Innovative Insights into Copper Oxide Nanoparticles
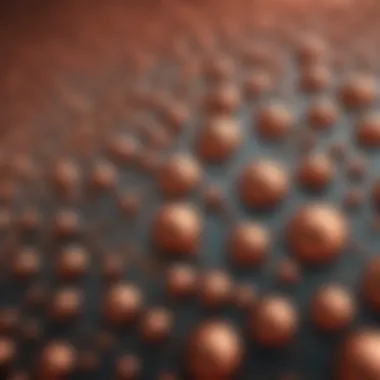
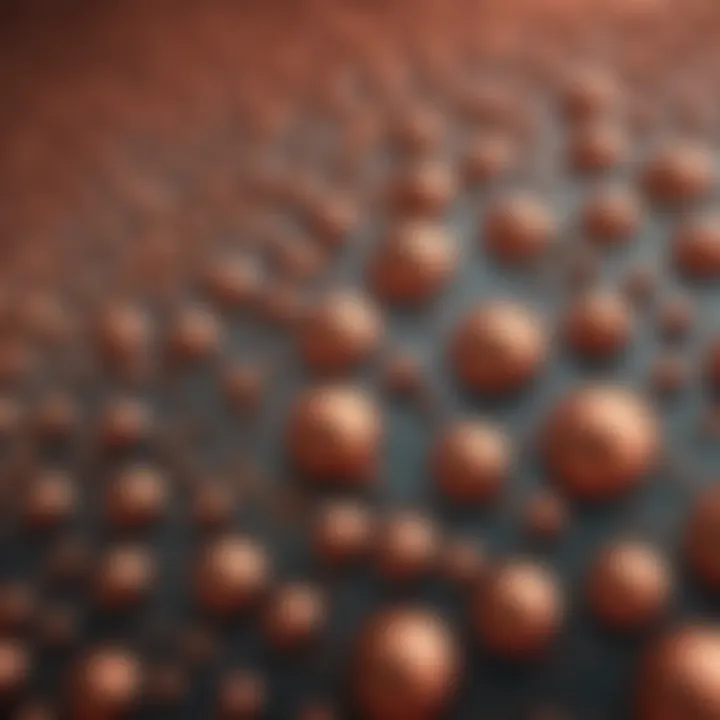
Intro
Copper oxide nanoparticles (CuO NPs) have recently gained considerable interest in various scientific fields for their unique properties and potential applications. These nanoparticles exhibit remarkable qualities, including high conductivity, catalytic activity, and antibacterial effects, making them suitable for diverse applications ranging from medicine to electronics and environmental science. This article provides an exploration of their synthesis methods, distinct physical and chemical characteristics, and the ongoing research aimed at harnessing their full potential.
Methodology
Study Design
The study of copper oxide nanoparticles incorporates a multi-faceted approach, combining experimental analysis with theoretical frameworks. This design focuses on understanding the synthesis methods as well as the properties and applications of these nanoparticles. Different techniques, including chemical, physical, and biological methods, are evaluated to determine their efficiency and effectiveness.
Data Collection Techniques
Data collection for the research involves a combination of quantitative and qualitative techniques. Primary methods include laboratory synthesis experiments, characterization of nanoparticles using techniques such as Transmission Electron Microscopy (TEM) and X-ray Diffraction (XRD). Additionally, literature reviews of existing studies provide a deep insight into prior findings and pave the way for future research.
Properties of Copper Oxide Nanoparticles
Copper oxide nanoparticles exhibit several key properties that contribute to their functionality:
- Size and Morphology: The size of CuO NPs typically ranges from 1 to 100 nm, influencing their reactivity and interactions.
- Optical Properties: These nanoparticles demonstrate distinctive optical characteristics, including UV absorption, which is crucial for applications in photodetection.
- Electrical Conductivity: Their ability to conduct electricity makes them ideal for electronic applications.
- Catalytic Activity: CuO nanoparticles have shown promising results in catalyzing various reactions, enhancing efficiency in chemical processes.
Applications
Copper oxide nanoparticles are utilized in multiple fields, highlighting their versatility:
- Medicine: Due to their antibacterial properties, they are being explored for use in drug delivery systems and antimicrobial coatings.
- Electronics: Their conductive nature renders them suitable for use in sensors, photovoltaic cells, and batteries.
- Environmental Science: CuO nanoparticles are increasingly noted for their potential in wastewater treatment and environmental remediation.
"The unique properties of copper oxide nanoparticles underscore their importance in future technological advancements."
Discussion
Interpretation of Results
Research indicates that the synthesis method significantly influences the properties and applications of copper oxide nanoparticles. Green synthesis methods, for instance, provide eco-friendly alternatives producing nanoparticles with desirable characteristics.
Limitations of the Study
Among the limitations noted is the variability in the characterization techniques applied across different studies. Discrepancies may arise, leading to challenges in synthesizing uniform copper oxide nanoparticles.
Future Research Directions
Future inquiries should focus on refining synthesis methods to improve consistency and exploring new applications, particularly in sustainable technologies. Investigating the interactions of CuO NPs with biological systems will also be crucial in advancing their medical applications.
