Cancer Cell Metabolism: Decoding Tumor Growth Dynamics
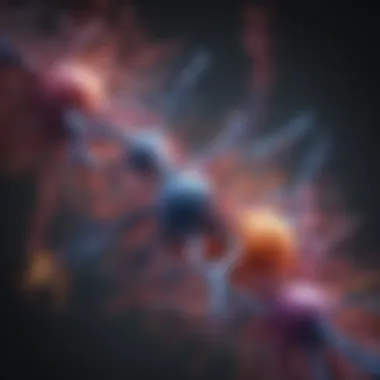
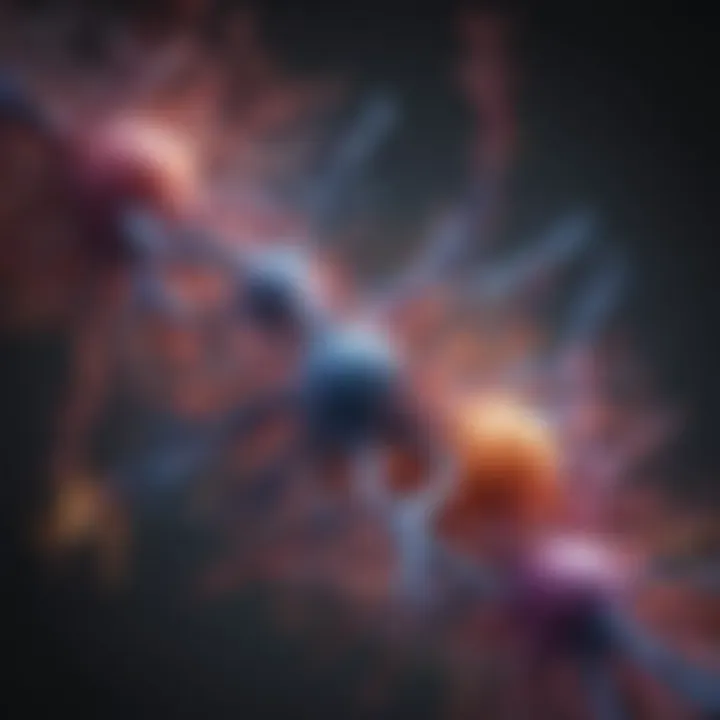
Intro
Cancer cell metabolism plays a pivotal role in the progression of tumorigenesis. This field of study acknowledges that cancer cells adopt distinct metabolic pathways, diverging significantly from those utilized by normal cells. Such adaptations in metabolic processes are not merely by-products of tumorigenesis; they are active contributors to the malignancy's growth, survival, and ability to withstand treatment.
Understanding these unique metabolic frameworks is essential for identifying potential therapeutic targets. By evaluating the intersecting pathways of metabolism and tumor biology, researchers can gain insights into how these metabolic alterations facilitate a thriving tumor environment. The implications of these adaptations are broad, impacting strategies for intervention, treatment efficacy, and ultimately, patient outcomes.
In this exploration of cancer cell metabolism, we will investigate the mechanisms behind these metabolic changes, their implications for tumor behavior, and the potential future directions in research focused on therapeutic enhancements.
Methodology
Study Design
The exploration of cancer cell metabolism relies on a multidisciplinary approach. Researchers often employ a combination of biochemical assays, genomics, and imaging techniques. This integration assists in mapping the metabolic pathways involved in cancer progression. The complexity of cancer metabolism can be appreciated through studies that design experiments to analyze both in vitro and in vivo models of tumor growth. Significant focus is placed on how these metabolic pathways are regulated by specific signaling molecules and endogenous conditions.
Data Collection Techniques
Data collection is critical in understanding how cancer cells exploit metabolic pathways. Methods include:
- Genomic Sequencing: This technique reveals mutations in metabolic genes that alter cell function.
- Metabolomics: Analyzing the metabolic profile of cancer cells provides insights into altered metabolite levels.
- Proteomics: Understanding protein expression related to metabolic enzymes aids in elucidating the metabolic shifts.
The integration of these methodologies allows for a comprehensive assessment of how cancer cells navigate and utilize their energetic resources to promote tumorigenesis.
Discussion
Interpretation of Results
The results from studies on cancer cell metabolism indicate a preference for glycolysis over oxidative phosphorylation, famously known as the Warburg effect. This observation suggests that tumor cells prioritize rapid energy production and create a favorable environment for growth. Furthermore, metabolic reprogramming often results in the accumulation of onco-metabolites, which can influence gene expression and cell signaling pathways.
