Comprehensive Analysis of Bacterial Culture Media
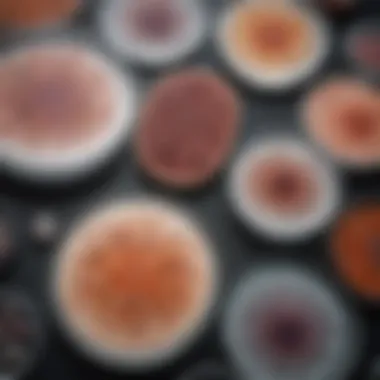
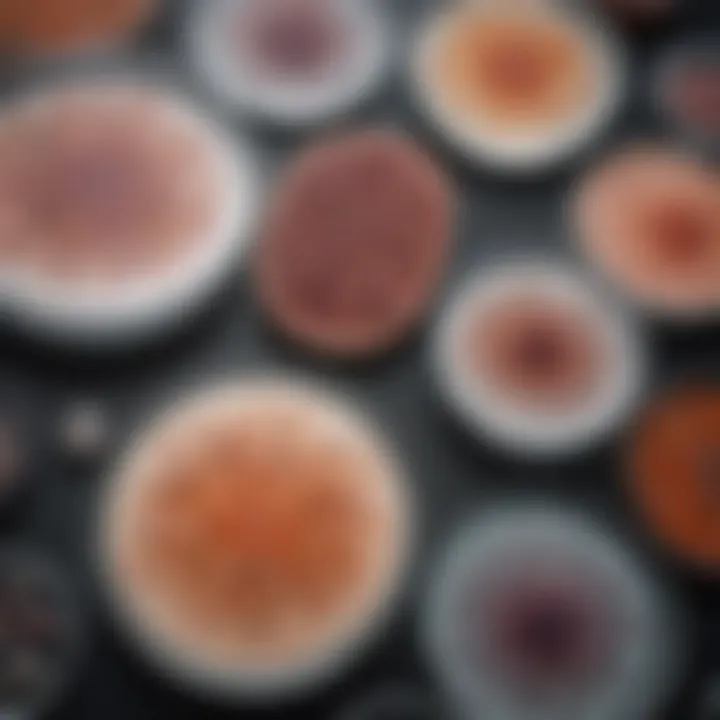
Intro
In the realm of microbiology, the ability to culture bacteria has been a cornerstone of research and experimentation. Understanding the diverse media used for this culturing process is not just about knowing ingredients; it involves appreciating how the composition of media influences bacterial growth, structure, and the production of metabolites. Bacterial media can range from simple nutrient broths to complex agar plates designed for specific strains. This deep dive into the world of bacterial culture media is essential not only for seasoned researchers but also for students navigating the intricate landscape of microbiological study.
The methods described herein will offer a seamless blend of traditional practices and contemporary innovations. By dissecting the components and characteristics of various media, one comes to grasp their specific roles and significance across different scientific fields. Ultimately, this exploration aims to illuminate the meticulous selection process of culture media, which can dramatically sway outcomes in laboratory settings.
The following sections will build a comprehensive narrative around methodologies for culturing bacteria, relevant discussions on findings and implications, and a vision for future explorations in this area. Let's embark on this meticulous examination and unravel the intricacies involved in the craft of bacterial cultivation.
Methodology
Study Design
For this investigation, an analytical approach was taken, taking stock of the vast array of media utilized in bacterial cultivation. The emphasis was placed on categorizing media based on their functions, composition, and the bacterial types they support. Each category serves as a chapter in understanding how media design merges with specific microbial requirements.
The study was structured as follows:
- Classification of media: Nutritional, selective, differential, and enrichment media.
- Examination of selected case studies illustrating successful application of various media in laboratory settings.
- Analysis of recent advances in media formulation, particularly in customized media designs for specific bacterial strains.
Data Collection Techniques
Data for this study was gathered through a combination of review articles, current research publications, and expert consultations in the field of microbiology.
- Literature Review: Extensive searches through databases like PubMed and Google Scholar to compile recent findings and established knowledge.
- Expert Interviews: Conversations with microbiologists to gain insight into practical applications and evolving trends in media use.
- Case Studies: Documentation of specific experiments from laboratory records showcasing the effectiveness of various media types.
Each source provided a corridor into understanding how the selection of media shapes experimental outcomes and the biological behavior of various bacterial species.
"The appropriate choice of culture media can mean the difference between observing growth and witnessing failure in bacterial cultures." - Expert Microbiologist
Discussion
Interpretation of Results
The findings highlight the profound impact that media selection has on bacterial growth behavior and metabolite yield. For instance, the use of selective media like MacConkey agar allows for the cultivation and differentiation of Escherichia coli and Salmonella spp., while supportive media like Luria-Bertani broth provides a rich environment for general use. These examples underscore the maxim that not all media are created equal. Each type serves a definitive purpose, playing a crucial role in defining research trajectories.
Limitations of the Study
While this analysis aimed to be comprehensive, certain limitations must be acknowledged. The focus on specific case studies may inadvertently ignore broader trends applicable across various laboratory environments. Additionally, the evolving nature of microbial research means that continuous updates are necessary for a truly current understanding of culture media applications.
Future Research Directions
Looking ahead, there is substantial scope for further exploration in media formulation. Future studies might aim at:
- Developing media that cater to previously unculturable bacteria to expand research possibilities.
- Investigating the metabolic outputs of bacteria in different media to unravel potential applications in biotechnology.
- Implementing technology like bioreactors that can monitor and adapt media conditions in real-time for precision cultivation.
As we embark on these future inquiries, it’s clear that the science of culturing bacteria will continue to be pivotal in microbiological research, shaping our understanding of these microscopic powerhouses.
Prolusion to Bacterial Culturing
Bacterial culturing is a fundamental technique in microbiology that provides scientists and researchers with the means to isolate, study, and manipulate bacterial strains. It serves as the backbone of various biological studies, playing a vital role in fields ranging from medicine to environmental science. Understanding the principles and methodologies behind bacterial culturing is not merely an academic exercise; it has significant implications for real-world applications, such as antibiotic development, disease treatment, and microbiome research.
The Importance of Bacterial Cultures
Bacterial cultures function as living entities, behaving differently according to their growth media, environmental conditions, and genetic backgrounds. The importance of culturing lies in its ability to:
- Isolate Specific Strains: Culturing allows researchers to target particular bacteria from complex samples, making it easier to study pathogens or beneficial microbes with precision.
- Investigate Growth Patterns: By varying culture conditions, scientists can observe how bacteria respond to different nutrients or stressors, leading to insights about their biology and ecology.