By delving into the properties, synthesis methods, and potential applications of copper oxide nanoparticles, this article aims to illuminate the path forward for researchers and professionals engaged in nanotechnology.
Preface to Copper Oxide Nanoparticles
Copper oxide nanoparticles play a crucial role in various fields such as medicine, electronics, and environmental science. Their unique properties make them a point of interest for researchers and professionals alike. Understanding copper oxide nanoparticles paves the way for innovative applications, which can help address global challenges like pollution and disease. This section aims to provide a comprehensive overview of copper oxide nanoparticles, highlighting their definition, composition, and historical development.
Definition and Composition
Copper oxide nanoparticles are nanomaterials consisting primarily of copper and oxygen. They are classified into two distinct forms: cuprous oxide (Cu2O) and cupric oxide (CuO). The difference lies in their oxidation states, with cuprous oxide containing copper in a +1 oxidation state and cupric oxide featuring it in a +2 state. Both forms exhibit unique characteristics and behaviors that influence their application potential.
The size of these nanoparticles typically ranges from 1 to 100 nanometers, allowing them to showcase unique physical and chemical properties due to their significantly high surface area to volume ratio. At the nanoscale, copper oxide displays enhanced electrical conductivity, catalytic activity, and antimicrobial efficacy when compared to their bulk counterparts. This distinctive behavior opens avenues for a spectrum of innovative applications that benefit from their nanoscale characteristics.
Moreover, the composition of copper oxide nanoparticles can be modified through various synthesis techniques, influencing their overall properties. Thus, knowing their definition and composition is essential for leveraging their potential, especially in research and industry.
Historical Development
The historical journey of copper oxide nanoparticles dates back several decades. Initial research concentrated on the properties of copper and copper oxides in bulk form. However, as nanotechnology began to emerge in the late 20th century, the focus shifted towards the nanoscale.
In the early 1990s, researchers discovered that reducing the size of materials to the nanoscale caused significant changes in their physical and chemical properties. This realization spurred investigations into the synthesis and characterization of nanoparticles, particularly copper oxides. Experimental studies began to emerge, demonstrating the unique characteristics of these materials, especially their enhanced catalytic and optical properties.
By the early 2000s, various synthesis methods were developed, enabling researchers to produce copper oxide nanoparticles more consistently and with higher quality. The advent of new techniques, such as sol-gel and chemical vapor deposition, facilitated further exploration of their potential applications. Today, copper oxide nanoparticles are studied extensively, not only in laboratories but also in various industries, including healthcare, electronics, and environmental science. This historical context underscores the evolution of copper oxide nanoparticles from concept to application, emphasizing their relevance in modern research and technology.
Synthesis Methods
The synthesis of copper oxide nanoparticles is critical for understanding their versatility and range of applications. Different methods offer various advantages that can affect particle size, shape, and overall properties. It is important to select the appropriate synthesis technique to achieve desired characteristics, tailored for specific applications in fields like medicine, electronics, and environmental science. The appropriate synthesis method can provide control over the physical and chemical properties of the nanoparticles, leading to improved performance in various applications.
Chemical Methods
Chemical methods are widely used for the synthesis of copper oxide nanoparticles due to their ability to produce high purity and uniform particles. These methods often enable precise control of parameters such as particle size and morphology, which are essential for optimizing the function of the nanoparticles in applications.
Sol-gel Technique
The sol-gel technique is a popular method for synthesizing copper oxide nanoparticles. This process involves the transition of a solution into a solid gel phase. A key characteristic of the sol-gel technique is its ability to produce nanoparticles at relatively low temperatures while maintaining compositional uniformity. This is beneficial because lower temperatures can prevent the incorporation of unwanted impurities.
One unique feature of the sol-gel method is the versatility in precursor selection, which may include various metal salts and alcohols. The method allows for easy tuning of the organic matrix and the synthesis conditions. The advantages include a wide range of compositional possibilities and the capability to produce large quantities of nanoparticles. However, one disadvantage may be the time-consuming steps involved in drying and calcination, which can extend the overall synthesis time.
Chemical Vapor Deposition
Chemical Vapor Deposition (CVD) is another significant method for producing copper oxide nanoparticles. This technique involves the chemical reaction of gaseous precursors to form a solid product on substrates. A key characteristic of CVD is its ability to create thin films and nanoparticles with high purity and excellent density control.