Limitations of the Study
Despite advancements, research in cancer cell metabolism faces challenges. The complexity of metabolic networks often hinders the ability to draw definitive conclusions. Moreover, heterogeneity among tumors leads to variability in metabolic signatures, complicating the identification of universal targets for therapy.
Future Research Directions
The elucidation of these metabolic pathways paves the way for innovative therapeutic strategies. Future research may focus on:
- Development of inhibitors targeting specific metabolic enzymes in cancer cells.
- Exploration of metabolic flexibility and its role in treatment resistance.
- Investigating the effects of the tumor microenvironment on cancer metabolism.
"A deeper understanding of cancer cell metabolism not only shapes therapeutic strategies but also redefines our approaches towards cancer treatment and prevention."
Prelims to Cancer Cell Metabolism
Understanding cancer cell metabolism is crucial for both clinical and research settings. Cancer cells exhibit distinct metabolic behaviors compared to normal cells. This difference underlies their growth, survival, and resistance to treatments, making it a pivotal area of study. Delving into cancer cell metabolism offers insights that could lead to innovative therapeutic strategies and enhance current treatment regimens.
Defining Cancer Cell Metabolism
Cancer cell metabolism refers to the biochemical processes that enable cancer cells to acquire energy and synthesize the necessary components for growth and proliferation. Unlike normal cells, which primarily rely on oxidative phosphorylation for ATP production, cancerous cells often favor glycolysis, even in the presence of oxygen. This preference for anaerobic metabolism—termed the Warburg effect—supports rapid proliferation and survival in hostile environments. Understanding these metabolic pathways is essential for developing effective interventions that target the unique needs of cancer cells.
Historical Perspective on Cancer Metabolism Research
Research on cancer metabolism has a rich history that dates back over a century. In the 1920s, Otto Warburg noted that cancer cells metabolizes glucose through glycolysis at a much higher rate than normal cells, even with sufficient oxygen. This observation laid the groundwork for further investigation into the metabolic alterations present in tumors. Over the decades, advancements in biochemistry and molecular biology have unveiled the complexity of cancer metabolism. Today, ongoing research continues to unravel metabolic pathways and adaptations, illuminating how these processes influence tumorigenesis.
The understanding of cancer metabolism has evolved significantly, shifting focus from purely genetic factors to include metabolic reprogramming as a key player in tumorigenesis.
Understanding the historical context is critical as it frames the ongoing discussions in cancer research. It highlights the necessity of interdisciplinary approaches in studying cancer, blending insights from metabolism, genetics, and signaling pathways. As the field continues to change, new technologies and methodologies are expected to deepen our understanding of cancer cell metabolism.
The Metabolic Phenotype of Cancer Cells
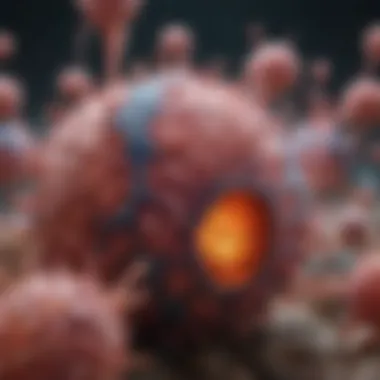
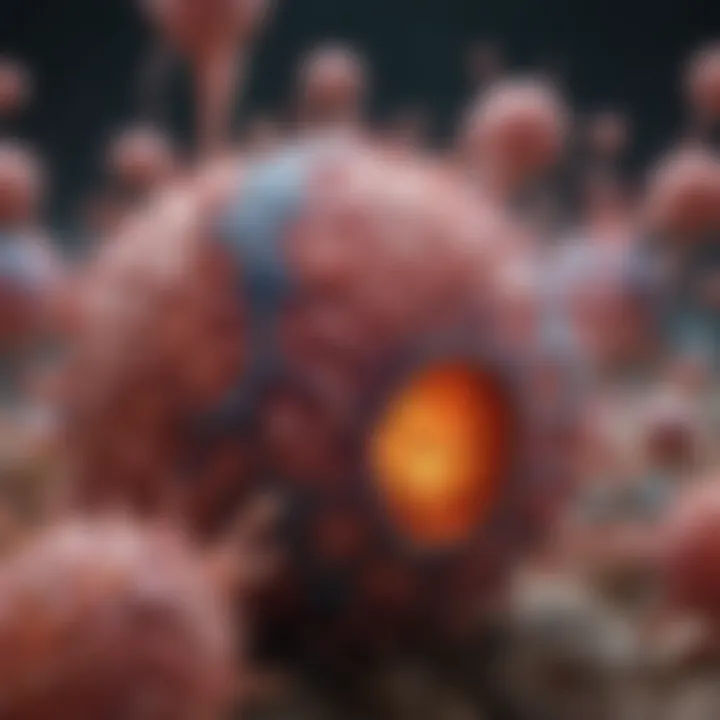
The understanding of the metabolic phenotype of cancer cells is crucial in the context of tumor biology. Cancer cells exhibit unique metabolic alterations that not only support their rapid growth but also enhance their survival under unfavorable conditions. Distinct from normal cells, the metabolic phenotype encompasses changes in energy production, nutrient utilization, and biosynthetic capabilities. These adaptations allow cancer cells to thrive even as the tumor microenvironment presents challenges such as nutrient deprivation, hypoxia, and oxidative stress.