- Develop Diagnostics and Treatments: The ability to culture pathogenic bacteria is crucial for identifying diseases and testing antibiotic efficacy. This practical application underlines the urgency and necessity of mastering culturing techniques.
"Understanding bacterial culture techniques is essential for advancing research and clinical applications in microbiology."
Historical Context of Bacterial Culturing
The practice of culturing bacteria has roots that date back over a century. Louis Pasteur and Robert Koch laid the groundwork for microbiological techniques in the late 1800s. Their work elucidated how bacteria can be isolated and manipulated, pushing the boundaries of scientific knowledge at the time.
The introduction of solid media, like agar, was a turning point in the history of bacterial culturing. It allowed for clearer isolation of individual colonies and helped distinguish between different bacterial species based on their morphology. Over the years, various types of culture media were developed to meet specific research needs:
- Nutrient-Rich Media: Initially, simple nutrient broths were used, but as understanding improved, media were cultivated to support diverse bacterial species.
- Specialized Media: Researchers began designing media tailored for particular types of bacteria, enhancing selectivity and informing best practices for research and clinical applications.
- Innovative Techniques: In the contemporary landscape, advances in technology have led to the use of automation and improved formulations, making culturing processes more efficient and reproducible.
The historical evolution of bacterial culturing not only reflects the growth of microbiology as a scientific discipline but also underscores its vital role in modern biology's myriad applications. The next sections will delve deeper into culture media types, their compositions, and their specific roles in supporting bacterial growth.
Understanding Culture Media
Understanding culture media is a crucial aspect of microbiology that lays the foundation for successful bacterial culturing. The right choice of media can significantly influence the growth, morphology, and metabolism of bacteria, impacting results in research, diagnostics, and industrial applications.
Definition and Purpose of Culture Media
Culture media, by definition, are nutrient solutions designed to support the growth of microorganisms in a controlled environment. Their primary purpose is to provide the essential nutrients, such as carbohydrates, proteins, vitamins, and minerals, that bacteria need to thrive. Additionally, the media can facilitate the isolation and identification of specific bacterial strains by presenting different environments that can favor one group of bacteria over others. In essence, culture media serve as the lifeblood for bacterial growth, aiding in understanding microbial behavior and interactions.
Types of Culture Media
Culture media can be broadly categorized into three main types, each serving a unique purpose in the realm of bacterial culturing.
Liquid Media
Liquid media are solutions that provide a homogeneous environment for bacterial growth. They are particularly beneficial for cultivating bacteria in bulk, making them a popular choice in many laboratory settings. One key characteristic of liquid media is its ability to support rapid growth as nutrients are uniformly distributed throughout the solution. This uniformity allows researchers to easily obtain high yields of bacterial cells for subsequent experimentation. Both tryptic soy broth and Luria-Bertani broth are notable examples of liquid media favored by microbiologists for their effectiveness.
However, while liquid media excel in nurturing growth, they may not provide adequate information on colony morphology and behavior. This limitation sometimes necessitates the use of solid media for more detailed analyses.
Solid Media
Solid media, on the other hand, come with a gelling agent, such as agar, which solidifies the nutrient-rich solution into a workable surface. This consistency allows for the isolation of distinct bacterial colonies, making solid media ideal for discerning characteristics among various bacterial strains. An important feature of solid media is that they allow researchers to conduct colony counting and morphological analysis without the interference that might occur in liquid media.
Some well-known solid media include nutrient agar and MacConkey agar, which offer the added advantage of differential staining, aiding in identification tasks. Yet, one downside to solid media is that they may support slower growth rates compared to their liquid counterparts, sometimes leading to extended culturing periods for certain bacteria.
Semi-solid Media
Semi-solid media represent a hybrid between liquid and solid media, containing a lower concentration of the gelling agent. This unique feature allows them to maintain a certain degree of viscosity while still permitting some diffusion of gases and nutrients. The main advantage of semi-solid media lies in their capability to assess motility in bacteria, as motile bacterial species can travel through the media, providing valuable insights into their behavior.
Examples of semi-solid media include motility agar, which is primarily used for evaluating the motility characteristics of various bacterial strains. One consideration with semi-solid media, however, is that they might not support as vigorous growth as liquid or solid media do due to the restricted movement of nutrients.
Understanding the nuances of each type of culture media is essential for researchers aiming to cultivate bacteria effectively and obtain reliable results. Choosing the right media, whether liquid, solid, or semi-solid, can have lasting impacts on experimental outcomes and downstream applications.
Nutrient Composition of Culture Media
Understanding the nutrient composition of culture media is essential for successful bacterial culturing. The right balance of ingredients not only supports bacterial growth but can also significantly influence the morphology, behavior, and metabolic output of these microorganisms. It’s like providing a meal tailored to a specific dietary need – too much or too little of certain nutrients can throw everything off balance.
The nutrient composition typically leans on two main types of elements: macronutrients and micronutrients. Each category plays unique yet interconnected roles in supporting bacterial health. As we dive into this topic, recognizing how these elements work together helps in formulating effective media for a variety of bacterial species, shifting our focus to the precision required in microbial cultivation.
Macronutrients and Their Roles
Macronutrients are the primary building blocks of culture media. They include compounds found in significant amounts and are vital for cellular functions. Some of the most common macronutrients are:
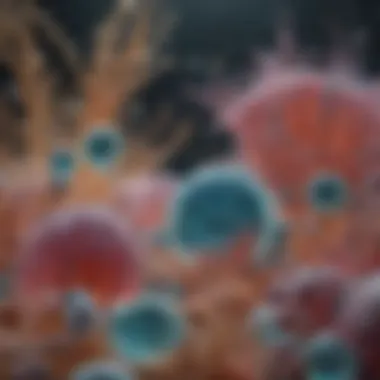
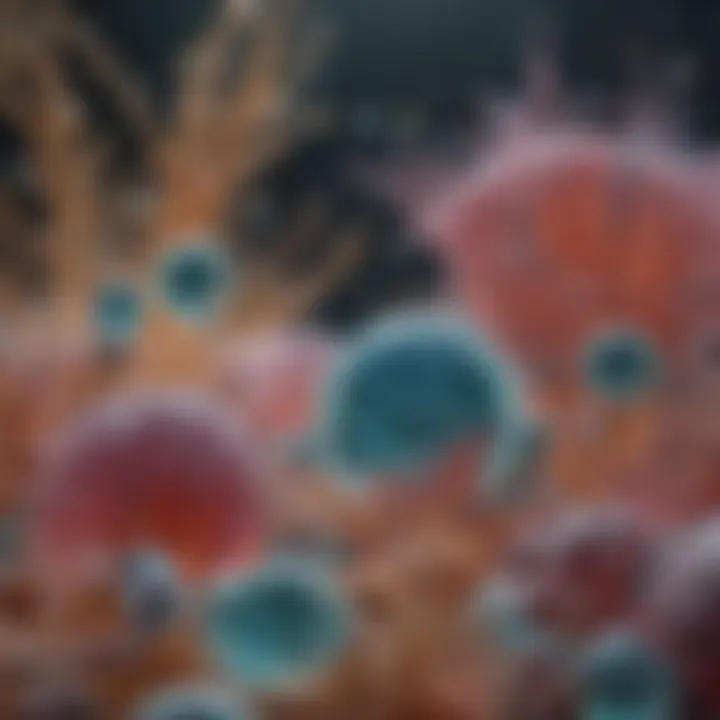
- Carbon Sources: Carbon is the backbone of all organic molecules. Sugars such as glucose and complex carbohydrates serve as energy sources and are crucial for growth.