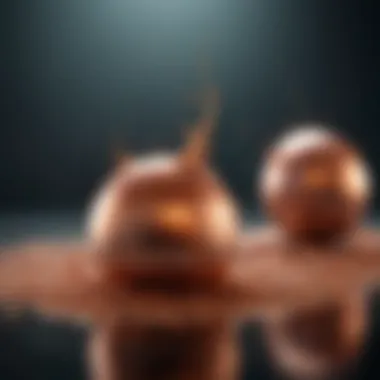
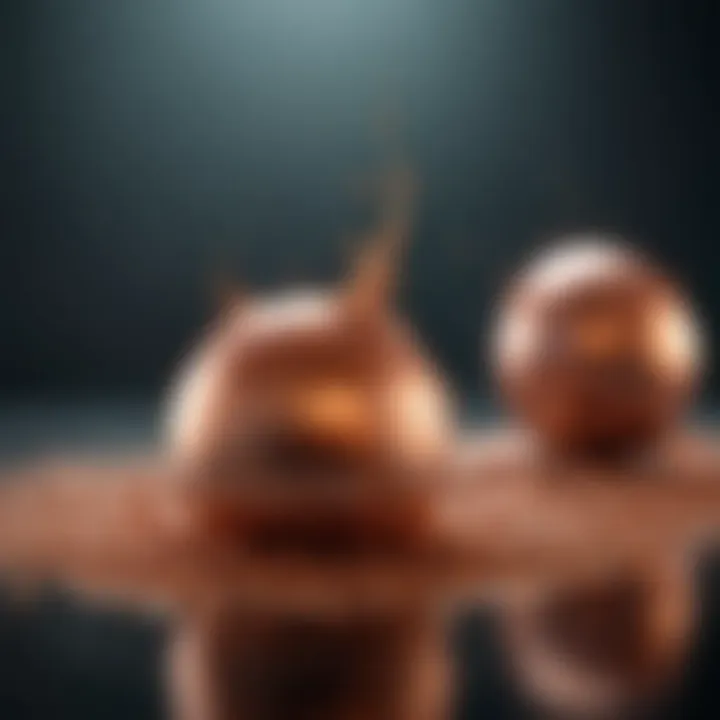
CVD is a beneficial choice for instances where uniform coating and homogenous distribution are essential, such as in electronic applications. Its ability to produce high-quality films is well recognized. The unique feature of CVD is its scalability, which can be adjusted based on operational needs. However, the major disadvantage of CVD is the requirement for complex and often costly equipment, which can limit accessibility to research institutions without adequate funding.
Physical Methods
Physical methods provide alternative approaches for synthesizing copper oxide nanoparticles. These techniques often involve mechanical or thermal processes and can yield nanoparticles with specific physical properties, often desired in electronic or structural applications.
Milling Techniques
Milling techniques are physical processes that generate nanoparticles by mechanically breaking down bulk materials. This method allows for a straightforward approach to producing copper oxide nanoparticles and is considered a cost-effective process. A key characteristic of milling is its capability to obtain a wide range of particle sizes.
The primary advantage of milling is the simplicity and ease of operation. It can swiftly produce nanoparticles without extensive chemical handling. Nonetheless, one limitation is the possible contamination from milling media, which can introduce unwanted elements, affecting the purity of the nanoparticles produced.
Laser Ablation
Laser ablation is a sophisticated method that involves using focused laser beams to remove material from a target, which subsequently forms nanoparticles. The key characteristic of laser ablation is its ability to produce nanoparticles in a controlled manner, yielding materials of high purity and size uniformity.
Laser ablation is a popular choice due to its high precision and the ability to generate nanoparticles without chemicals that may alter their properties. This method can also produce unique morphologies depending on the laser parameters used. However, the disadvantages include high operational costs and the necessity for advanced equipment, which may limit practical application in some settings.
Biological Methods
Biological methods for synthesizing copper oxide nanoparticles have gained popularity due to their environmentally friendly approach and potential for scalability. These methods often utilize natural processes to derive nanoparticles, contributing to sustainable practices in nanotechnology.
Plant Extracts
The use of plant extracts for the synthesis of copper oxide nanoparticles involves leveraging phytochemicals in plants to facilitate reduction. One key aspect of utilizing plant extracts is their rich content of antioxidants, which can assist in the nanoparticle formation process.
Plant extracts are beneficial due to their sustainability and low environmental impact. The unique feature of this method is its capability to produce nanoparticles without the use of toxic chemicals or high energy consumption. However, the indefinite nature of the extraction process can lead to variability in nanoparticle characteristics, which may present challenges in standardization.
Microbial Synthesis
Microbial synthesis involves using microorganisms to produce copper oxide nanoparticles. This method highlights an innovative approach, utilizing bacteria or fungi to synthesize materials naturally. A key characteristic of microbial synthesis is its ability to produce biocompatible nanoparticles suitable for medical applications.
Microbial synthesis is frequently regarded as beneficial because it avoids toxic chemicals and leverages natural biological processes. The unique aspect of this method lies in the potential for tailoring nanoparticle properties through genetic modification of the microbes. However, one disadvantage is the relatively slower production rate compared to chemical or physical methods, which may not be suitable for large-scale applications.
Characterization Techniques
Characterization techniques are essential for understanding the properties and behavior of copper oxide nanoparticles. The accurate characterization of these materials is crucial to maximize their potential applications in various fields. Proper characterization can reveal critical details about size, morphology, structure, and optical properties. This analysis ultimately aids in tailoring nanoparticles for specific uses, ensuring efficacy and safety.
Microscopic Analysis
Microscopic analysis underpins the assessment of physical attributes in nanoparticles. Two main techniques play pivotal roles: Transmission Electron Microscopy and Scanning Electron Microscopy.