The metabolic phenotype fundamentally enables cancer cells to utilize energy more effectively. Through altered metabolic pathways, cancerous cells can generate ATP in a manner that is not only efficient but suited for their high proliferation rate. Better understanding these pathways holds potential for identifying therapeutic targets that can disrupt the energy supply to tumors, ultimately improving treatment options.
Altered ATP Production
One of the hallmark features of cancer cell metabolism is altered ATP production. Tumor cells often display heightened glycolytic activity, even in the presence of oxygen. This phenomenon is termed the Warburg Effect, named after Otto Warburg, who first described this metabolic shift. Unlike normal cells that preferentially use mitochondrial respiration for energy, cancer cells tend to rely more on glycolysis to produce ATP. This shift in energy production can be advantageous for rapidly dividing cells. It allows for more metabolic intermediates to be diverted into pathways that support rapid cell growth and division.
"The capability to generate ATP through glycolysis provides cancer cells with both energy and building blocks essential for growth."
Moreover, this alteration in ATP production is often coupled with changes in the expression of proteins involved in glycolytic and mitochondrial metabolism, reflecting the so-called metabolic reprogramming typical of cancer cells. As a result, treatments that target the unique energy metabolism of these cells can potentially inhibit their growth significantly.
Aerobic Glycolysis and the Warburg Effect
Aerobic glycolysis remains a central feature of cancer metabolism. Under normoxic conditions, normal cells tend to favor oxidative phosphorylation for ATP production. Cancer cells, however, adopt aerobic glycolysis, converting glucose to lactate even when oxygen is plentiful. This not only facilitates increased ATP production but also the synthesis of cellular components necessary for proliferation. The inefficiency of glycolysis compared to oxidative phosphorylation allows for faster processing of glucose and generates precursors for biosynthetic pathways.
The Warburg Effect also contributes to the acidification of the tumor microenvironment due to the accumulation of lactate. This acidic environment can promote invasive properties of cancer cells and enhance their ability to evade immune detection. Understanding this metabolic trait is vital not only for delineating tumor biology but also for developing therapies that can exploit these metabolic vulnerabilities.
Deregulated Biosynthesis Pathways
The metabolic profile of cancer cells is also characterized by deregulated biosynthesis pathways. Cancer cells often exhibit increased de novo lipogenesis, enhancing their ability to synthesize fatty acids from glucose and other substrates. This is important for maintaining cellular membrane integrity and signaling pathways but can also provide energy reserves. Furthermore, cancer cells exhibit altered amino acid metabolism, which enables them to adapt their growth under varying nutrient conditions.
These metabolic alterations are tightly linked to oncogenic signaling pathways, which further promote the deregulation of biosynthetic processes. By targeting these pathways, research is focusing on ways to inhibit tumor growth by disrupting the metabolic needs of these cells.
Key Metabolic Pathways in Cancer
Understanding the key metabolic pathways in cancer is crucial for deciphering the unique energetic needs of tumor cells. Cancer cells exhibit altered metabolism compared to normal cells, adapting their metabolic processes to support rapid proliferation and survival in challenging environments. These pathways are essential for the synthesis of macromolecules, energy supply, and maintenance of redox homeostasis. Exploring these pathways not only provides insights into tumor biology but also identifies potential therapeutic targets.