- Nitrogen Sources: Proteins, amino acids, and ammonia provide nitrogen necessary for the synthesis of nucleotides and amino acids. Without sufficient nitrogen, bacteria struggle to grow and replicate.
- Phosphorus: This nutrient is key for nucleic acids and phospholipids, vital for forming cellular membranes and energy storage in the form of ATP.
- Sulfur: Important for amino acids like cysteine and methionine, sulfur is necessary for protein synthesis as well as enzymatic functions.
The careful selection of these macronutrients enables microbiologists to tailor the culture media to different bacteria’s needs. For instance, certain pathogens might require a high nitrogen load due to their rapid growth rates, whereas other species could thrive on a more minimalistic composition. Balancing these elements is akin to preparing a recipe – too much salt or too little spice can drastically alter the outcome.
Micronutrients in Bacterial Growth
Micronutrients, though needed in smaller concentrations, are no less significant than their macronutrient counterparts. These trace elements often serve as cofactors in enzymatic reactions, supporting various metabolic pathways. Key micronutrients include:
- Iron: Essential for electron transport and enzyme activity, iron often takes the form of iron salts in media formulations. It’s crucial for the survival of many bacteria, especially those involved in pathogenic processes.
- Zinc, Copper, and Manganese: These are typical trace metals that assist in enzymatic functions and structural integrity of proteins. Deficiency can lead to poor growth and dysfunctional cellular processes.
- Vitamins: While not always considered essential for all bacteria, some species require vitamins to conduct vital functions. For instance, certain bacteria rely on B vitamins for energy metabolism.
These micronutrients exemplify the intricate balance necessary in culture media compositions. Inadequate levels can cause stunted growth and even result in unusual metabolic behaviors.
"Understanding the nutrient composition of media is akin to mastering the art of cooking; the right mix of ingredients leads to the desired outcome in bacterial proliferation."
Common Culture Media Used in Microbiology
Understanding common culture media is crucial for anyone diving into the intricate world of microbiology. These media lay the foundational ground for cultivating bacteria, enabling researchers and educators alike to study microbial characteristics, behaviors, and responses under various conditions. Considered the lifeblood of microbiological work, common culture media have specific attributes that determine their effectiveness in supporting different bacterial growth.
When it comes to selecting culture media, numerous factors come into play. The choice often hinges on the desired outcome of the experiment: whether to isolate a specific organism, understand growth patterns, or gauge metabolic functions. Additionally, these media often serve dual purposes—acting not just as a nutritive source but also as a diagnostic tool to differentiate various bacterial species.
Nutrient Agar
Nutrient agar stands as one of the most fundamental and widely utilized media in microbiology. This simple yet effective medium typically comprises peptone, agar, and sodium chloride in distilled water. The primary appeal of nutrient agar is its ability to support a vast range of non-fibrous organisms, making it an excellent general-purpose medium for cultivating bacteria.
- Key Components:
- Peptone: Supplies essential nitrogen, amino acids, and carbon.
- Agar: Acts as a solidifying agent, providing a stable and supportive surface for bacterial colonies to develop.
- Sodium Chloride: Helps maintain osmotic balance.
The versatility of nutrient agar cannot be overstated. It’s often the go-to choice for isolating and identifying various bacterial species from environmental samples or clinical specimens. Moreover, due to its straightforward composition, nutrient agar is also ideal for educational purposes, allowing students to observe growth patterns and colony characteristics without complex media formulations.
Luria-Bertani (LB) Broth
Luria-Bertani broth, often simply referred to as LB broth, plays a significant role in the cultivation of bacteria, especially in molecular genetics and microbiological research. This rich medium is composed of tryptone, yeast extract, and sodium chloride, providing a nutrient-rich environment conducive for bacterial growth.
- Characteristics:
- Tryptone: A source of peptides and amino acids, crucial for bacterial metabolism.
- Yeast Extract: Offers vitamins and additional growth factors that enhance bacterial proliferation.
- Sodium Chloride: Maintains osmotic pressure, ensuring cells remain hydrated and active.
Thanks to its composition, LB broth is particularly favored for the cultivation of Escherichia coli, a staple in laboratory settings. This medium’s robust nutrient profile supports the growth of not only wild-type strains but also genetically modified variants used in various scientific applications.
MacConkey Agar
MacConkey agar serves as a selective and differential culture medium primarily for enteric bacteria. Its formulation includes peptones, sodium chloride, and bile salts, alongside a pH indicator (neutral red) that allows for differentiation based on lactose fermentation.
- Selective Properties:
- Bile Salts: Inhibit the growth of Gram-positive organisms, allowing only Gram-negative bacteria to thrive.
- Lactose: Serves as the fermentable carbohydrate; bacteria fermenting lactose produce acid, leading to color changes in the medium.
One of the primary uses of MacConkey agar is to identify lactose fermenters, such as E. coli, which produce pink colonies, against non-fermenters like Salmonella and Shigella, which remain colorless. This characteristic makes MacConkey agar indispensable in clinical diagnostics and food safety testing, allowing microbiologists to weed out the bad from the good swiftly and effectively.
Using the right culture media is like having the right compass in the world of bacteria; it helps navigate the intricate paths of research and discovery.
Selective and Differential Media
Selective and differential media play a critical role in microbiological practices. These types of culture media are tailored to either favor the growth of specific microorganisms or distinguish between various species based on certain biological characteristics. Understanding these media is paramount for those who venture into bacteria cultures, as they equip researchers and technicians with the tools needed to isolate and identify bacteria effectively.
Definition and Purpose
Selective media are designed to suppress the growth of unwanted bacteria while allowing specific bacteria to grow. This selectivity is often achieved through the inclusion of certain inhibitors, such as antibiotics or bile salts, which act to restrain the growth of non-target organisms.
