s transition into understanding how the methodology applies to the exploration of CsI scintillators.
Preamble to CsI Scintillators
CsI scintillators, particularly cesium iodide, play a significant role in modern scientific fields such as radiation detection, medical imaging, and experimental physics. Their importance lies not just in their ability to detect radiation, but also in how they enhance imaging techniques and provide data that are crucial for various applications. Understanding CsI scintillators is essential for advancing technology, particularly in areas that rely heavily on precise measurements and high-quality imaging.
Definition and Composition
Cesium iodide (CsI) is a compound made up of cesium cations and iodide anions. This unique combination gives CsI its scintillation properties, whereby it is capable of converting high-energy radiation into visible light. The crystalline form of CsI provides a solid-state solution, making it stable and efficient in detecting different forms of radiation. What sets CsI apart from other scintillators is its relatively simple composition and high atomic number, which contributes to its effectiveness in capturing gamma rays and X-rays.
In practical terms, imagine needing to capture a fleeting moment of high-energy radiation. CsI scintillators can do this by emitting light almost immediately after absorbing that radiation. This immediate response makes them especially valuable for scientific research where timely data collection matters. The efficiency of cesium iodide translates into sharper images in medical applications, such as in CT scans and PET scans, where precision is vital.
Historical Context
The advent of scintillation detectors in the early to mid-20th century marked a pivotal development in science. The use of organic and inorganic scintillators paved the way for advancements in numerous fields. CsI entered the spotlight primarily during the 1950s, as researchers sought more efficient materials for gamma-ray detection. It didn’t take long for cesium iodide to establish its place because it offered advantages in terms of light yield and energy resolution compared to its contemporaries.
Throughout the decades, innovations in material science have fostered a deeper understanding of CsI's capabilities. Researchers have explored different fabrication methods, enhancements to the crystal structure, and doping techniques to improve light emission. This journey from a basic compound to a sophisticated detector mirrors the evolution of technology itself, where each step brings about improvements that serve a greater purpose. Today, CsI scintillators are a fundamental component in many technological advances across scientific disciplines.
"The history of CsI scintillators is more than just dates and facts—it's about the evolution of technology that shapes our understanding of the world around us, providing insights that were once beyond our reach."
In summary, integrating the definition and historical context of CsI scintillators lays the groundwork for understanding their significance in the landscape of modern science. As the article unfolds, it will delve deeper into their physical properties, mechanisms, and varied applications, embellishing the narrative of this crucial material.
Physical Properties of CsI
The physical properties of cesium iodide (CsI) are fundamental to understanding its application in various scientific fields. The unique characteristics of this substance—ranging from its crystalline structure to its thermal attributes—foster its use in radiation detection and imaging technologies. When assessing the efficacy and reliability of scintillators, these properties play a pivotal role, making it imperative to evaluate them thoroughly. Let’s dive into the core aspects.
Crystal Structure
CsI crystallizes in a body-centered cubic lattice, characterized by its high symmetry and distinct arrangement of atoms. This regular structure not only contributes to its high effective atomic number, but also influences its interaction with radiation. The highly ordered arrangement allows for efficient photon transport and energy pooling. Due to the inherent properties of its crystal lattice, CsI offers increased light yield compared to other scintillating materials, making it an exceptional choice in high-energy physics experiments.
"The crystal structure of CsI is ideal for manipulating light and energy, further positioning it at the forefront of scintillation technology."
Moreover, defects or irregularities in this structure can impact the scintillator’s performance. Scientists rely on meticulous synthesis techniques to promote optimal crystal growth and minimize the chances of defects. A better understanding of the crystal characteristics at the atomic level leads to developments in material fabrication, pushing the limits of its applications.
Optical Characteristics
The optical attributes of CsI significantly influence its performance in detecting and imaging radiation. It exhibits notable transparency to its own emitted light, which is emitted within the visible spectrum, usually peaking around 550 nm. This wavelength corresponds with the sensitivity range of many photodetectors, making CsI a popular choice in scintillation detectors.
Additionally, the interaction between incident radiation and CsI leads to efficient light production. This optical performance is assessed through parameters such as light yield, decay time, and emission spectrum. CsI typically shows high light yields—upwards of 50,000 photons per MeV—exceeding many other scintillators. Decay times in the order of hundreds of nanoseconds allow for faster signal processing, suited for both medical imaging applications, such as in positron emission tomography (PET), and in high-energy physics environments.