Glycolysis
Glycolysis is the metabolic process whereby glucose is converted into pyruvate, yielding a small amount of ATP and reduced NADH in the process. While normal cells primarily perform glycolysis under low oxygen conditions, cancer cells favor glycolysis even in the presence of oxygen. This phenomenon, often dubbed the Warburg effect, allows cancer cells to generate energy rapidly while diverting metabolites towards anabolic pathways necessary for biomass accumulation. The upregulation of key enzymes, such as hexokinase and phosphofructokinase, facilitates this metabolic shift.
A critical consequence of this reliance on glycolysis is the production of lactate, which can acidify the tumor microenvironment, promoting a more invasive phenotype. Furthermore, inhibitors targeting glycolytic enzymes are under investigation as a potential strategy to limit tumor growth.
The TCA Cycle
The tricarboxylic acid (TCA) cycle, also known as the citric acid cycle or Krebs cycle, plays a pivotal role in cancer cell metabolism by supplying crucial intermediates for various biosynthetic processes. The TCA cycle operates in mitochondria, where it generates NADH and FAD for the electron transport chain. In cancer, there is often a dysregulation of this cycle due to mutations in metabolic enzymes or alterations in signaling pathways.
Oncogenic mutations can lead to the accumulation of certain metabolites, such as fumarate and succinate, which act as signaling molecules in pathways regulating cell growth and survival. Additionally, targeting the enzymes of the TCA cycle may present novel opportunities for therapeutic interventions. For instance, inhibitors of isocitrate dehydrogenase have shown promise in clinical trials for specific cancers.
Fatty Acid Metabolism
Fatty acid metabolism has emerged as a significant player in cancer cell metabolism. Cancer cells often upregulate fatty acid synthesis to meet their increased demand for membrane synthesis. Fatty acid synthase, the key enzyme in this process, is frequently overexpressed in various cancers, correlating with poor prognosis.
Moreover, fatty acids can be broken down through beta-oxidation to produce ATP, serving as an alternative energy source during periods of metabolic stress. Understanding the balance between fatty acid synthesis and degradation is essential for targeting lipid metabolism in cancer therapy. Inhibitors that block fatty acid synthesis may help in disrupting the energy supply of tumors.
Amino Acid Metabolism
Amino acids are not just building blocks for proteins; they also play critical roles in cancer cell metabolism. Tumors often exhibit altered amino acid metabolism, with increased demand for certain amino acids such as glutamine, leucine, and serine. Glutamine, for instance, is a key nitrogen source and supports both the TCA cycle and lipid synthesis in cancer cells.
Additionally, enzyme dysregulation in amino acid pathways, like the upregulation of glutaminase, further highlights the metabolic plasticity of cancer cells. Targeting amino acid transport and metabolism offers potential therapeutic opportunities.
In summary, metabolic pathways like glycolysis, the TCA cycle, fatty acid metabolism, and amino acid metabolism each contribute distinctly to cancer cell survival and proliferation. Unraveling these pathways not only enriches our understanding of tumor biology but also points toward potential targets for intervention in cancer therapy.
Influence of the Tumor Microenvironment
The tumor microenvironment plays a critical role in cancer metabolism and tumor progression. It is not merely the sum of various cell types present in it, but a dynamic entity that influences cancer cell behavior, metabolism, and therapeutic responses. The interactions between tumor cells and their surrounding environment, including stromal cells, immune cells, extracellular matrix components, and signaling molecules, significantly affect how tumors grow and adapt. Understanding this influence can lead to better therapeutic strategies that target not just the tumor cells but also their microenvironment.