Transmission Electron Microscopy
Transmission Electron Microscopy, often called TEM, allows for the investigation of the internal structure of nanoparticles. TEM can provide images with atomic resolution, revealing insights into shape, size, and crystal structure. This high-resolution capability is a key characteristic of TEM, making it a preferred tool in nanoparticle studies.
The unique feature of TEM is its ability to analyze specimens at such high magnification. It can differentiate between various phases within a nanoparticle, which is vital for understanding how different structures impact their functionality.
However, there are advantages and disadvantages to using TEM. On the plus side, the resolution is extremely high. On the downside, sample preparation can be complex and time-consuming, which might introduce artifacts. Thus, while TEM is a powerful method, careful consideration is necessary during the sample preparation phase.
Scanning Electron Microscopy
Scanning Electron Microscopy, known as SEM, is another technique utilized for examining the surfaces of copper oxide nanoparticles. SEM provides detailed three-dimensional images and can analyze surface morphology effectively. Its key characteristic is the ability to generate high-depth-of-field images, showcasing surface details in a way that TEM cannot.
One of the unique features of SEM is its versatility. It can be used for various types of samples, which makes it a beneficial choice in nanoparticle research. Additionally, SEM is faster than TEM regarding sample observation.
However, SEM also has drawbacks. The resolution is lower compared to TEM, meaning it may not capture fine internal structures. Furthermore, the samples often require a conductive coating, which may alter the nanoparticles’ behavior. Thus, while SEM is beneficial, it is essential to consider its limitations.
Spectroscopic Methods
Spectroscopic methods complement microscopic analyses, providing insight into the chemical and physical properties of nanoparticles. Two prevalent techniques are X-ray Diffraction and UV-Vis Spectroscopy.
X-ray Diffraction
X-ray Diffraction, abbreviated as XRD, serves as a fundamental technique for determining the crystalline structure of copper oxide nanoparticles. This method can yield information about the phase composition of the material. The key characteristic of XRD is its ability to identify and differentiate between various crystalline phases, which is crucial for assessing purity and phase stability.
The unique feature of XRD is its non-destructive nature, allowing for the study of samples without altering them. The advantages of this technique include its wide acceptance in research and straightforward data interpretation. However, it requires well-crystallized samples for accurate results, which could be a limiting factor in some cases.
UV-Vis Spectroscopy
UV-Vis Spectroscopy focuses on the optical properties of nanoparticles. This method measures the absorbance of UV and visible light, providing information on electronic transitions within the material. A notable characteristic of UV-Vis Spectroscopy is its ability to monitor the optical band gap of the nanoparticles efficiently.
The unique aspect of UV-Vis Spectroscopy is its relative simplicity and speed. It does not usually require extensive sample preparation. Nevertheless, it may not reveal detailed structural information, which limits its utility when comprehensive characterization is needed. The complementarity of UV-Vis Spectroscopy with other characterization methods enhances its value in the study of nanoparticles.
Physical Properties
Understanding the physical properties of copper oxide nanoparticles is crucial for their effective utilization across various fields. These nanoparticles exhibit unique characteristics that influence their performance in applications ranging from electronics to medicine. The specific physical properties—optical, electrical, and thermal—determine how these nanoparticles interact with their environment, ultimately affecting their efficacy and potential in real-world scenarios.
Optical Properties
The optical properties of copper oxide nanoparticles have significant implications, particularly in fields like photovoltaics and sensors. These nanoparticles can absorb and scatter light effectively due to their distinct size and shape.
Copper oxide nanoparticles exhibit a strong absorption spectrum in the visible region, which can be attributed to their electronic structure. This absorption can be manipulated by altering the synthesis conditions, allowing for tailored optical responses. For example, smaller nanoparticles typically show greater absorption compared to larger ones, which leads to increased light harvesting efficiency in solar cells. Furthermore, these properties play a vital role in their application as photothermal agents in medical therapies, where they convert absorbed light into heat to target and destroy cancerous cells.