The optical properties are also affected by temperature and composition. Altering the ratio of cesium to iodine can tailor the scintillator's response to particular environments, potentially enhancing performance for specialized applications. Hence, a thorough understanding of these characteristics can offer vast opportunities for innovation in the design of scintillator materials.
Thermal Conductivity and Stability
Thermal conductivity is a key factor that can not be overlooked when considering scintillator materials. CsI demonstrates good thermal conductivity, which is vital for handling heat generated during high-energy interactions. Improved thermal management directly relates to overall device performance and stability during prolonged operation. Failing to account for thermal aspects can lead not only to degraded functionality but also to irreversible damage in detecting systems.
In terms of stability, CsI remains relatively resilient against various external factors, such as humidity and radiation damage. Its durability is further enhanced through the use of protective coatings and materials that can mitigate environmental degradation. However, prolonged exposure to moisture can lead to hygroscopic effects, which may compromise crystal integrity.
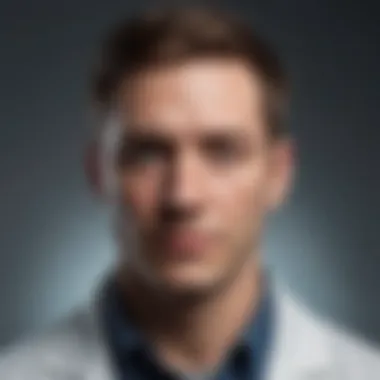
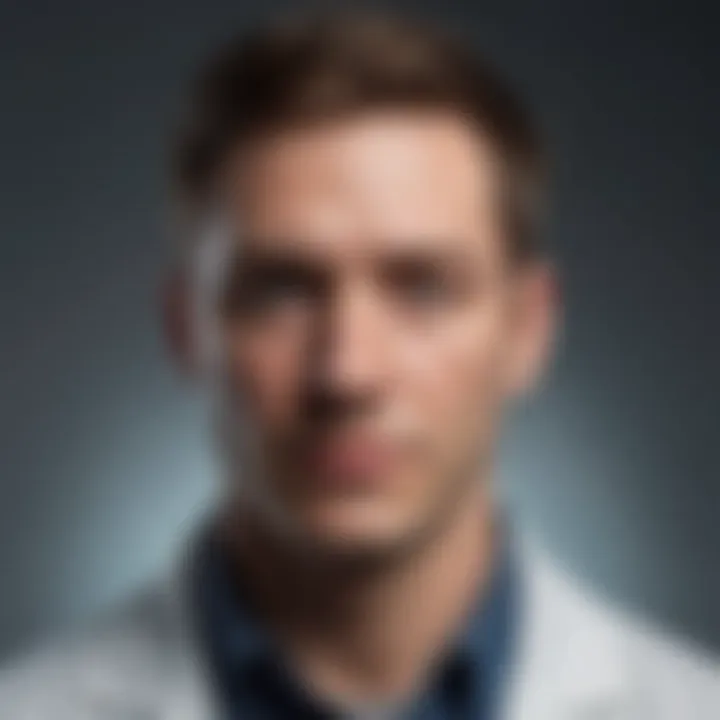
Understanding thermal conductivity and stability alongside other physical properties plays an integral part in the development of scintillator-based technologies. This knowledge helps researchers to strategize more effective methodologies for integrating CsI into systems, ensuring they function optimally across varying conditions.
Mechanism of Scintillation
Understanding the mechanism of scintillation is fundamental to grasping how CsI scintillators operate within various scientific fields. This topic not only sheds light on how these materials interact with radiation but also illustrates their utility in real-world applications such as nuclear physics and medical imaging. The processes taking place at the atomic level pave the way for the effective detection and measurement of ionizing radiation, ultimately benefiting sectors such as healthcare and environmental monitoring.
Energy Absorption Process
The energy absorption process in CsI scintillators revolves around the interaction of incoming radiation with the material’s atomic structure. When high-energy photons strike the CsI crystal, they transfer their energy to the electrons within the crystal lattice, exciting them to higher energy levels. This can be seen as akin to a game of billiards; the incoming ball (radiation) hits another ball (electron), causing it to move and rebound into a new state.
Once the electrons reach their excited state, they can either return to the ground state, losing energy in the form of heat, or they can participate in further processes. Specifically, in CsI, a process known as electron-hole pair generation occurs.