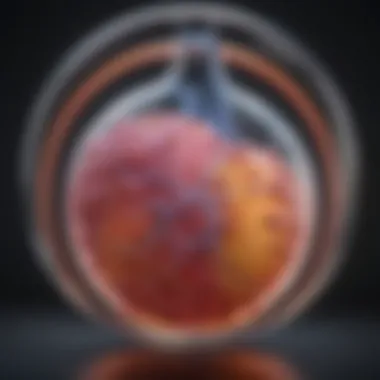
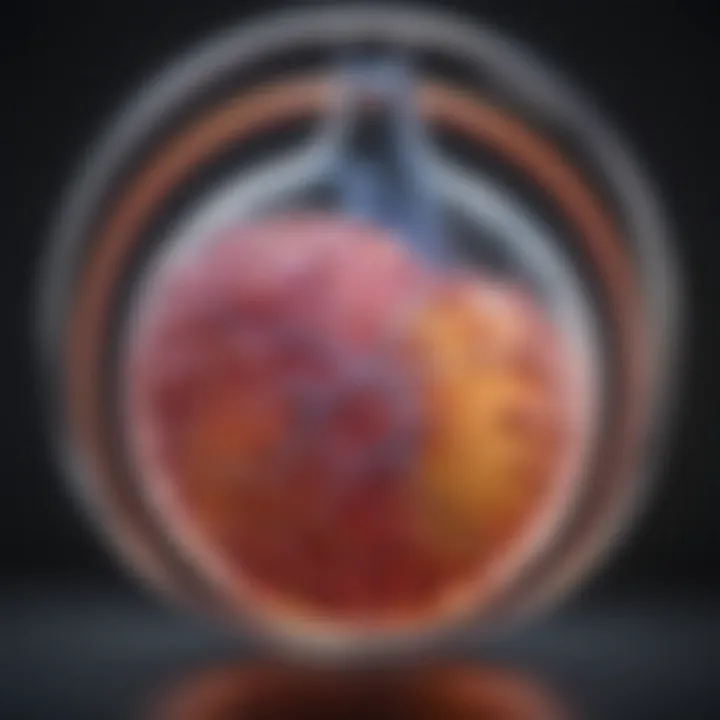
Hypoxia and Its Effects on Metabolism
Hypoxia, or low oxygen levels, is a common characteristic of solid tumors due to the rapid growth of cancer cells that outpaces blood supply. This condition forces tumor cells to adapt their metabolism. Cancer cells often switch from oxidative phosphorylation to glycolysis to meet their energy demands, even in the presence of oxygen. This phenomenon is known as the Warburg effect.
The adaptations to hypoxia lead to an accumulation of lactate in the tumor microenvironment, creating an acidic environment. This acidity can promote tumor invasion and metastasis while also influencing the immune response. For instance, the acidic microenvironment can hinder the ability of immune cells to function effectively against cancer cells.
Nutrient Availability and Competition
Nutrient availability in the tumor microenvironment is a crucial factor that dictates cancer cell metabolism. Tumors often experience competition for essential nutrients such as glucose, amino acids, and lipids. This competition can influence cancer cell proliferation and survival.
As tumors grow, they may develop mechanisms to increase nutrient uptake or alter metabolic pathways to utilize alternative sources. For example, many tumors upregulate glucose transporters to enhance glucose uptake or alter their amino acid metabolism to support rapid growth. Furthermore, the presence of stromal and immune cells can further complicate nutrient dynamics, as these cells may consume nutrients, limiting their availability to tumor cells.
Extracellular Matrix Contributions to Metabolism
The extracellular matrix (ECM) is more than just structural support for cells; it plays a significant role in regulating cancer metabolism. Components of the ECM, such as collagen and fibronectin, can influence the behavior and metabolic state of cancer cells.
Cancer cells interact with the ECM through various receptors, leading to signaling cascades that can affect metabolic pathways. For instance, signaling through integrins can lead to the activation of pathways that enhance glycolysis and lipid metabolism, supporting increased cellular proliferation. Additionally, changes in the ECM composition can alter mechanical properties and influence how cancer cells migrate and invade surrounding tissues. This could lead to enhanced tumor progression and metastasis.
"The tumor microenvironment is a pivotal player in regulating cancer cell metabolism and adapting to therapeutic challenges."
In summary, the tumor microenvironment is multifaceted and its influence on cancer cell metabolism is complex. Factors like hypoxia, nutrient availability, and extracellular matrix contributions profoundly shape the metabolic landscape of tumors. Understanding these interactions can foster the development of targeted therapies that address not only cancer cells but also the supporting microenvironment.
Signaling Pathways and Cancer Metabolism
In the context of cancer research, understanding signaling pathways is essential. These pathways govern how cancer cells metabolize nutrients and adapt to challenging environments. Cancer cells often exploit these pathways to maintain their growth and survival. For instance, they can modify their metabolic processes to counteract treatments aimed at diminishing their energy supply. Therefore, exploring these signaling pathways provides insights into the aggressive nature of cancer and points toward innovative therapeutic strategies.