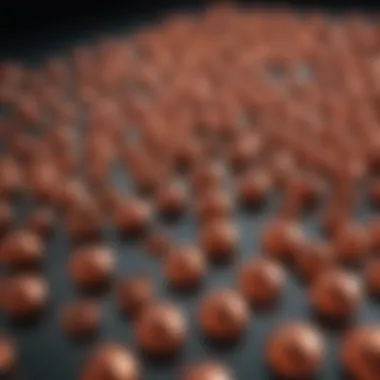
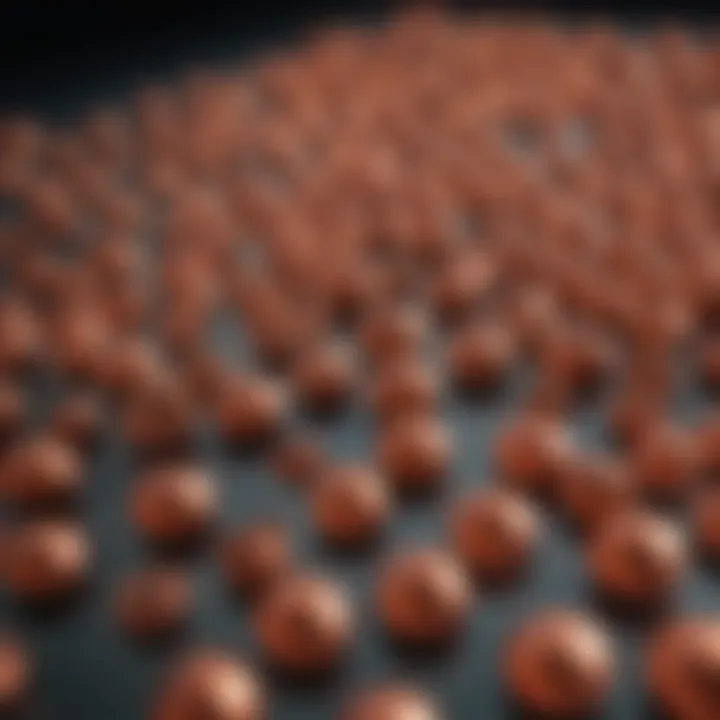
Electrical Properties
The electrical properties of copper oxide nanoparticles are essential for their application in electronic devices. These nanoparticles display semiconductor behavior, which makes them suitable candidates for various applications such as sensors and conductive inks. The conductivity of copper oxide nanoparticles can be influenced by factors such as temperature, environmental conditions, and the presence of impurities.
One important feature is their potential in gas sensing applications. The electrical resistance of these nanoparticles changes in response to gas concentration, making them effective in detecting pollutants and toxic gases. Additionally, the charge carrier mobility in copper oxide nanoparticles can be engineered through doping processes to improve their electrical performance, which is crucial for the development of more efficient electronic devices. Ultimately, optimizing these properties can lead to advancements in the functionality and efficiency of electronic circuits and sensors.
Thermal Properties
The thermal properties of copper oxide nanoparticles are a critical area of study, especially in applications that involve thermal management. These nanoparticles possess high thermal stability, which allows them to maintain their performance in harsh environments. Understanding how they conduct heat is essential for their use in thermal insulation and in devices that require efficient heat dissipation.
Copper oxide nanoparticles also exhibit interesting thermal conductivity characteristics. They can act as effective heat transfer materials in composites, which is advantageous for applications such as electronics cooling systems. Moreover, their ability to withstand elevated temperatures makes them suitable for various industrial applications.
In summary, the physical properties of copper oxide nanoparticles play a pivotal role in determining their functionality in a range of applications. The specific characteristics, such as optical response, electrical conductivity, and thermal stability, underscore the versatility and potential of these materials in advancing technology across multiple domains.
Chemical Properties
The study of chemical properties is essential for understanding copper oxide nanoparticles. These properties fundamentally dictate how these nanoparticles interact in different environments. Knowledge of these chemical characteristics also supports effective application in various fields.
Reactivity
Reactivity refers to the tendency of copper oxide nanoparticles to undergo chemical reactions with other substances. Their surface chemistry plays a vital role in determining their reactivity. The high surface area-to-volume ratio of nanoparticles makes them more reactive compared to their bulk counterparts.
Some typical reactions include oxidation and reduction processes. For instance, copper(II) oxide can lose oxygen under high temperatures, thus transforming into copper metal. This property can be advantageous in catalysis, where the nanoparticles can be used to facilitate reactions effectively.
A key aspect to consider is the fact that reactivity of copper oxide nanoparticles can be influenced by several factors, such as the size of the particles, their shape, and the surrounding medium. Smaller particles often demonstrate increased reactivity due to their greater surface area. Thus, controlling these parameters during synthesis is crucial for tailoring the performance of copper oxide nanoparticles in practical applications.
Catalytic Activity
Catalytic activity of copper oxide nanoparticles has attracted significant research interest. They have shown great potential as catalysts in chemical reactions, particularly in oxidation reactions. For example, copper oxide is used in processes like methanol oxidation and CO oxidation.
This catalytic capability arises primarily from their ability to facilitate electron transfer. The facets of the copper oxide can promote the adsorption of reactant molecules, enhancing their conversion rates. Additionally, copper oxide nanoparticles can serve in reactions that involve both acid and base, showcasing their dual functionality.
Environmentally, the catalytic properties extend to the degradation of pollutants. Copper oxide nanoparticles have demonstrated effectiveness in breaking down organic contaminants in water. This aspect aligns with growing concerns over environmental protection and cleanup.
"Understanding the chemical properties of nanoparticles is critical for harnessing their full potential in real-world applications."
As scientists delve deeper into the realm of copper oxide nanoparticles, the continuous exploration of their chemical properties opens new avenues for innovation.
Applications in Medicine
The applications of copper oxide nanoparticles (CuO NPs) in medicine demonstrate significant potential for advancements in healthcare. Their unique properties, including antimicrobial effects and suitability for drug delivery systems, position them as valuable tools in medical research and clinical practices. This section explores the key applications, emphasizing their relevance in developing innovative therapeutic approaches.