- Ionization Impact: The energy from the radiation creates electron-hole pairs as the crystal’s arrangement permits relatively mobile electrons to escape from their core positions.
- Excitation Level: These excited electrons may stay in a higher energy state for a fraction of a second before bouncing back down, but not without leaving a trace of energy behind.
Overall, it’s these excited states that ultimately trigger the scintillation light when the electrons return to their lower energy levels.
Light Emission Phenomena
Once electrons have jumped back to their more stable configurations, scintillation light is emitted. This light emission phenomenon is critical; it is what allows the detectors to identify and analyze radiation. In CsI crystals, this occurs through a mechanism called radiative recombination. As electrons recombine with holes, they release photon energy in the visible spectrum, primarily in the blue-green range. This is where the magic truly happens.
- Efficiency of Photons: High efficiency in photon production gives CsI its edge in various applications. A well-designed CsI scintillator can emit a significant number of visible light photons per keV of x-ray energy absorbed. This can be likened to a well-balanced engine that generates just the right amount of power; excess energy translates into more detectable light.
- Scintillation Timing: The speed of this light emission also plays a key role in applications requiring rapid measurements, such as in medical diagnostics or radiation monitoring. The shorter the time between energy absorption and light emission, the more effective the scintillator is in these fast-paced environments.
"The mechanism of scintillation in CsI plays a pivotal role in applications ranging from basic research to life-saving medical technologies. Understanding this mechanism is crucial to maximize the potential benefits of scintillator technology."
In summary, the mechanism of scintillation encapsulates both the energy absorption and the light emission processes, crucial for harnessing the power of cesium iodide in a variety of modern scientific applications. Its intricacies not only enhance detection capabilities but also have profound implications for future innovations in the fields of radiation detection and imaging.
Applications of CsI Scintillators
The applications of CsI scintillators stretch far beyond mere technical curiosity. Their significance emerges strongly in fields such as radiation detection and medical imaging, positioning them as essential tools in both research and practical scenarios. With the ability to effectively convert high-energy radiation into visible light, CsI scintillators have become a go-to option for scientists and professionals alike, ensuring that they maintain a leading edge in various applications. The reliability and robustness of cesium iodide crystals underpin their pivotal role in advancing technology in health and physics.
Radiation Detection
Radiation detection serves as one of the most critical applications of CsI scintillators. In environments where ionizing radiation is prevalent, such as nuclear facilities or hospitals with radiological equipment, having a dependable detection method can mean the difference between safety and hazard. CsI scintillators excel in identifying gamma rays and other radiative elements due to their high atomic number and density. This allows them to interact effectively with high-energy photons, producing detectable light signals that indicate the presence of radiation.
Moreover, CsI scintillators are used in portable devices designed for radiation monitoring. These devices benefit from the materials' compactness and lightweight nature. The integration of CsI in personal dosimeters affirms their practicality in everyday settings, helping ensure that radiation exposure remains within safe limits.
Medical Imaging Techniques
Medical imaging techniques, particularly positron emission tomography (PET) scans and single-photon emission computed tomography (SPECT) imaging, have greatly benefited from the use of CsI scintillators. This segment of applications highlights both the technological prowess and versatility of cesium iodide crystals in diagnosing health conditions.
Use in PET Scans
In the realm of PET scans, CsI scintillators play a vital part in enhancing image quality. The fundamental aspect of PET involves the detection of gamma rays emitted during a radioactive decay process. CsI scintillators provide excellent light output in response to these high-energy interactions, helping to produce clearer images. The high detection efficiency coupled with a fast response time makes them a favored choice for this imaging technique.
The standout feature of CsI in PET scans lies in its ability to maintain performance under high radiation load, which is key during dynamic studies of metabolic activities. However, while they shine in terms of light yield and efficiency, practitioners must consider their relatively higher cost compared to other scintillation materials, which could be a limiting factor for widespread adoption in certain facilities.
Use in SPECT Imaging
SPECT imaging, like its PET counterpart, also relies on detecting gamma radiation. Here, CsI scintillators have proven valuable for their energy resolution and spatial resolution capabilities. The primary function of CsI crystals in SPECT is to convert incoming gamma photons into visible light, which is then captured by photodetectors.