Differential media, on the other hand, provide visual cues that help differentiate types of bacteria based on their metabolic reactions. For instance, certain media might change color in response to acid production by fermentative bacteria. The capacity to visually categorize growth enables more precise identification of bacteria in a mixed population.
The importance of these media lies not just in identification but also in understanding microbial ecology, disease pathways, and industrial applications. For example, in a clinical environment, selective and differential media can help pinpoint pathogens quickly, which is vital for effective treatment methodologies.
Examples of Selective Media
- Mannitol Salt Agar (MSA): This medium is both selective and differential. It contains a high concentration of salt, inhibiting most bacteria except for Staphylococcus species. If S. aureus is present, it ferments mannitol, causing a color change from red to yellow.
- MacConkey Agar: A well-known selective medium for gram-negative bacteria. The bile salts and crystal violet inhibit the growth of gram-positive bacteria, allowing for the growth of enteric bacteria. Lactose fermenters produce acid, resulting in pink colonies, whereas non-fermenters remain colorless.
- Xylose Lysine Deoxycholate (XLD) Agar: This medium is selective for enteric gram-negative bacteria. It identifies lactose fermenters based on color changes while inhibiting gram-positive organisms due to the presence of deoxycholate.
Differential Media Characteristics
Differential media are instrumental for microbiologists because they expose metabolic variations among bacteria. Some key characteristics include:
- Color Changes: Bacteria may produce specific enzymes that alter the appearance of the medium. For example, phenol red is often incorporated as a pH indicator, changing color in response to fermentation.
- Aerobic vs. Anaerobic Growth: Some media can distinguish bacteria based on their oxygen requirements. For instance, certain differential media support aerobic growth, while others facilitate anaerobes.
- Biochemical Reactions: A differential medium might include substrates that upon utilization catalyze a visible reaction. An example is the sulfide indole motility (SIM) medium, which can reveal sulfide production and indole presence through black precipitates or color changes.
Ultimately, the use of selective and differential media enriches the understanding of microbial communities, plays a vital role in diagnostic microbiology, and streamlines the identification processes that are crucial in both research and clinical settings.
Enrichment Media for Specific Bacteria
Enrichment media hold a significant place in the art and science of culturing bacteria. They are designed to enhance the growth of particular microorganisms while inhibiting others, effectively tipping the scales in favor of the target bacteria. This process is not merely about fostering life; it is about nurturing the right kind of life amidst a cacophony of microbial competitors. By using enrichment media, scientists can isolate and cultivate bacteria that may otherwise remain hidden or suppressed in mixed samples. This is particularly crucial in clinical diagnostics and microbiological research, where identifying specific pathogens can make a world of difference.
Concept of Enrichment Cultures
The concept behind enrichment cultures is straightforward yet powerful. It revolves around creating a conducive environment that favors the growth of a desired bacterial species. Enrichment media typically contain specific nutrients, growth factors, or concentrations of substances that are tailored to stimulate the growth of the target microbes while stifling those that are non-essential or undesirable. This selective advantage is often achieved through altered pH, temperature, or osmotic pressure, along with the inclusion of inhibitors directed at non-target organisms.
A practical example of this selective culture technique can be seen in the case of Salmonella during food safety inspections. An enrichment broth like Buffered Peptone Water (BPW) allows Salmonella to flourish even in a mixed bacterial population typically present in food samples, enabling its detection despite the complexity of the sample.
This selective growth characteristic of enrichment cultures is integral, especially when dealing with samples that are not pure cultures and might contain a plethora of bacteria. It helps researchers not just to find what they're looking for, but also to understand the dynamics of microbial populations within a specific environment.
Examples of Enrichment Media
Several types of enrichment media have been developed for various target microbes, each with its unique formulation designed to provide optimal growth conditions. Here’s a closer look at a few notable examples:
- Selenite Broth: This medium is particularly effective for the enrichment of Salmonella species. It contains sodium selenite, which inhibits the growth of competing bacteria while promoting the development of Salmonella in fecal specimens.
- Tetrathionate Broth: Another selective medium used primarily for isolating Salmonella, it helps suppress the growth of other enteric bacteria, allowing Salmonella to thrive.
- Manitol Salt Agar (MSA): This medium can be classified as both an enrichment and selective medium, specifically useful for isolating Staphylococcus species, especially Staphylococcus aureus. The high salt concentration inhibits most other microbes, while mannitol serves as a fermentable carbohydrate.
- Cetrimide Agar: Formulated for the isolation of Pseudomonas aeruginosa, this medium contains cetrimide to inhibit other organisms, highlighting the selective nature of enrichment cultures.
Enrichment media not only enhance the isolation of specific bacteria but also offer insights into microbial ecology and interactions.
In summary, enrichment media are vital tools in microbiology, enabling the focused investigation of specific bacteria in complex environments. They allow scientists to sift through a microbial haystack to find their needle, ensuring that critical species are not overlooked in the quest for knowledge and discovery.
Hemolytic Media and Their Applications
Hemolytic media play a critical role in microbiological research, particularly in the study of bacteria that can lyse red blood cells. Their applications extend beyond mere cultivation; they help identify and differentiate pathogenic strains from non-pathogenic ones. Understanding the nuances of hemolytic media is essential for researchers and clinical microbiologists as it offers insights into microbial behavior and pathogenicity.
Understanding Hemolysis
Hemolysis, the breakdown of red blood cells, serves as a fundamental process not just in understanding bacterial metabolism but also in diagnosing infections. Certain bacteria, like Streptococcus and Staphylococcus, exhibit this ability, which becomes apparent when cultured on specific types of media. This is essential because the characteristics of hemolysis can signal which bacteria are present in a sample, guiding further testing and treatment.
There are three primary types of hemolysis:
- Alpha hemolysis: Partial lysis that produces a greenish discoloration due to the reduction of hemoglobin to methemoglobin.
- Beta hemolysis: Complete lysis resulting in a clear zone around the colonies, indicating the production of hemolysin enzymes that fully lyse red blood cells.
- Gamma hemolysis: No lysis occurs, meaning bacteria grow without affecting the red blood cells, which can sometimes complicate diagnosis as these strains are non-hemolytic.