Antimicrobial Properties
Copper oxide nanoparticles have garnered attention due to their potent antimicrobial activity. Research shows that these nanoparticles can effectively inhibit the growth of various pathogens, including bacteria and fungi. The inherent physiochemical properties, such as small size and high surface area, facilitate greater interaction with microbial cells. This can lead to enhanced penetration and disruption of the microbial cell membrane, ultimately resulting in cell death.
Factors affecting the antimicrobial efficacy include nanoparticle size, concentration, and exposure time. Studies indicate that smaller nanoparticles tend to exhibit stronger antimicrobial activity. Additionally, it is crucial to consider the potential for development of resistance among pathogens. Understanding this mechanism is vital to enhance the long-term effectiveness of copper oxide nanoparticles in medicinal applications.
"The unique properties of copper oxide nanoparticles make them an effective candidate for combating antibiotic-resistant strains."
The incorporation of CuO NPs in coatings and textiles might extend their antimicrobial properties to everyday items, thereby reducing infection rates in clinical settings. Furthermore, research continues to explore their synergy with traditional antibiotics to improve treatment outcomes, making copper oxide nanoparticles a promising avenue in addressing public health concerns.
Drug Delivery Systems
Another important application of copper oxide nanoparticles is their role in drug delivery systems. Traditional drug delivery methods often face challenges, including low bioavailability and systemic toxicity. CuO NPs can overcome some of these limitations due to their ability to encapsulate therapeutic agents, ensuring targeted delivery to disease sites.
The biological compatibility of copper oxide nanoparticles, along with their easy functionalization, allows for the development of multifunctional systems. For instance, CuO NPs can be modified to attach targeting ligands that direct them to specific cells or tissues. This ensures that drugs are released in a controlled manner, maximizing therapeutic effects while minimizing side effects.
Some benefits of employing copper oxide nanoparticles for drug delivery include:
- Enhanced solubility of poorly soluble drugs.
- Controlled release profiles, which leads to sustained therapeutic action.
- Potential for combination therapy, where multiple drugs can be delivered simultaneously.
Research also indicates that copper oxide nanoparticles can assist in overcoming biological barriers, such as the blood-brain barrier, allowing for targeted treatment of neurological diseases. Their versatility in drug delivery applications indicates broad future potential, as ongoing studies aim to refine and test these systems in various therapeutic contexts, representing an important field within nanomedicine.
Applications in Electronics
Copper oxide nanoparticles are increasingly being recognized for their significant role in electronics. Their unique properties facilitate advancements in various electronic components, providing both efficiency and cost-effectiveness. Understanding these applications helps researchers, educators, and industry professionals grasp the potential of these nanoparticles in shaping the future of electronic technologies.
Conductive Inks
Conductive inks containing copper oxide nanoparticles are a key innovation in the field of printed electronics. These inks are essential for developing flexible electronic circuits, which can be applied to a wide range of substrates. This flexibility allows for the production of electronic components that are not only lightweight but also tailored to different shapes and surfaces.
The advantages of using copper oxide nanoparticles in conductive inks include:
- Enhanced Conductivity: Copper oxide nanoparticles exhibit excellent electrical conductivity, making them ideal for circuit applications.
- Cost Effectiveness: Copper is relatively cheap compared to other conductive materials like silver, making these inks financially attractive for mass production.
- Environmental Impact: Utilizing copper oxide, which is less harmful than other materials, aligns with sustainable practices in electronics.
These properties enable the design of eco-friendly products that do not compromise performance. Furthermore, conductive inks can facilitate advances in wearable technology and Internet-of-Things (IoT) devices by enabling seamless integration of electronic components.
Sensors
Sensors represent another vital application of copper oxide nanoparticles in electronics. Their use in gas sensors is particularly promising, as these nanoparticles can effectively detect various gases at low concentrations. The high surface area of copper oxide nanoparticles enhances their sensitivity, leading to improved signal detection accuracy.
The notable features of copper oxide-based sensors include:
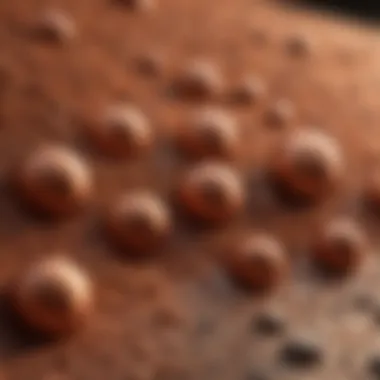
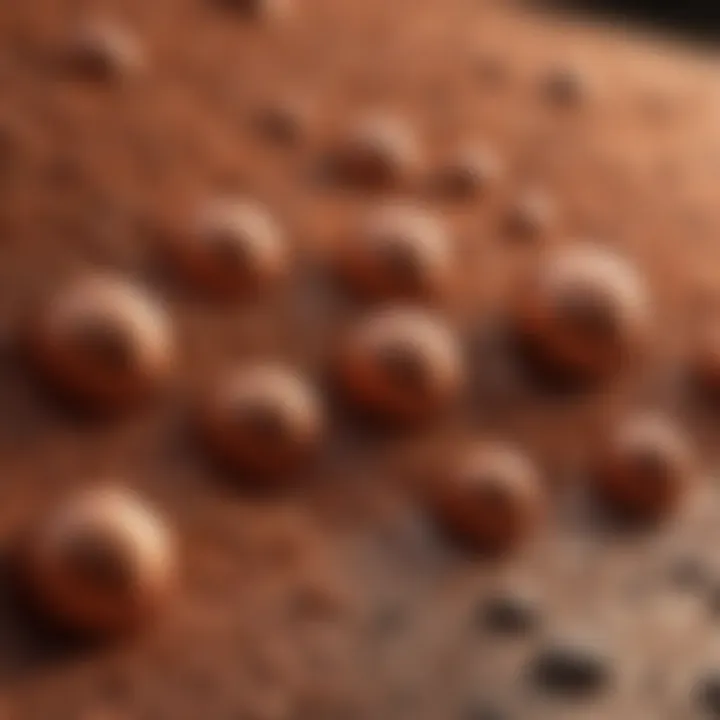
- High Sensitivity: These sensors can detect minute changes in gas concentrations, making them ideal for environmental monitoring.