The appealing characteristic of CsI for SPECT is its durability and high resolution, which allows for more detailed imaging of physiological processes within the body. However, as with PET, a drawback exists; their performance can vary under prolonged use, with some degradation affecting light output over time. Thus, understanding the balance between their benefits and limitations is crucial for healthcare professionals when selecting scintillation materials for specific imaging tasks.
Applications in High-Energy Physics
High-energy physics relies heavily on scintillator materials, with CsI scintillators taking center stage. From particle accelerators to astrophysical observations, these materials enable researchers to detect and analyze fundamental particles and cosmic rays. The high detection efficiency coupled with solid structural integrity under extreme conditions makes CsI invaluable for experiments that demand precision and reliability. The ongoing advancements and innovations in the application of CsI scintillators are likely to continue opening new avenues in both research and practical applications, establishing the material's relevance well into the future.
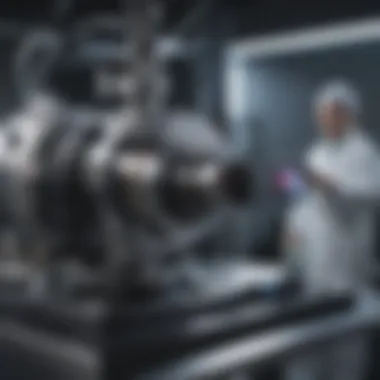
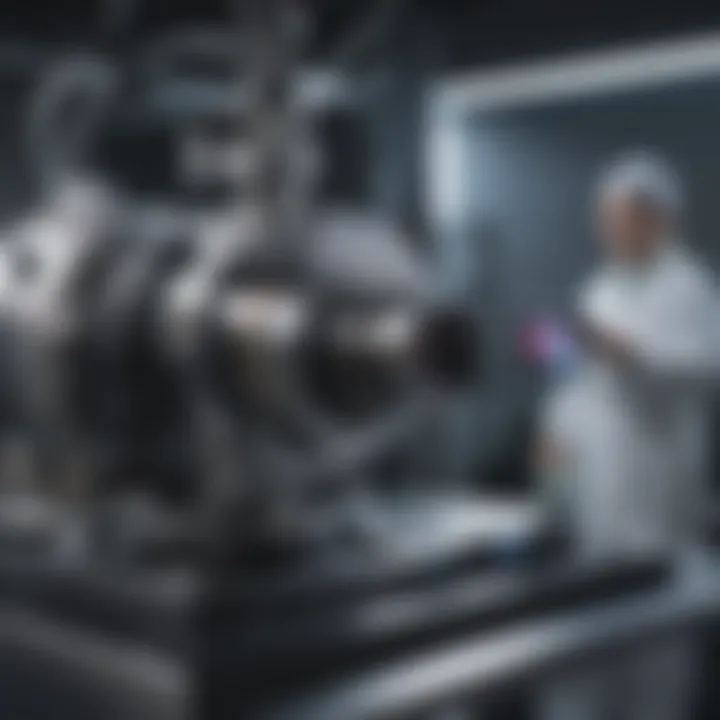
Advantages of Using CsI
CsI scintillators have carved out a significant place in various scientific applications due to their unique properties. Understanding the advantages of using cesium iodide (CsI) can help researchers and professionals appreciate why these materials are preferred in monitoring and detecting radiation, as well as in medical imaging techniques. Let's delve into some specific elements that shine a light on the benefits of CsI scintillators.
High Detection Efficiency
The high detection efficiency of CsI scintillators is one of the standout features that make them indispensable in various fields. Detection efficiency refers to the ability of a scintillator to convert incoming radiation into a detectable signal of light effectively. CsI scintillators achieve this with remarkable proficiency.
One of the reasons for this efficiency is the material's atomic composition. Cesium and iodine atoms have large cross-sections for absorbing gamma rays, which means they effectively capture high-energy photons and convert them to scintillation light. This leads to a greater yield of visible light when radiation interacts with the detector.
Moreover, when compared to other materials, CsI scintillators provide a broader range of energy response, accommodating both low-energy and high-energy photons. This versatility makes them suitable for various applications, such as:
- Medical imaging technologies, including PET and SPECT scans
- Radiation monitoring in nuclear facilities
- Experimental setups in particle physics
In light of these capabilities, researchers often prefer CsI over other scintillators, as it can provide higher sensitivity and accuracy in detecting radiation.