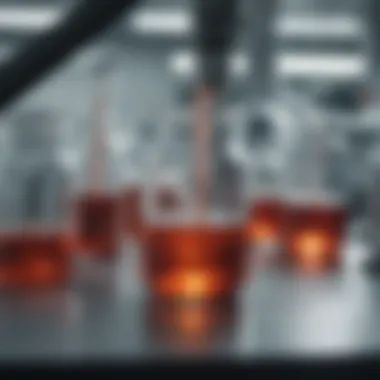
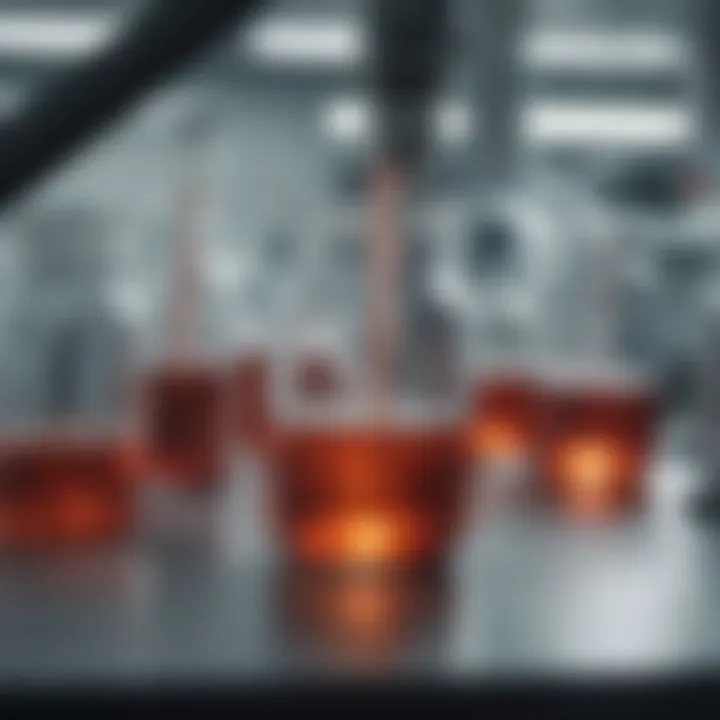
This classification helps in narrowing down the bacterial species and understanding their pathogenic potential.
Types of Hemolytic Media
Various hemolytic media allow for the differentiation of bacterial species based on their hemolytic properties. Here are key media often employed in laboratories:
- Blood Agar: One of the most common hemolytic media, blood agar contains sheep red blood cells and facilitates the growth of a wide range of bacteria while allowing for assessment of their hemolytic properties. The medium supports alpha, beta, and gamma hemolytic reactions, making it versatile for various applications.
- Nutrient Broth with Blood: This variant includes a nutrient broth augmented with blood, offering a liquid medium that supports similar growth characteristics as blood agar while simplifying the observation of hemolytic behavior in liquid cultures.
- Chocolate Agar: Though not a direct indicator of hemolysis, chocolate agar is used to culture fastidious organisms and can provide information about hemolytic capabilities when used alongside blood agar. The red blood cells in this media are lysed, allowing for the detection of nutrients that bacteria may use.
The choice of hemolytic media can dramatically influence the outcome of bacterial culturing and identification.
These types of media allow for detailed examination of bacteria's ability to produce hemolysins. In practice, selecting the right hemolytic medium not only facilitates isolation but also contributes to a deeper understanding of the pathogenic mechanisms involved.
By focusing on hemolysis characteristics, scientists can distinguish not just between types of bacteria but also understand their implications in health and disease, paving the way for targeted research and treatment strategies.
Modern Advances in Culture Media
The landscape of bacterial culturing has transformed dramatically, and modern advances in culture media are at the forefront of this evolution. As researchers strive for more efficient and effective means to cultivate diverse bacterial species, innovative approaches to media composition and preparation have emerged, catering to the complex needs of various microorganisms. These advancements are not just about keeping pace with the demands of contemporary research; they are crucial for enhancing reproducibility, minimizing contamination, and broadening the scope of applicable studies.
Innovative Formulations
In today’s microbiological research, the formulation of culture media has become a dynamic process. Gone are the days when a single, generic medium sufficed for bacterial growth. Today, scientists can tailor media to meet the specific nutritional needs of different bacterial strains, optimizing growth factors to enhance viability and yield.
Some examples of these innovative formulations include:
- Use of specific carbon sources: Some bacteria thrive on particular sugars or organic acids; thus, selecting the right carbon source is vital. For instance, utilizing galactose instead of glucose can favor the growth of certain strains that are sensitive to glucose fermentation.
- Addition of metabolic inducers: incorporating elements like IPTG (isopropyl β-D-1-thiogalactopyranoside) can trigger specific pathways in genetically modified strains, allowing for increased protein expression or metabolite production.
These modifications lead to not only enhanced growth but also enable researchers to investigate specific biochemical pathways, making breakthroughs in synthetic biology and metabolic engineering more attainable.
Automated Media Preparation
As we progress deeper into the age of automation, the preparation of culture media has also seen significant advancements. Automated systems are becoming indispensable in laboratories, offering high-throughput solutions that save time and reduce human error. The design of automated media preparation systems allows researchers to streamline the entire process—right from mixing components to dispensing finished media into suitable containers.
This technology offers several benefits:
- Consistency and Precision: Automated systems ensure that each batch of media is prepared with exact measurements, vastly reducing variability that can affect experimental outcomes.
- Time Efficiency: By minimizing manual labor, scientists can redirect their focus towards more critical aspects of their research, accelerating the pace at which experimental results can be obtained.
- Scalability: Automated processes can easily scale up, accommodating the needs of large-scale studies without compromising quality.
"With the integration of automation in media preparation, researchers can execute experiments with a level of consistency that was previously unachievable."
Selectivity and Specificity of Bacterial Media
In the realm of microbiology, the terms selectivity and specificity are cornerstone concepts when discussing bacterial media. They play a crucial role in crafting an environment that favors the growth of targeted bacteria while suppressing unwanted ones. This selective process is not only essential for isolating pure cultures but also for studying particular strains and understanding their behaviors in different contexts. By optimizing these factors, researchers can achieve more reliable results, paving the way for significant discoveries in both academic and industrial settings.
Factors Influencing Selectivity
Several elements come into play when determining the selectivity of culture media. Here are a few significant ones:
- Nutrient Composition: Different bacteria require specific nutrients for optimal growth. For instance, the addition of certain sugars can favor the growth of lactose fermenters over non-fermenters. Tailoring the nutrient profile can thus create a selective advantage for the desired organisms.
- pH Levels: The acidity or alkalinity of the media can significantly affect bacterial proliferation. Certain bacteria thrive in acidic environments, while others prefer a neutral or alkaline pH. Adjusting the pH can promote the growth of specific bacteria while inhibiting others.
- Antimicrobials and Inhibitory Substances: Incorporating agents that inhibit the growth of certain microorganisms while allowing others to flourish is a common practice. For instance, adding antibiotics like penicillin in selective media can effectively suppress Gram-positive bacteria, thereby targeting Gram-negative organisms.