- Selective Detection: Certain formulations of copper oxide may selectively respond to specific gases, increasing their utility in diverse applications such as industrial safety and pollution control.
- Rapid Response Time: The response time of copper oxide sensors is notably quick, which is crucial for timely data in critical environments.
In summary, the applications in electronics for copper oxide nanoparticles are vast and growing. Conductive inks and sensors exemplify the potential of these materials to innovate and enhance current technologies. As research advances, new applications may emerge, further solidifying the importance of copper oxide nanoparticles in the electronics field.
"Copper oxide nanoparticles offer a unique combination of properties that can lead to significant improvements in electronic devices. Their applications in conductive inks and sensors are just the beginning of their potential."
These innovations not only promise to revolutionize existing technologies but also open doors for new applications, solidifying copper oxide nanoparticles as a fundamental component of future electronics.
Environmental Applications
The relevance of environmental applications of copper oxide nanoparticles extends beyond mere scientific inquiry. These nanoparticles exhibit unique properties that play a crucial role in addressing pressing environmental issues. Their importance is underscored by their applications in water purification and waste treatment, two critical areas in environmental management. Both sectors face challenges that demand innovative solutions, and copper oxide nanoparticles have potential as effective agents in these applications.
Water Purification
Water purification is a vital process that ensures access to clean drinking water, which is essential for public health. Copper oxide nanoparticles offer a promising approach for enhancing the efficiency of various purification methods. The nanoparticles possess antifungal and antibacterial properties, which can degrade harmful microorganisms commonly present in contaminated water.
In practical terms, these nanoparticles can be used in filtration systems, coatings, and membranes. When integrated into existing water treatment technologies, they can significantly improve pathogen removal rates. Some studies have demonstrated that copper oxide nanoparticles are effective against bacteria, such as E. coli, providing a dual functionality of disinfection and supporting traditional filtration.
Another aspect is the ability of copper oxide nanoparticles to adsorb heavy metals. This characteristic can aid in the removal of pollutants, such as lead and cadmium, thereby contributing to more comprehensive water treatment solutions. The effectiveness of copper oxide nanoparticles in such applications underscores their potential to revolutionize water purification processes, especially in regions where access to clean water is limited.
Waste Treatment
The challenge of waste management is growing globally, necessitating the development of efficient treatment technologies. Copper oxide nanoparticles contribute to waste treatment primarily through their catalytic properties. They can enhance the degradation of organic pollutants found in wastewater, such as dyes, pharmaceuticals, and pesticides.
The incorporation of copper oxide nanoparticles in waste treatment systems can lead to the development of more sustainable and effective systems. For instance, when these nanoparticles are used in conjunction with UV light or other energy sources, they can generate reactive oxygen species that break down complex organic molecules into simpler, less harmful compounds.
Moreover, their application in advanced oxidation processes (AOPs) shows great promise. AOPs can achieve significant degradation of pollutants, which is essential for meeting stringent environmental regulations. The use of copper oxide nanoparticles optimizes these processes, making them more cost-effective and efficient.
Copper oxide nanoparticles represent a dual advantage: they not only aid in minimizing environmental pollution but also enhance the overall efficiency of waste treatment systems.
Safety and Toxicology
Understanding safety and toxicology is crucial as we explore copper oxide nanoparticles. These materials, while promising for various applications, also raise significant questions regarding their potential health impacts and environmental consequences. Evaluating safety is not just about addressing risks. It's also about ensuring responsible development. This section highlights potential health risks and regulatory considerations in the context of copper oxide nanoparticles.
Potential Health Risks
Copper oxide nanoparticles may pose certain health risks due to their small size and high reactivity. Their ability to enter biological systems raises concerns in several areas:
- Inhalation Risks: Breathing in aerosols containing these nanoparticles can lead to pulmonary issues. Studies have shown that they can cause inflammation in lung tissue.
- Skin Exposure: Contact with skin may lead to irritation or allergic reactions in some individuals. Long-term exposure might increase the risk of dermatological conditions.
- Cytotoxic Effects: Research indicates that high concentrations of copper oxide nanoparticles can be toxic to various cell types, including neuronal cells. Such toxicity raises alarms regarding long-term exposure in medical and industrial applications.