The Role of mTOR Pathway
The mechanistic target of rapamycin (mTOR) pathway plays a significant role in regulating cell growth and metabolism. It integrates signals from growth factors, nutrients, and energy status. In cancer cells, mTOR signaling is often dysregulated, leading to increased protein synthesis and cellular proliferation. This overactivity contributes to the tumorigenic process.
Research has shown that inhibiting mTOR can reduce the proliferation of cancer cells. Drugs like rapamycin and its analogs are being explored in clinical settings. Thus, targeting the mTOR pathway may provide an effective approach in cancer therapy. It represents a vital intersection between cellular metabolism and cancer treatment.
AMPK Activation and Tumor Suppression
AMP-activated protein kinase (AMPK) functions as an energy sensor in cells. When cellular energy levels decline, AMPK is activated, promoting processes that generate ATP while inhibiting those that consume it. This response serves as a protective mechanism against metabolic stress.
In cancer cells, AMPK activation can lead to tumor suppression. It reduces lipogenesis and boosts fatty acid oxidation, both of which can limit the energy resources available to tumors. Some studies suggest that activating AMPK in cancer cells may enhance their sensitivity to chemotherapy. As researchers continue to evaluate this pathway, AMPK emerges as a potential target for innovative metabolic therapies.
The PI3K/AKT Pathway
The phosphatidylinositol 3-kinase (PI3K)/AKT signaling pathway is crucial in cancer biology. It responds to various stimuli, including growth factors. When activated, it promotes cell survival, growth, and metabolism. The dysregulation of this pathway in cancer leads to unchecked cell growth and resistance to apoptosis.
Inhibitors targeting the PI3K/AKT pathway have been developed and are undergoing clinical evaluation. These inhibitors aim to tackle specific cancer types characterized by aberrant PI3K/AKT signaling. Understanding the intricacies of this pathway is vital for rational drug design and therapeutic interventions.
In summary, exploring the role of signaling pathways in cancer metabolism is essential for advancing treatment modalities. By understanding how these pathways interact, researchers can develop targeted therapies that exploit the unique metabolic dependencies of cancer cells.
Metabolic Reprogramming in Cancer Therapy
Metabolic reprogramming is crucial in the context of cancer therapy. As tumors develop, they undergo significant metabolic changes that distinguish them from normal tissues. Understanding these changes opens up new avenues for therapeutic strategies. The reprogramming of cancer cell metabolism proves useful in targeting the unique metabolic vulnerabilities that malignant cells harbor. This section aims to explore specific elements, benefits, and considerations involved in metabolic reprogramming for cancer treatment.
Targeting Metabolism as a Therapeutic Strategy
Targeting metabolism presents a promising yet challenging strategy in cancer therapy. By focusing on the altered metabolic pathways, researchers can develop agents that specifically disrupt cancer cell viability. For instance, inhibitors of glycolysis, such as 2-deoxyglucose, have the potential to starve cancer cells by blocking their primary energy production route. Unlike normal cells, which can adapt metabolic pathways in response to stress, cancer cells often rely heavily on their reprogrammed metabolism for survival.
The benefits of this strategy lie in its ability to selectively target cancer cells, potentially sparing normal, healthy tissues and reducing side effects associated with standard chemotherapies. Furthermore, metabolic interventions can synergize with existing treatments, enhancing their effectiveness. For example, combining traditional chemotherapies with metabolic inhibitors could induce synthetic lethality, meaning cancer cells with specific metabolic alterations may be driven to apoptosis when both therapies are applied.
However, several considerations arise when targeting metabolism. One major concern is the development of resistance. Cancer cells can adapt rapidly and potentially find alternative routes for energy production. Therefore, ongoing monitoring and adjustment of therapeutic strategies are essential to enhance treatment efficacy and reduce the likelihood of relapse.