Robustness and Stability
Another critical advantage of CsI scintillators is their robustness and stability, which allow them to operate effectively in diverse and sometimes harsh environments. Unlike some other scintillation materials that may degrade or lose performance when subjected to radiation or fluctuating temperatures, CsI maintains its integrity under a variety of conditions.
The crystal structure of CsI is intrinsically stable. It shows excellent performance over extended periods without significant degradation. This stability not only enhances the longevity of the scintillator but also ensures that the measurements remain accurate and reliable over time. Furthermore, CsI scintillators are less susceptible to humidity and moisture than other types, a trait that is especially advantageous in fluctuating environmental conditions.
As a result of this durability, users can expect:
- Reduced need for frequent calibrations
- Longer lifespan for scintillation devices
- Fewer unexpected failures in critical applications
These properties make CsI scintillators ideal candidates for both laboratory and field applications, where reliability is of utmost importance.
The combination of high detection efficiency and robust stability positions CsI scintillators at the forefront of radiation detection technologies, making them a favorite among professionals in the scientific community.
Challenges in CsI Utilization
Identifying the hurdles in the use of CsI scintillators is essential for a complete understanding of their role in modern science. These challenges impact not only the performance of the materials but also their feasibility in various application fields. When considering implantation for radiation detection, medical imaging, or even in experimental physics, it's crucial to understand the specific drawbacks, so informed conclusions can be made. Not everything shines bright in the world of scintillators, and addressing these limitations provides a more comprehensive picture of their capabilities.
Material Limitations
When we talk about material limitations in CsI scintillators, several factors need to be examined closely. Firstly, the intrinsic properties of cesium iodide itself present both advantages and disadvantages. CsI has a relatively high atomic number, which makes it suitable for detecting a range of radiation types. However, its hygroscopic nature poses risks. This means that CsI can absorb moisture from the environment, leading to degradation of the scintillator’s performance. Hence, special packaging or handling precautions are often necessary, which adds complexity to its usage.
Secondly, the crystalline structure of CsI, while useful in many contexts, also limits its sensitivity at lower energy levels. In practical terms, this means that CsI scintillators might not effectively pick up very low energy gamma rays or X-rays. As technologies advance and the need for ways to detect weaker signals grows, this limitation becomes increasingly notable.
"Material considerations are a balancing act; the benefits often come with noteworthy caveats that mustn't be brushed aside."
Lastly, there's an ongoing conversation in material science regarding the toxicity and safety of elements used in scintillators. CsI's composition raises some eyebrows, particularly considering potential toxicological implications if mishandled or improperly disposed of. The quest for safer materials, possibly through the development of alternative formulations, is a hot topic among researchers.
Cost Considerations
Cost considerations serve as a significant hurdle when assessing the widespread deployment of CsI scintillators in various fields. The manufacturing process for CsI crystals can be quite expensive, particularly when quality control measures ensure that the scintillators meet the high standards necessary for sensitive scientific applications. This price tag limits options for larger installations or widespread utility where budget restrictions are a major factor.
Additionally, the costs don't stop at initial procurement. There are ongoing expenses related to maintenance and operation. Due to the need for careful handling—thanks to its sensitivity and hygroscopic nature—extra resources must be allocated for the upkeep of these materials, potentially driving up operational costs over time.
Another point worth noting is market fluctuations. The materials market can be unpredictable, often influenced by geopolitical factors. The eventual rise in the cost of rare materials can drastically impact the entire supply chain, complicating things for researchers and companies alike.
Recent Advancements in Scintillator Technology
Recent advancements in scintillator technology play a crucial role in the field of modern science, particularly as the demands for precision in radiation detection and imaging continue to escalate. In the last few years, researchers have earmarked novel materials and innovative formulations that significantly enhance the performance of cesium iodide (CsI) scintillators. The relevance of these advancements not only caters to the existing applications but also opens new avenues for Research and Development (R&D) initiatives.