"Addressing potential health risks is essential to harnessing the benefits of copper oxide nanoparticles."
Awareness of these risks is fundamental for researchers and professionals involved in the synthesis and application of copper oxide nanoparticles. Rigorous research is needed to fully understand and mitigate these risks over time.
Regulatory Considerations
The rising use of copper oxide nanoparticles has prompted regulatory bodies to formulate guidelines to ensure safety and compliance. Regulatory frameworks are necessary to assess potential risks and promote safe laboratory practices and industrial applications. Key points include:
- Safety Data Sheets (SDS): Manufacturers are required to provide SDS. This document outlines hazards, handling, and emergency procedures related to copper oxide nanoparticles.
- Material Safety: Various organizations, such as the Environmental Protection Agency (EPA), emphasize assessing the toxicity of nanomaterials. These assessments guide regulatory decisions on the allowable concentrations and exposure limits.
- International Guidelines: Adopting international best practices is vital. Organizations like OECD (Organization for Economic Co-operation and Development) focus on harmonizing testing for nanomaterials, which can inform national regulations.
Future Directions in Research
The study of copper oxide nanoparticles continues to evolve, presenting numerous future directions for research. This chapter emphasizes the importance of exploring both emerging synthesis methods and innovative applications. These aspects play a critical role in enhancing our understanding of copper oxide nanoparticles and their potential impact on technology and society. As researchers delve deeper into this field, they must consider how advancements in synthesis techniques can lead to improved properties and greater applicability across various sectors.
Emerging Synthesis Methods
Recent advancements in the synthesis of copper oxide nanoparticles have led to improved methods that are more efficient and environmentally friendly. One promising approach is the use of green synthesis techniques, which often employ natural compounds to facilitate the formation of nanoparticles. This method not only reduces the need for toxic reagents but also minimizes the environmental impact of synthesis processes. Such approaches promote sustainability while generating nanoparticles with favorable properties.
Additionally, the exploration of two-dimensional materials for the synthesis of copper oxide nanoparticles is gaining traction. These methods employ techniques such as chemical exfoliation and layer-by-layer assembly. This could offer tailored properties, enhancing the versatility of nanoparticles for specific applications. Furthermore, the incorporation of microwave synthesis has shown potential for significantly reducing reaction times while maintaining size and shape uniformity.
Innovative Applications
As research progresses, the applications of copper oxide nanoparticles are expanding beyond traditional fields such as medicine and electronics. One notable area is in energy storage and conversion technologies. Developers are investigating the use of copper oxide nanoparticles in batteries and supercapacitors, focusing on their ability to enhance energy density and charge-discharge rates. This can lead to more efficient energy systems, contributing to the development of sustainable technologies.
Moreover, the potential of these nanoparticles in catalysis is being heavily explored. Their unique surface properties can facilitate chemical reactions more effectively than bulk materials. This drives innovation in chemical production processes, leading to greener and more cost-effective outcomes.
Research in copper oxide nanoparticles is not only significant for enhancing existing technologies but also for paving the way toward new solutions to global challenges.
Other future directions involve studying their roles in environmental applications, particularly in the remediation of pollutants. Their surface reactivity can be utilized to absorb heavy metals and organic compounds from contaminated water. This aspect opens possibilities for sustainable water treatment solutions.
Culmination
The conclusion serves as a crucial component of this article, bringing together the various threads discussed throughout the sections. It encapsulates the significance of copper oxide nanoparticles, highlighting their synthesis techniques, unique properties, and their far-reaching applications. The synthesis methods outlined provide foundational knowledge for researchers and professionals seeking to explore this area further. The emphasis on the diverse properties showcases why these nanoparticles are increasingly relevant in science and industry today.
Summary of Findings
In reviewing the nature and uses of copper oxide nanoparticles, several key findings emerge:
- Diverse Synthesis Methods: Various techniques including chemical, physical, and biological methods offer flexibility in producing copper oxide nanoparticles tailored for specific applications.
- Unique Properties: The optical, electrical, and thermal characteristics make these nanoparticles suitable for advanced applications, particularly in electronics and medicine.
- Broad Applications: Their functions extend across medical applications, such as antimicrobial agents and drug delivery, to electronic components and environmental solutions, including water treatment and sensors.
These findings illustrate a clear trajectory of growth and potential in the field of nanotechnology, focusing on copper oxide nanoparticles.
Implications for Various Fields
The implications of copper oxide nanoparticles span across multiple disciplines:
- Medicine: Their antimicrobial and therapeutic properties indicate a promising future in treating infections and enhancing drug delivery systems.
- Electronics: As components for conductive inks and sensors, they could lead to more efficient electronic devices and smarter technologies.
- Environmental Science: Their ability to purify water and treat waste demonstrates a significant impact on environmental sustainability.
Overall, copper oxide nanoparticles present opportunities for innovation and interdisciplinary collaboration, prompting continued investigation into their synthesis and optimal application. As research expands, these nanoparticles may play a pivotal role in tackling contemporary challenges, making their study essential for future advancements in nanotechnology.