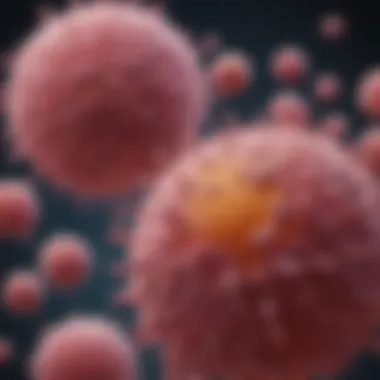
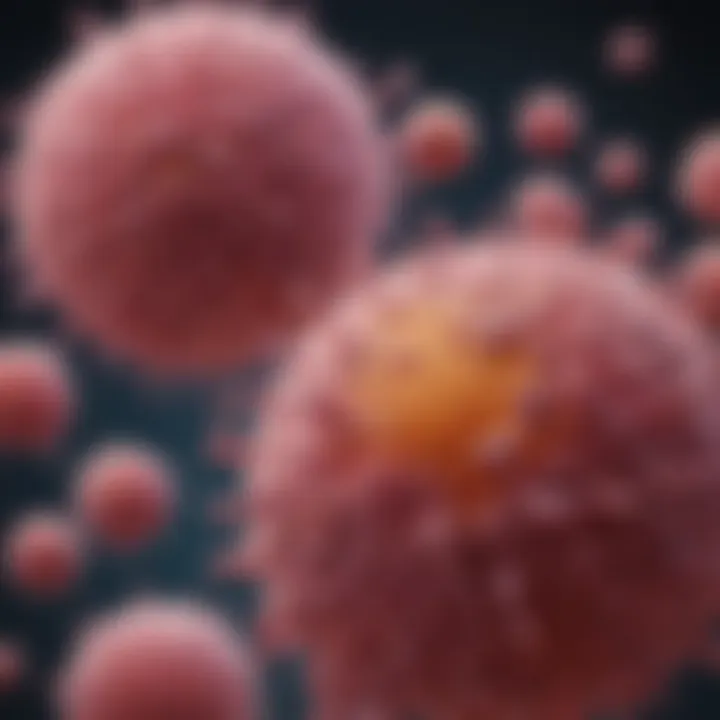
Combination Therapies and Metabolic Modulation
Combination therapies represent an innovative approach to cancer treatment, particularly through metabolic modulation. By simultaneously targeting multiple pathways, these therapies aim to hinder cancer cell adaptation and overcome treatment resistance more effectively.
For instance, combining glycolytic inhibitors with inhibitors of the oxidative phosphorylation pathway can create a dual blockade of energy production. This approach exploits the metabolic flexibility of cancer cells, making it harder for them to survive. Another relevant strategy includes using immunotherapy in conjunction with metabolic interventions. Modifying the tumor microenvironment through metabolic changes may enhance the efficacy of immune checkpoint inhibitors by preventing immune evasion of tumors.
Moreover, metabolic modulation via dietary interventions, such as ketogenic diets, may support combination therapy by inducing further metabolic stress on tumor cells. These innovative approaches require rigorous clinical validation but highlight the exciting potential of metabolic reprogramming in cancer therapy.
In summary, targeting cancer metabolism through reprogramming and combination therapies represents a transformative approach in oncology, opening new horizons for effective cancer treatments.
As this field evolves, ongoing research is vital to identify optimal combinations and refine therapeutic protocols, ultimately advancing the fight against cancer.
Emerging Concepts in Cancer Metabolism Research
Understanding emerging concepts in cancer metabolism is essential for several reasons. As new research continues to reveal how cancer cells manipulate their metabolic pathways, these insights offer critical opportunities for novel therapeutic strategies. The focus should be not only on established metabolic processes but also on how these newer findings can inform and enhance anti-cancer treatments. Two significant areas of interest here are the microbiome's influence on cancer metabolism and the link between metabolic aging and cancer risk.
Microbiome Influence on Cancer Metabolism
The human microbiome plays a significant role in various physiological processes, and its influence on cancer metabolism has started to attract considerable attention. Recent studies suggest that the composition of gut microbiota can impact tumor growth and the metabolic environment of tumors. Certain bacteria can produce metabolites that either promote or inhibit tumor development.
For instance, specific short-chain fatty acids generated by gut bacteria can influence immune responses and inflammation. This can alter how tumors respond to therapies and how effectively they grow. The interaction between gut microbiota and cancer metabolism could lead to new strategies for treatment, perhaps by modulating the microbiome to enhance therapeutic effectiveness.
Moreover, understanding these interactions offers potential for personalized therapy, tailoring treatments based on an individual's microbiota composition. This concept prompts further investigation into how to best manipulate the microbiome for positive outcomes in cancer treatment.
Metabolic Aging and Cancer Risk
Another emerging concept is the connection between metabolic aging and cancer risk. As individuals age, their metabolic processes naturally change, leading to a decline in cellular efficiency. These metabolic changes may create an environment that is more conducive to cancer development. For example, age-related declines in mitochondrial function can lead to increased oxidative stress, which damages DNA and facilitates tumorigenesis.