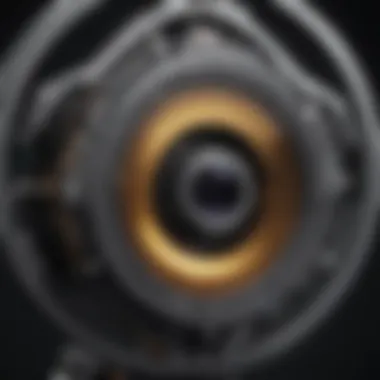
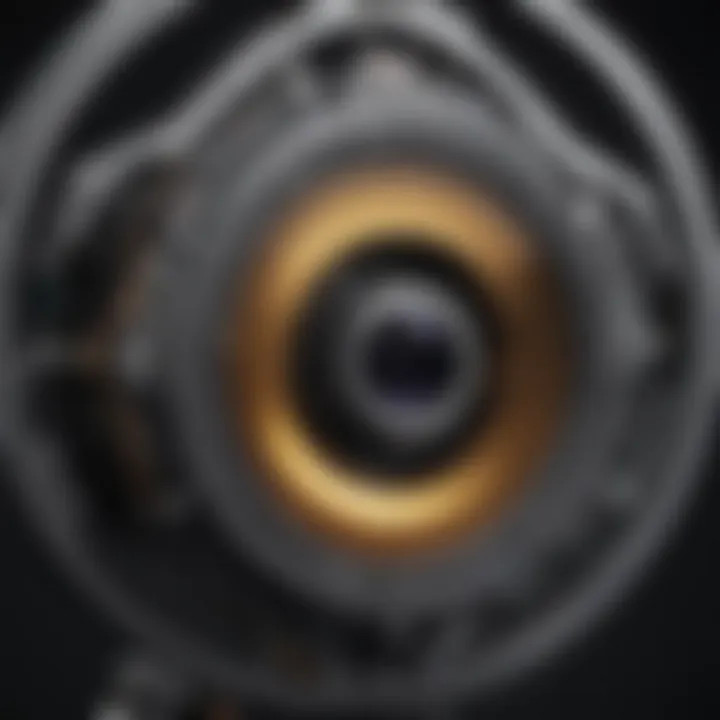
Innovative Materials and Formulations
One of the key shifts in scintillator technology is the exploration of innovative materials that promise higher efficiency and better light output. Traditionally, CsI has been the material of choice, but recent investigations into various doping elements have yielded promising results. For instance, the inclusion of thallium or sodium in CsI crystals has been shown to improve the light yield, making the scintillator more sensitive to incoming radiation.
In addition to conventional dopants, researchers are experimenting with composite materials that incorporate other scintillation crystals, such as LSO (lutetium oxyorthosilicate) or GSO (gadolinium oxyorthosilicate). This blend offers a tactical approach to enhance the scintillation properties by capitalizing on the best attributes of each material.
Moreover, advancements in fabrication technologies, such as improved crystal growth techniques, facilitate the production of higher-purity scintillators. This results in reduced defects within the crystal lattice, leading to superior performance, particularly in environments subjected to high radiation levels. As these materials gain traction, they pave the way for new applications in fields such as nuclear medicine and high-energy physics.
Enhanced Light Yield Techniques
Light yield is a determining factor in the efficacy of scintillators. Efforts to boost this parameter have led to enhanced light yield techniques recently emerging on the horizon. One notable approach is the optimization of crystal size and geometry. Tailoring the dimensions of CsI crystals has shown to amplify the light output. The clever design of these crystals ensures that emitted light is effectively coupled to photodetectors.
Another area of innovation encompasses the surface treatment of scintillator materials. Techniques such as coating with reflective or luminescent materials can trap emitted photons, leading to an increase in detected signals. As part of this trend, researchers are also exploring alternative ways to utilize nanomaterials to work alongside CsI scintillators. These protocols could provide additional avenues for capturing and enhancing emitted light, potentially revolutionizing performance metrics in various applications.
"Innovations in materials and light yield techniques enable scintillators to meet ever-evolving scientific demands, transforming them into indispensable tools in modern research."
Overall, the ongoing advancements in scintillator technology, particularly in the realm of innovative materials and enhanced light yield techniques, illuminate new possibilities for CsI applications. These strides not only contribute to handling complex scientific challenges but also underscore the importance of continued investment in research for further breakthroughs.
Future Trends and Research Directions
The field of CsI scintillators is continually evolving. As researchers dig deeper into the properties and potential applications of cesium iodide, it becomes clear that there are several promising trends on the horizon. Understanding these future directions is vital as they could play a significant role in enhancing the performance of scintillators in various domains.