- Oxygen Tension: The need for anaerobic or aerobic conditions varies among bacterial species. By creating layers of oxygen within the media or by using anaerobic techniques, researchers can selectively culture either aerobic or anaerobic bacteria.
Each of these factors must be carefully balanced to create a media mix that efficiently targets specific bacteria, enhancing the accuracy of microbial investigations.
Optimizing Media for Specific Bacteria
When dealing with specific bacterial strains, optimization of the media is a crucial step to ensure successful outcomes. Here are several approaches focused on optimizing media:
- Tailoring Nutrient Profiles: Understanding the nutritional needs of the target bacteria is fundamental. For example, for Escherichia coli, a modified nutrient agar enriched with yeast extract can stimulate growth significantly.
- Adjusting Physical Properties: Viscosity, solidifying agents, and moisture levels in the media often need tweaking. Some species, like Mycobacterium spp., require a liquid medium that’s more viscous to create a better environment for growth.
- Incorporating Specific Selective Agents: Utilizing compounds that selectively inhibit non-target organisms while enhancing the growth of the specific bacterium can be beneficial. This may include the use of sodium chloride for halophiles or certain bile salts for enteric bacteria.
- Testing and Refinement: Constant experimentation and subsequent adjustment based on observed growth patterns are key. Employing both qualitative and quantitative analyses can shed light on how well the media performs and guide necessary modifications.
“The success of a particular media formulation can make the difference between isolating a rare strain and losing it in the crowd.”
Ultimately, achieving an ideal selectivity and specificity within bacterial media ensures that researchers can conduct focused studies and attain impactful results, making it a vital aspect of microbiological practices.
Applications of Bacterial Culturing in Research
Bacterial culturing has become a pivotal practice in scientific research, serving as the foundation for understanding various biological phenomena. The ability to isolate and cultivate specific strains has profound implications within numerous disciplines, ranging from microbiology to pharmacology. The manipulation of culture media, clearly, plays a crucial part in these endeavors. The understanding of different media types facilitates tailored experiments, which can lead to groundbreaking discoveries.
In the realm of research, the applications of bacterial culturing are as varied as they are significant. Researchers rely on bacterial cultures to elucidate microbial pathogenesis and develop industrial solutions. They gain insights not just into the organisms themselves, but also the environments they thrive in and the roles they play within ecosystems. This comprehensive analysis aims to delve into these specific applications, dissecting their importance and the nuances involved.
Microbial Pathogenesis Studies
The study of microbial pathogenesis is critical in uncovering how certain bacteria can cause diseases in humans, animals, and plants. By culturing bacteria isolated from infected samples, researchers can analyze their characteristics and behaviors under controlled laboratory conditions. These studies enable scientists to dissect intricate interactions between host and pathogen.
For example, examining the bacterial strains responsible for tuberculosis or strep throat requires a reliable and suitable culture medium. Selective media helps isolate pathogens from a mixed sample, while enrichment media can enhance the growth of bacteria that are otherwise difficult to cultivate. Understanding these pathogens not only reveals information about their virulence factors but also assists in developing effective treatments, vaccines, and preventive measures.
Moreover, unlike in the past, where only standard strains were primarily used, researchers now exploit advanced techniques that allow for the study of more diverse bacterial populations. This includes investigating biofilms—communities of bacteria that adhere to surfaces—which have implications in chronic infections and medical device-related complications. Thus, thorough culturing can lead to breakthroughs in clinical microbiology, enhancing patient outcomes across various medical fields.
Industrial Applications
Beyond the realm of health and clinical studies, bacterial culturing fulfills essential roles in various industrial sectors. It is the backbone of processes such as fermentation and bioprocessing, contributing to everything from the production of enzymes to the creation of biofuels.
Take, for instance, the food and beverage industry. Culturing bacteria like Lactobacillus in specific media allows manufacturers to produce yogurt and other fermented dairy products. The choice of culture media influences not only the taste but also the texture and nutritional value of the end product.
In pharmaceuticals, specific bacterial strains are cultivated to produce antibiotics, vitamins, and amino acids. Engineers harness these microbes in bioreactors to optimize conditions for maximal yield. This process includes investigating the precise composition of nutrient media, ensuring the right balance of nutrients for efficient growth and metabolite production.
The benefits of culturing bacteria in industrial applications are vast, providing advantages such as:
- Sustainability: Bacterial processes can often be more environmentally friendly compared to traditional chemical methods.
- Cost-Effectiveness: High yields from cultured processes lower production costs.
- Diversity: A wide variety of products can be generated from microbial metabolism, catering to a plethora of industries.
All in all, the applications of bacterial culturing extend well beyond simple laboratory experiments and into practical, everyday uses in numerous fields.
Understanding how to optimize bacterial culture media significantly impacts both academic research and industrial production.
Quality Control in Culture Media
Quality control in culture media is not just a checkbox to tick off; it's an essential component of any microbiological study or industrial application. The credibility of results hinges on the reliability of the media used to culture bacteria. If the quality of culture media is compromised, the entire experimental setup can falter, leading to skewed results and wasted resources. Thus, maintaining stringent quality assurance processes is critical.
Importance of Quality Assurance
Quality assurance in culture media relates to the systematic processes that ensure the reliability, reproducibility, and validity of results. This encompasses a few key aspects:
- Consistency in Composition: The formulation of culture media can differ, impacting the growth of specific bacteria. Ensuring that each batch maintains a standardized composition is vital.
- Testing for Contaminants: A crucial step in quality assurance involves checking for unwanted microorganisms. Contamination can drastically affect the outcomes of culturing, rendering the results useless. For instance, if a sample meant to detect pathogenic E. coli ends up being contaminated with non-pathogenic strains, it could lead to incorrect diagnoses.
- Performance Validation: Regular validation of media performance against standard organisms ensures that the media supports desired bacterial growth patterns. This is basically a reality check to confirm that the media is doing its job right.
In sum, quality assurance is the backbone of reliable and valid microbiological research. It ensures that the scientific community can trust the results being reported. As someone deep in microbiological research or applications, you really can't afford to overlook this aspect. It’s like trying to bake a cake without measuring the ingredients; it might turn out fine once in a while, but more often than not, you’re left with a mess.
Regulatory Standards and Practices
Navigating the world of quality control requires adherence to various regulatory standards and practices, which set the groundwork for ensuring that culture media are safe and effective for use. Some of the key regulations include:
- ISO Standards: The International Organization for Standardization offers guidelines on testing methods for microbiological media. Compliance with standards like ISO 11133 ensures that media meet the specified requirements for performance and safety.