Research indicates that older adults might have heightened sensitivity to certain metabolic disruptions, potentially increasing their risk of developing cancer. This connection prompts a need to study how aging influences metabolic pathways specifically in cancer cells.
When we consider intervention strategies, approaches that target metabolic aging could offer a dual benefit: improving overall health while simultaneously reducing cancer risk.
"The study of how aging impacts cancer metabolism is crucial for providing insights that may lead to novel preventive measures or therapies."
Overall, these emerging concepts not only expand our understanding of cancer metabolism but also spotlight the need for more integrated research approaches that consider both the systemic environment and intrinsic cellular changes associated with cancer. As the field progresses, these areas will likely yield innovative and transformative strategies in the fight against cancer.
Future Directions in Cancer Metabolism Research
The study of cancer cell metabolism is dynamic and continually evolving. Future directions in research are vital as they promise to unravel complexities that could lead to new therapeutic approaches. Understanding how cancer cells adapt their metabolism can inform innovative strategies that improve patient outcomes. Researchers are focusing on identifying novel metabolic pathways that may be exploited in the development of new drugs. Moreover, personalized metabolic therapies are becoming more significant, acknowledging that not all patients respond uniformly to treatment due to differences in metabolism.
Investigating Novel Metabolic Pathways
One promising area in cancer metabolism research is the identification of novel metabolic pathways that are active in cancer cells but not in normal cells. For example, recent studies have highlighted the role of the serine-glycine one-carbon cycle in certain cancers. This pathway is crucial for nucleotide and amino acid synthesis, supporting rapid cell proliferation. Investigations into other less characterized metabolic pathways may uncover targets for therapeutic intervention. Furthermore, understanding how these pathways interact with the tumor microenvironment provides critical insights. Emphasis is also placed on the metabolic network's plasticity, as the tumor may adapt to changing conditions or therapies.
"By targeting specific metabolic alterations in cancer cells, researchers hope to develop more effective and less toxic treatment modalities."
Personalized Metabolic Therapies
Personalized medicine is revolutionizing how cancer treatment is approached. In the context of cancer metabolism, this means tailoring therapies based on the individual’s metabolic profile. Researchers are looking at metabolic biomarkers that could predict treatment response. For instance, some cancers rely heavily on glycolysis while others utilize oxidative phosphorylation. Understanding these preferences can guide the selection of targeted therapies. New drug candidates are being designed to specifically inhibit metabolic pathways pivotal for individual tumors. By incorporating patient-specific data, clinicians can optimize treatment regimens, increasing efficacy while minimizing side effects. This holistic view on therapy resonates deeply with the future of cancer care, focusing not just on the tumor but on the patient as a whole.
The End
The exploration of cancer cell metabolism reveals critical insights into tumor biology and therapeutic approaches. Understanding the abstract framework of tumorigenesis is vital for developing effective treatment strategies. This article emphasizes key elements of metabolic shifts in cancer cells, detailing how they divert energy and resources towards growth and survival.
Cancer cells demonstrate a distinct metabolic phenotype, primarily relying on altered ATP production mechanisms such as aerobic glycolysis. This phenomenon, commonly known as the Warburg effect, allows tumors to thrive in conditions where normal cells would struggle. Additionally, the deregulation of biosynthesis pathways plays a significant role in providing cancer cells with necessary building blocks, enhancing their ability to proliferate.
Summary of Key Points
- Cancer cells significantly alter their metabolism compared to normal cells.
- The Warburg effect represents a shift to aerobic glycolysis for energy production.
- Key metabolic pathways such as glycolysis, the TCA cycle, and fatty acid metabolism are pivotal for tumor growth.
- The tumor microenvironment influences cellular metabolism through factors like hypoxia and nutrient competition.
- Understanding signaling pathways like mTOR and AMPK can lead to new therapeutic strategies.
The Importance of Continued Research
Ongoing research in cancer metabolism is essential for several reasons. Novel insights into how metabolic pathways are exploited by cancer can facilitate the identification of new drug targets. Advances in personalized medicine hinge on our ability to tailor therapies based on individual metabolic profiles, enhancing treatment efficacy and reducing side effects.
Moreover, the role of the tumor microenvironment and its metabolic interactions necessitates further investigation. As we deepen our understanding of metabolic reprogramming, we can pave the way for innovative therapies that disrupt the energetic foundations of tumors, potentially leading to better patient outcomes.