Potential New Applications
The versatility of CsI scintillators leads us to speculate about potential new applications. Imagine their integration in emerging technologies such as portable radiation detectors used in homeland security or emergency response scenarios. As technology advances, the aim would be to develop thinner and lighter scintillation detectors without sacrificing sensitivity. This would allow for more effective field applications, making it easier for professionals to conduct non-invasive assessments in hazardous environments.
Another exciting potential application could lie in environmental monitoring. CsI scintillators could be employed in collecting data on background radiation in urban settings or near nuclear facilities, providing datasets for researchers focused on environmental safety.
Moreover, considering their effectiveness in medical imaging, there is a possibility that new formulations or hybrid materials using CsI could enhance imaging quality in MRI techniques, allowing for sharper image resolutions and potentially uncovering conditions that go undetected today. It’s about pushing the envelope to new frontiers, making use of their inherent properties in ways we have yet to explore.
Sustainability in Material Sourcing
Sustainability is a critical consideration, especially as environmental issues loom larger in today’s world. The sourcing of materials for CsI scintillators must be scrutinized to ensure that the development and usage processes minimize environmental impact. Striking a balance between high-performance materials and eco-friendliness is not just good practice but a necessity.
Efforts to refine the extraction of cesium and iodine should focus on reducing waste and energy consumption. Technological innovations could pave the way to better methods, potentially utilizing recycled materials where applicable. In addition, exploring alternative materials that can mimic or enhance scintillation properties without relying heavily on rare earth elements might also yield significant benefits.
It’s important to highlight that by pushing for more sustainable processes in producing CsI scintillators, we not only protect the environment but also reduce costs. As the pressure mounts from both consumers and regulatory bodies, a move toward sustainability could define the next era of scintillator development, ensuring that the field grows responsibly while meeting the demands of modern science.
"Innovation in material sourcing is not only a trend but a necessity that impacts the credibility and acceptance of scientific advancements in the long run."
Culmination
The conclusion serves as a crucial element in the comprehensive exploration of CsI scintillators, emphasizing not only the significance of cesium iodide in various scientific applications but also the future trajectories for research and development. In summarizing the key findings, it reflects on the pivotal role CsI plays in enhancing the effectiveness of radiation detection technologies, advancing medical imaging, and facilitating precise measurements in high-energy physics. Each application highlights how these materials have transformed scientific endeavors, ensuring clearer results and improving the efficiency of detection methodologies.
"The advancements in scintillator technology are not just incremental; they redefine the benchmarks for performance in essential scientific fields."
With regards to the implications for future research, this conclusion outlines beneficial considerations as specialists continue to innovate. It points out the ongoing research dedicated to enhancing the properties of CsI, such as improving light yield and developing new formulations that may offer increased sensitivity and reliability. Future studies might also explore sustainability aspects, particularly in sourcing materials used in the fabrication of these scintillators, aiming to balance technological advancement with environmental responsibility.
Ultimately, the understanding and application of CsI scintillators not only serve current scientific needs but also pave the way for surprises that lie ahead in diverse fields. As the scientific community endeavors to uncover new applications and improve existing technologies, the focus on CsI scintillators remains pivotal, marking them as a cornerstone in the fabric of modern scientific inquiry.
Summary of Key Findings
CsI scintillators have proven to be invaluable across a range of disciplines. Here are some key takeaways:
- High Detection Efficiency: The high atomic number of cesium and iodine contributes to exceptional radiation detection capabilities.
- Versatility in Applications: From medical imaging techniques like PET and SPECT to high-energy physics experiments, CsI has shown broad relevance and applicability.
- Advancements in Technology: Recent innovations include enhanced light yield techniques, which promise to elevate the functionality of scintillators even further.
- Material Considerations: Challenges such as material limitations and cost can influence the adoption of CsI scintillators, but ongoing research provides pathways for overcoming these issues.
Implications for Future Research
Future research directions in the field of CsI scintillators should focus on:
- Innovative Material Development: Exploring new formulations that may yield better performance or be more cost-effective.
- Sustainability Initiatives: Reducing environmental impact by seeking alternative or recycled materials for scintillator production.
- Expanded Applications: Investigating potential uses in fields like astrophysics, environmental monitoring, and security.
- Interdisciplinary Collaboration: Engaging with researchers from various fields to enhance the knowledge base and application possibilities of CsI materials.
By understanding these aspects, the scientific community stands on the cusp of significant advancements, ensuring that CsI scintillators remain at the forefront of technology conducive to future exploration and discovery.