- EPA Guidelines: In cases where culture media are utilized for environmental studies, it’s imperative to follow the standards set by the Environmental Protection Agency. These guidelines assess the media’s effectiveness for microbial analysis in environmental samples.
- GMP Compliance: Good Manufacturing Practice not only emphasizes quality control measures but also stipulates documentation, traceability, and process validation. This helps ensure that culture media are produced in controlled environments and follow rigorous testing protocols.
Implementing these regulations serves several purposes: it provides a framework for consistency; it enhances the credibility of research findings; and it fosters confidence among stakeholders, including regulatory bodies, which can be pivotal, especially in clinical microbiology, food safety, and pharmaceutical applications.
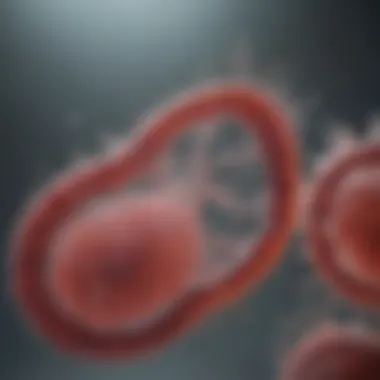
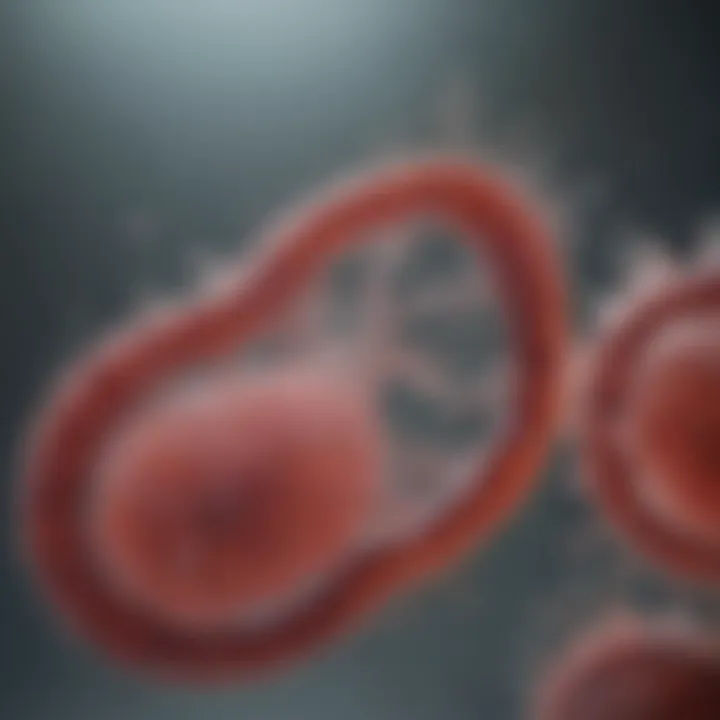
"Quality is never an accident; it is always the result of intelligent effort." - John Ruskin
In summation, the standards and practices surrounding quality control serve as a vital compass, guiding researchers and manufacturers alike to create reliable, effective culture media that contribute meaningfully to the advancement of microbiology.
Challenges in Bacterial Culturing Techniques
Culturing bacteria is not all sunshine and rainbows. There are various hurdles that researchers and microbiologists need to navigate in their quest to grow and study bacteria effectively. Understanding these challenges is crucial as it sheds light on the broader context of microbiological research and its implications in healthcare, industry, and environmental science. Below, we delve into some specific elements that complicate bacterial culturing techniques and discuss considerations that can pave the way for better practices.
Contamination Issues
Contamination is the nemesis of anyone working in a lab related to microbiology. When it comes to bacterial cultures, the presence of unwanted microorganisms can skew results, leading to false conclusions and wasted resources. These contaminants can enter cultures in various ways. It could be from improper handling of samples, insufficient sterilization of equipment, or even airborne particles from the lab environment.
Once a culture gets contaminated, it can be difficult, if not impossible, to discern the original bacteria from the invaders. This complication emphasizes the need for rigorous aseptic techniques during media preparation, handling, and storage. The significance of proper laboratory protocols cannot be overstated:
- Use of sterile techniques when inoculating media.
- Regular environmental monitoring to check for potential contaminant sources.
- Employing filters and laminar flow hoods to create a contamination-free workspace.
Failing to tackle contamination effectively can result in skewed data and unreliable findings, ultimately leading researchers down the wrong path. Therefore, by focusing on enhancing aseptic methods and regularly updating staff training on sterile practices, the reliability of bacterial cultures can be vastly improved.
Media Storage and Stability
Another significant aspect that many might overlook is how the storage and stability of culture media can significantly impact bacterial growth outcomes. Improperly stored media can undergo chemical changes or microbial contamination over time, drastically altering their effectiveness. For instance, if a culture medium is stored in an environment that is too warm or too humid, the physical and chemical properties of the media may degrade.
Considerations regarding media storage include:
- Cool, dark environments: Media stored in places that are temperature-controlled and free from light can significantly extend shelf life.
- Proper sealing and labeling: Each media container should be tightly sealed and clearly labeled with the date of preparation and the intended use.
- Regular checks for signs of degradation: Periodically inspect media for changes in color, consistency, or odor, which can indicate spoilage.
Ensuring that culture media maintain their integrity not only minimizes the risks of contamination but also enhances the reliability of experiments conducted using those media. By addressing these storage issues, researchers can save time, effort, and resources that would otherwise be spent on troubleshooting faulty results.
"Effective bacterial culturing requires vigilance, not just in media preparation, but encompassing every step of the process."
In closing, the challenges associated with bacterial culturing techniques must not be brushed aside. With a strong focus on combating contamination and maintaining media stability, researchers can pave the way for more successful and accurate microbial research.
Transport and Preservation of Culture Media
Transport and preservation of culture media are critical elements in the field of microbiology. The handling of culture media not only impacts their effectiveness but also dictates the reliability of experimental results. Any deviation from optimal storage and transportation conditions can compromise the media, leading to inaccurate data or failed experiments. Even though scientists often focus on the composition of the media itself, the integrity of that media during transport and before use can be just as crucial.
When dealing with culture media, several factors must be considered. First, temperature control is paramount. Many media need to be stored at specific temperatures to maintain their efficacy. For example, some nutrient agar must be kept away from extreme heat or cold to avoid precipitation or degradation of components. This stability in temperature ensures that the nutrients remain bioavailable and the pH levels stay balanced. A fluctuation could lead to changes in osmotic pressure or alter the salt concentration, impacting bacterial growth.
In addition to temperature, the atmosphere in which the media are kept during transport influences their longevity. Exposure to light or certain atmospheric conditions might provoke reactions that can alter the media's components. Hence, using opaque containers or vacuum-sealed packaging can extend the shelf-life significantly.
Key Takeaway: Proper transport and preservation of culture media directly affect the viability and reliability of bacterial growth experiments, ensuring accurate and repeatable results in microbiological research.
Methods for Preserving Media
Several methods can effectively preserve culture media to maintain their robustness during transport and storage. These methods range from simple techniques to more sophisticated approaches that require specialized equipment.
- Refrigeration:
- Freeze-drying (Lyophilization):
- Chemical Preservatives:
- Storage in Controlled Environments:
- Most commonly used method; typically keeping media at 4°C.
- Limits microbial growth and preserves nutrient integrity.
- Prolongs shelf-life significantly by removing moisture.
- Facilitates storage at room temperature.
- Useful for specific formulations that are prone to spoilage.
- Adding agents like sodium azide or formaldehyde can inhibit microbial growth.
- Must be used cautiously to ensure they don’t affect bacterial growth.
- Utilizing desiccators or inert gas environments help in keeping moisture and oxygen at bay.
- Ideal for specialized media that are quite sensitive to elemental presence.
Transporting Culture Media Safely
It is essential to transport culture media with care to avoid potential contamination and degradation. Here are some strategies for ensuring safe transport:
- Use of Secure Containers:
- Temperature-Sensitive Labels:
- Regular Monitoring:
- Packaging:
- Containers should be designed to prevent leaks and contamination. Materials that are resistant to corrosion and impact should be preferred.
- Adding labels that notify of temperature variations helps in tracking the conditions during transport.
- Use of temperature and humidity data loggers during transit allows for continual assessment.
- Utilize padded or insulated packaging to maintain stability during transport.
- Clearly label all packages to delineate contents and storage requirements.
The need for meticulous handling of culture media during its entire lifecycle—from preparation to actual usage—cannot be overstated. Awareness of preservation and transport methods provides a backbone for successful microbial studies, propelling research endeavors forward.
Future Perspectives in Bacterial Culturing
The landscape of bacterial culturing is at a transformative crossroads, with technological advances reshaping old paradigms. Understanding the future of this field is crucial as researchers, educators, and professionals look to innovate while grappling with traditional methods. The implications for science and industry hinge on emerging trends that hold promise for enhancing the precision and efficiency of bacterial culture.
Emerging Trends and Technologies
As the scientific community advances, multiple trends in bacterial culturing catch the eye:
- Microfluidics: This defines a way to manipulate tiny volumes of fluids. In the lab, it allows for quick generation of micro-cultures, enabling scientists to study single bacteria in isolation rather than in bulk. It can uncover unique properties of individual strains, which could be lost when studying masses of cells together.
- Metagenomics: This powerful technique is revolutionizing how we understand microbial communities without needing to isolate them first. By examining genetic material directly from environmental samples, researchers can identify and characterize diverse bacteria that traditional culturing might overlook.
- Organoids: Growing human tissues in gastronomic systems can provide unmatched environments for studying bacteria in a realistic host setting. This direct approach can significantly improve understanding of pathogenic interactions and treatment responses.
These emerging trends not only push the boundaries of microbiology but also promise much-needed advancements in healthcare and environmental science. Understanding these technologies will shape future methodologies and could lead to a significant rewriting of bacterial cultivating practices.
The Role of Artificial Intelligence
Artificial Intelligence (AI) makes a strong entrance into bacterial culturing, carrying the potential to revolutionize the field further. Here’s how AI integrates into this research area:
- Predictive Analysis: By analyzing existing data, AI can forecast the growth patterns of various bacterial strains, helping in media selection and optimizing conditions. Using algorithms to predict what might work best advances knowledge and aids researchers by cutting down trial and error time.
- Image Recognition: AI systems can be trained to recognize bacterial colonies visually, significantly speeding up the analysis process. Such technology also minimizes human error in identifying morphologies and behaviors of microbes.
"The integration of AI in microbiology unlocks opportunities to explore the unknown realms of bacterial behavior and growth more effectively than ever before."
- Data Management: With an influx of data generated from various experiments, AI offers an effective solution for managing and interpreting large datasets. This adds efficiency and clarity in research, making it easier to glean actionable insights quickly.
The confluence of bacterial culturing and artificial intelligence paves the way for a future marked by precision and clarity in research.
End
The conclusion serves as a vital part of this article, tying together the threads of insight shared throughout the discussion on bacterial culturing media. It crystallizes the significance of choosing the right culture media, emphasizing how it directly impacts bacterial growth and research outcomes. Without a doubt, the selection of media cannot be treated lightly. Each specific type, whether it's nutrient agar or Luria-Bertani broth, offers distinct contributions to the culturing process, affecting everything from morphology to metabolic activity.
Summary of Key Insights
In wrapping up, several key takeaways stand out:
- The diversity of culture media is staggering, each type suited for different bacterial needs.
- Composition plays a critical role. Macronutrients and micronutrients must be balanced to ensure optimal growth.
- Modern advancements, including automated preparation and tailored media formulations, have revolutionized how we approach culturing, increasing efficiency and reducing potential for errors.
- The challenges of contamination and stability in storage highlight ongoing areas for improvement in laboratory practices.
Significantly, the discussion also underscores the relevance of specialized media, such as enrichment or hemolytic media, which can selectively encourage the growth of certain bacterial types over others. These insights are not just academic; they inform real-world applications across research sectors, from pathogenesis studies to industrial microbiology.
Future Directions in Research
Looking ahead, several directions emerge as promising for future research in bacterial culturing. First, emerging trends in genomic and metabolic engineering could lead to even more specialized media formulations, tailored not just to optimize growth but also to enhance desired metabolite production. Also, further integration of artificial intelligence could streamline media design processes, allowing for predictive modeling to rapidly assess media effectiveness before experimental applications.
In addition, the challenge of contamination in culturing will likely spur innovations aimed at developing self-sterilizing media or surfaces that reduce microbial interference. As microbiology continues to branch into more niche fields, fine-tuning media composition will become increasingly crucial.
Maintaining high-quality standards amidst these advancements will remain fundamental. Regulatory practices will need to evolve, ensuring that as we push the boundaries of what’s possible in bacterial culturing, we also uphold safety and reliability in our methods.
Ultimately, the future of bacterial culturing media is ripe with potential, ready to be explored by upcoming generations of scientists and researchers. The depth of knowledge accumulated so far lays a solid foundation, yet the horizon still beckons with unexplored opportunities.